Background
Three-dimensional (3D) strain analysis may help overcome the limitations of Doppler and two-dimensional strain analysis for the left ventricle and become the method of choice for left ventricular (LV) systolic function. The aims of this study were to evaluate the feasibility and reproducibility of LV global 3D systolic strain by real-time 3D speckle-tracking echocardiography (STE) in children and to establish their maturational growth patterns and normal values.
Methods
A prospective study was conducted in 256 consecutive healthy subjects using real-time 3D echocardiography. Full-volume 3D data were acquired using a 3D matrix-array transducer. Three-dimensional LV peak systolic global strain (GS), global longitudinal strain (GLS), global radial strain (GRS), and global circumferential strain (GCS) values were determined using real-time 3D STE.
Results
A total of 228 patients (89%) met the criteria for analysis; 28 (11%) were excluded. The correlations between age and strain variables by real-time 3D STE were poor ( R 2 = 0.01–0.05, P < .05). The differences in GLS and GCS among the five age groups were statistically significant but clinically irrelevant. There were no statistical differences in GRS and GS values among the age groups, nor were there statistical differences between the genders for all 3D strain parameters. Intraobserver and interobserver variability ranged from 5.0 ± 4.3% to 10.1 ± 8.5% versus 6.9 ± 6.1% to 17.0 ± 16.2% for coefficients of variation, respectively. Interclass correlation coefficients ranged from 0.78 to 0.87 and from 0.75 to 0.79 for intraobserver and interobserver measurements for GS, GLS, GCS, and GRS, respectively.
Conclusions
LV global 3D systolic strain analysis using the new 3D STE is feasible and reproducible in the pediatric population. There are small maturational changes in GLS and GCS, but not in GRS and GS, that are statistically significant but probably clinically irrelevant. Further investigation is warranted for potential clinical application of this new technology in a pediatric population.
Conventional indices of regional and global ventricular function, measured by endocardial excursion, such as fractional shortening and ejection fraction, are largely dependent on loading conditions and geometric assumptions. Myocardial velocities determined by Doppler tissue imaging do not rely on geometric assumptions but are inherently unidimensional, angle dependent, variable with aging, and influenced by anthropometrics and heart rate. Myocardial strain has been shown to be more robust for assessment of regional ventricular myocardial function using both echocardiography and cardiac magnetic resonance (CMR) imaging. Recently, two-dimensional (2D) speckle-tracking echocardiography (STE) has been introduced as a new method to quantify myocardial strain. This technique measures myocardial deformation by means of frame-by-frame tracking and motion analysis of speckles within B-mode images. Validation studies with tagged CMR imaging and sonomicrometry in the adult and pediatric populations have provided evidence that 2D STE is a reliable method to determine ventricular myocardial function. However, 2D STE is limited by a number of factors. First, myocardial deformation measured by 2D STE is affected by loss of speckles due to motion outside the imaging plane. Second, 2D STE has limited reproducibility, likely because of variability in the choice of image planes and a lack of standardization in image analysis. Finally, the analysis is cumbersome, and there is a need for a total of six planes for complete analysis, which is a major limitation for automation and potential clinical use.
More recently, the advent of three-dimensional (3D) STE has the potential to overcome the limitations of 2D STE for the assessment of left ventricular (LV) global and regional systolic function. This method tracks the motion of speckles within the scan volume, allowing more complete and accurate assessment of myocardial deformation in all three spatial dimensions by avoiding the loss of speckles because of out-of-plane motion. It has been validated for the quantification of LV volumes and LV wall motion in ischemic heart disease in adults.
Nonetheless, data are scarce and incomplete for the pediatric population with or without congenital heart disease. Thus, the establishment of feasibility, reproducibility, maturational changes, and normal values in this population is a prerequisite for evaluation of these indices for assessing LV function for diagnosis, prognosis, and risk stratification of various congenital and acquired heart diseases in the young before and after medical, percutaneous, and surgical intervention.
The aims of our study were (1) to evaluate the feasibility and reproducibility of LV 3D strain analysis by real-time (RT) 3D STE and (2) to establish the maturational changes and normal values of 3D strain analysis in a pediatric cohort.
Methods
Study Population
A total of 256 consecutive subjects from birth to 18 years of age were prospectively enrolled at Union Hospital in Wuhan, China, between April 1, 2007, and May 1, 2011. The inclusion criteria were (1) referral for echocardiographic evaluation for clinical reasons, (2) no history of congenital or acquired heart disease, and (3) completely normal results on 2D and Doppler echocardiography, with no structural defects and with normal chamber size and systolic function. Exclusion criteria were (1) structural congenital or acquired heart disease, (2) abnormal cardiac rhythms, and (3) hypertension and/or other acute or chronic illnesses. Demographic characteristics, including age and gender, were collected at the time the echocardiographic studies were performed. Informed consent was obtained. The study was approved by the local research ethics committee.
On the basis of somatic growth rate changes in infancy (rapid growth), preschool years (deceleration in growth), preadolescence (relatively steady growth), onset of puberty (beginning of growth spurt), and adolescence (heterogeneous growth in early, middle, and late adolescence), the effects of age and cardiac growth on LV functional indices were examined. Study subjects were divided into five representative age groups : group 1 (birth to 1 year of age), group 2 (1–5 years of age), group 3 (6–9 years of age), group 4 (10–13 years of age), and group 5 (14–18 years of age). Weight, height, and blood pressure measurements were collected for all subjects ( Table 1 ).
Variable | Age (y) | ||||
---|---|---|---|---|---|
Group 1 (<1) | Group 2 (≥1 to <5) | Group 3 (≥5 to <9) | Group 4 (≥9 to <13) | Group 5 (≥13 to <18) | |
n | 23 | 83 | 61 | 36 | 25 |
Male | 13 (56.5%) | 45 (54.2%) | 33 (54.1%) | 17 (47.2%) | 11 (44%) |
Age (y) | 0.56 ± 0.23 | 2.7 ± 1.19 ∗ | 6.88 ± 1.16 ∗ | 10.86 ± 1.23 ∗ | 14.83 ± 1.88 ∗ |
Height (cm) | 65.65 ± 4.45 | 91.74 ± 11.31 ∗ | 122.8 ± 9.38 ∗ | 142.61 ± 9.04 ∗ | 163.35 ± 8.79 ∗ |
Weight (kg) | 7.49 ± 2.04 | 14.16 ± 3.33 ∗ | 24.98 ± 6.26 ∗ | 33.44 ± 7.58 ∗ | 53.02 ± 10.22 ∗ |
BSA (m 2 ) | 0.34 ± 0.05 | 0.59 ± 0.11 ∗ | 0.92 ± 0.13 ∗ | 1.15 ± 0.14 ∗ | 1.52 ± 0.18 ∗ |
SBP (mm Hg) | 81.2 ± 6.9 | 96.5 ± 7.9 ∗ | 101.7 ± 6.5 ∗ | 108.9 ± 8.7 ∗ | 115 ± 9.3 ∗ |
DBP (mm Hg) | 56.4 ± 4.8 | 62.8 ± 6.6 ∗ | 72.3 ± 8.8 ∗ | 86.4 ± 8.8 ∗ | 83.5 ± 8.7 |
HR (beats/min) | 117.13 ± 12 | 101 ± 12.04 ∗ | 91.2 ± 12.95 ∗ | 85.89 ± 13.8 ∗ | 80.75 ± 15.34 |
Echocardiographic Image Acquisition
All subjects underwent complete transthoracic echocardiography to determine cardiac structure, chamber size, and cardiac function according to the recommendations of the American Society of Echocardiography. All studies were performed at rest without sedation. A commercially available system (iE33; Philips Medical Systems, Andover, MA) was used with S5, S8, or S10 broadband phased-array transducers, depending on the age of the subject. Subsequently, all subjects underwent 3D echocardiography using the same system with either an X3-1 or an X7-2 transducer. Images were optimized to obtain the entire left ventricle in a full-volume apical four-chamber view. Data sets were acquired using a four-heartbeat or seven-heartbeat acquisition setting during a period of stable heart rate, defined as the heart rate not changing during the acquisition time and not varying by >2 beats/min during the 30 sec immediately before acquisition. End-expiratory breath holding was performed when feasible. A minimum of four data sets were acquired for each subject, and the three highest quality data sets were selected for offline analysis. Data sets that excluded a portion of the left ventricle, had indistinct endocardial borders, or had stitch artifacts were excluded. Image acquisition was performed by the same investigator (J.G.) within a 30-min time frame.
Echocardiographic Parameters
Cardiac chamber size, mass, and systolic LV function were measured in accordance with the recommendations for chamber quantification of the American Society of Echocardiography. LV end-diastolic length (LVEDL), LV end-systolic length, and mid right ventricular diameter (RVD2) were measured in the apical four-chamber view at the level of the LV papillary muscles. The thickness of the LV septal wall and posterior wall and the LV internal dimension were measured from the parasternal long-axis (LAX) acoustic window. QLAB 3D (Philips Medical Systems) quantification software algorithms were used for semiautomated analysis of LV end-diastolic volume, LV end-systolic volume, LV end-diastolic mass, LV end-systolic mass, and LV ejection fraction (LVEF), as previously described.
3D STE
Offline 3D speckle-tracking echocardiographic analysis was performed using software for echocardiographic quantification (4D LV-Analysis 3.0; TomTec Imaging Systems, Unterschleissheim, Germany). Measurements were made using the data set with the best image quality, which was selected by consensus of the two readers. The frame rate of the volumetric image was 15 to 24 frames/sec. The 3D data sets were displayed as multiplanar reconstruction images corresponding to four tiles containing three standard LAX views (apical four chamber, apical three chamber, and apical two chamber) and a short-axis view, which is orthogonal to the LAX views ( Figure 1 A). The software automatically aligned the four-dimensional data set such that the standard LAX views (apical four chamber, apical three chamber, and apical two chamber) were correctly displayed and the end-diastolic and end-systolic frames were automatically defined. In these LAX views, the LV boundaries were initialized by manually pointing at three anatomic landmarks (mitral annulus and LV apex). The aortic valve landmark was shown within the short-axis view in the middle of the aortic valve at the valve level. Adjustments were made in each multiplanar reconstruction image until the landmarks (mitral valve, LV apex, and aortic valve) were well positioned in each standard view. Then, the 3D endocardial surface was automatically reconstructed and tracked in 3D space throughout the cardiac cycle. The endocardial surface was manually adjusted when necessary until a best match with the actual endocardial position was visually verified in the different views. Subsequently, the Beutel revision state displayed a static 3D surface model of the left ventricle (Beutel) automatically calculated by the application. Finally, the left ventricle was automatically divided into 16 3D segments using the standard segmentation of the American Society of Echocardiography. The curves and maps of the global and the 16-segment 3D LV strain analyses (circumferential, radial, and longitudinal strain) were calculated. Figure 1 illustrates the steps for 3D strain imaging and analysis.

The following parameters of LV strain analysis were evaluated: LV peak systolic global longitudinal strain (GLS), LV peak systolic global radial strain (GRS), LV peak systolic global circumferential strain (GCS), and LV peak systolic global strain (GS), defined as the combination of GLS and GCS. GLS, GRS, and GCS were averaged over the 16 segments. The peak value of each index was defined as its maximum absolute value with a positive or negative sign. Negative strain values reflect shortening, whereas positive strain values represent lengthening or thickening. All strain values are dimensionless and are expressed as percentages.
Reproducibility of 3D Speckle-Tracking Echocardiographic Study Data
Interobserver and intraobserver variability of the measurements was assessed in 50 randomly selected subjects (10 in each age group). To assess intraobserver variability, the same observer (L.Z.) measured the 3D strain analysis twice at an interval of 2 months to avoid recall bias. To assess interobserver variability, 3D strain measurements were performed by a second observer (Y.L.), who was blinded to the results of the first observer (L.Z.).
Statistical Analysis
All demographic, conventional echocardiographic, and 3D strain data are presented as numbers or percentages (mean ± SD). The relations between age and 3D GS parameters were determined using scatterplots, one-way analysis of variance, and second-order polynomial regression analysis. Multiple linear regression analysis was performed to determine the additional effect of anthropometrics (including heart rate, weight, and height) and conventional echocardiographic parameters on the 3D strain variables. The anthropometric and echocardiographic parameters were considered as potential dependent variables, and all variables simultaneously entered in the regression. Intraobserver and interobserver agreement were calculated using the coefficient of variation (i.e., the percentage absolute difference between the measurements divided by their mean value) and intraclass correlation coefficient. P values < .05 were considered statistically significant. Statistical analyses were performed using SPSS for Windows version 16.0 (SPSS, Inc., Chicago, IL).
Results
A total of 256 subjects were evaluated for inclusion in the study. Of those, 28 (10.9%) were subsequently excluded: 10 subjects because of low frame rates as determined by the software system and 18 because of poor 3D images. Thus, the final study group consisted of 228 healthy subjects (mean age, 6.17 ± 4.55 years; 119 boys, 109 girls).
With increasing age, subject characteristics and anthropometric parameters of height, weight, body surface area, systolic blood pressure, and diastolic blood pressure increased significantly ( P < .01), and the heart rate decreased significantly ( Table 1 ).
Conventional echocardiographic parameters of the study subjects are presented in Table 2 . Age differences were noted in LV structural and functional variables ( P < .05), except for LVEF. The mean value of LVEF remained unchanged throughout the age groups ( P > .05).
Variable | Age (y) | P | ||||
---|---|---|---|---|---|---|
Group 1 (<1) | Group 2 (≥1 to <5) | Group 3 (≥5 to <9) | Group 4 (≥9 to <13) | Group 5 (≥13 to <18) | ||
LVEDL (cm) | 3.65 ± 0.42 | 4.23 ± 0.58 | 5.29 ± 0.58 | 5.81 ± 0.67 | 6.84 ± 1.02 | <.0001 |
LVESL (cm) | 2.91 ± 0.43 | 3.16 ± 0.46 | 4.05 ± 0.49 | 4.43 ± 0.58 | 5.26 ± 0.97 | <.0001 |
LVID (cm) | 2.25 ± 0.19 | 2.83 ± 0.36 | 3.41 ± 0.56 | 3.84 ± 0.43 | 4.04 ± 0.49 | <.0001 |
SWT (cm) | 0.41 ± 0.06 | 0.48 ± 0.06 | 0.55 ± 0.09 | 0.67 ± 0.11 | 0.75 ± 0.08 | <.0001 |
PWT (cm) | 0.33 ± 0.04 | 0.36 ± 0.05 | 0.44 ± 0.06 | 0.53 ± 0.08 | 0.56 ± 0.08 | <.0001 |
RVD2 (cm) | 1.63 ± 0.24 | 2 ± 0.29 | 2.4 ± 0.36 | 2.8 ± 0.35 | 2.94 ± 0.39 | <.0001 |
LVEDV (mL) | 14.76 ± 5.59 | 22.49 ± 7.38 | 41.22 ± 11.95 | 53.52 ± 13.94 | 81.78 ± 26.44 | <.0001 |
LVESV (mL) | 5.63 ± 2.48 | 8.08 ± 2.64 | 15.21 ± 4.33 | 19.47 ± 5.9 | 30.34 ± 10.23 | <.0001 |
LVEF (%) | 62.08 ± 4.72 | 63.63 ± 5.47 | 63.15 ± 4.81 | 63.5 ± 5.98 | 62.55 ± 4.86 | .7188 |
End-diastolic mass (g/m 2 ) | 26.31 ± 11.14 | 37.33 ± 12.19 | 62.72 ± 17.97 | 78.95 ± 20.9 | 134.06 ± 41.16 | <.0001 |
End-systolic mass (g/m 2 ) | 14.96 ± 6.07 | 21.68 ± 6.99 | 37.33 ± 9.78 | 48.91 ± 14.89 | 79.25 ± 22.76 | <.0001 |
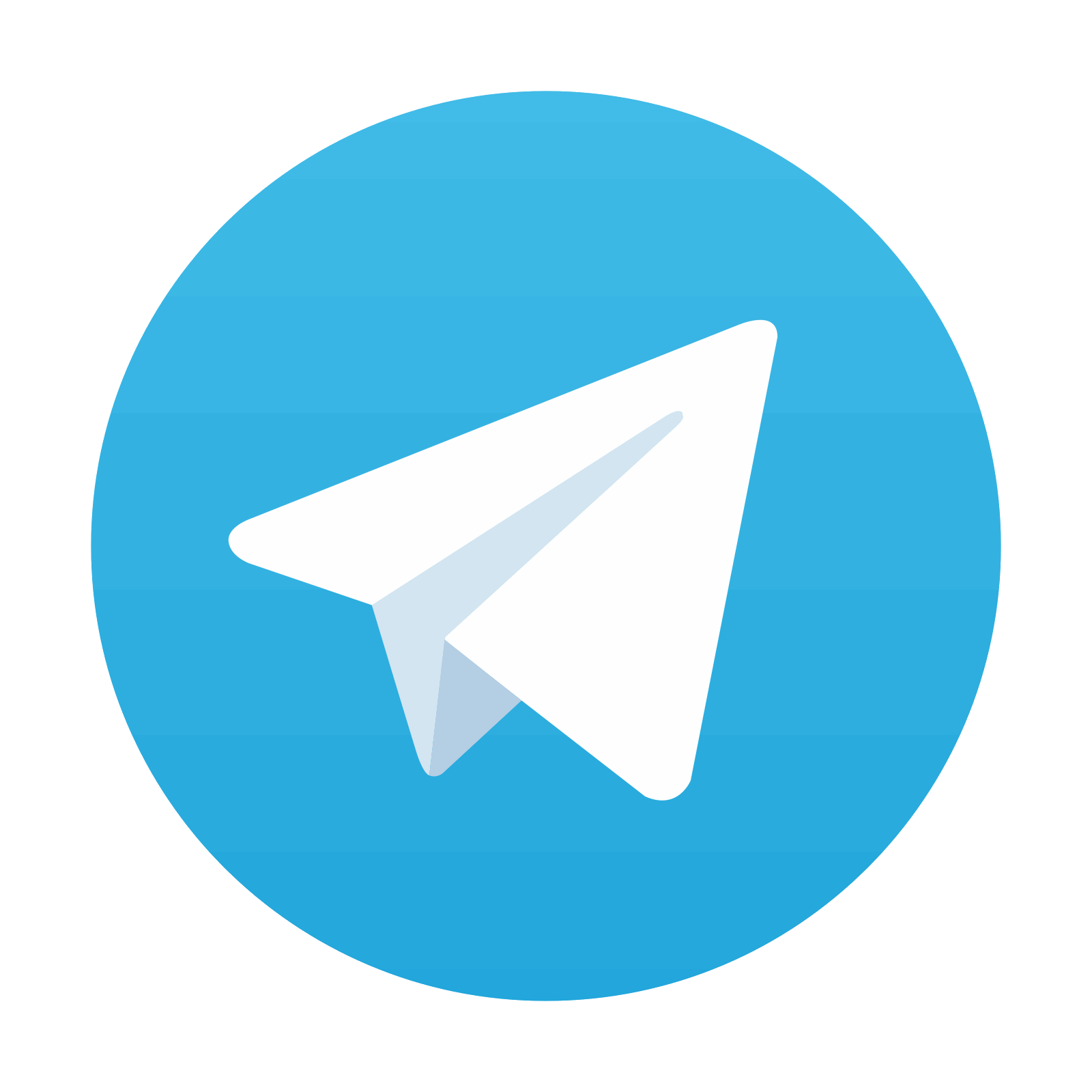
Stay updated, free articles. Join our Telegram channel

Full access? Get Clinical Tree
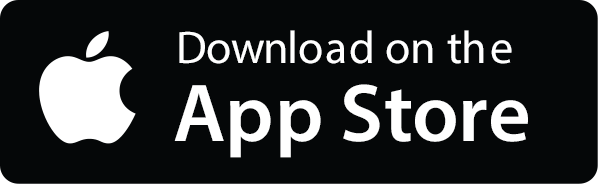
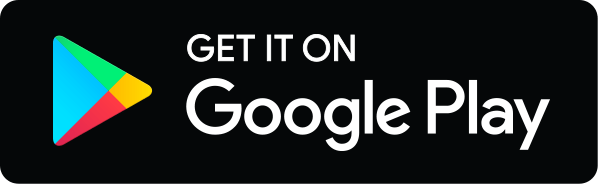
