Background
The impact of mitral valve surgery on left ventricular outflow tract (LVOT) dimensions is unclear. Real-time three-dimensional transesophageal echocardiography permits excellent visualization of the LVOT and might improve standard two-dimensional measurements. In this study, LVOT area and shape were assessed before and after mitral valve surgery.
Methods
Thirty-five patients undergoing mitral valve repair or replacement were retrospectively included in the study and compared with 15 patients undergoing coronary artery bypass grafting. LVOT area was measured by planimetry. Maximum possible methodologic errors by assuming a circular LVOT and an eccentricity index were calculated. LVOT diameter in a midesophageal long-axis view served to calculate the error for the circular LVOT determined in common intraoperative practice.
Results
Common intraoperative two-dimensional measurements underestimated actual LVOT area by 21%. Mitral valve surgery led to a significant reduction of LVOT area by 7%. Although LVOT height remained unchanged, width decreased from 2.72 to 2.53 cm (−7%), resulting in a more circular shape of the LVOT. This effect was more pronounced the smaller the size of the implanted annuloplasty ring or prosthesis. Coronary artery bypass grafting did not affect the LVOT. Left ventricular ejection fraction was significantly correlated with LVOT eccentricity. Impaired ventricular function and higher end-systolic volumes were associated with a rounder shape.
Conclusions
The eccentric LVOT shape leads to a distinct underestimation of its area with two-dimensional measurements. LVOT eccentricity is less distinct in patients with low ejection fractions and higher end-systolic volumes. LVOT width is decreased through annuloplasty rings and prostheses, and the smaller the implanted device, the more profound the reduction.
The morphologic proximity and functional interdependence of the left ventricular (LV) outflow tract (LVOT) and mitral valve (MV) were demonstrated by Lansac et al. This is most evident in the phenomenon of systolic anterior motion, a situation characterized by the dynamic obstruction of the LVOT through the anterior MV leaflet. However, the intraoperative effects of implanted MV annuloplasty rings or MV prostheses on LVOT dimensions have not been previously evaluated. Only one study addressed changes in LVOT through MV surgery, using long-term follow-up magnetic resonance imaging measurements. The results showed a reduction of LVOT diameter variation of 3.6% 2 years after the implantation of rigid annuloplasty rings in a small group of patients ( n = 20).
Recent advances in the clinical measurement of LVOT dimensions have been achieved through the development of three-dimensional (3D) transthoracic echocardiography, magnetic resonance imaging, and multidetector computed tomography, which have all demonstrated the superiority of LVOT planimetry and elliptical calculations over the assumption of a circular shape with single diameter measurements. We therefore considered planimetry as a reference method. All studies evaluating LVOT shape in addition to LVOT size involved populations of either healthy subjects or patients with nondilated or hypertrophic left ventricles. It remains unclear whether the changes caused by MV surgery can be detected reliably with two-dimensional (2D) echocardiography. All preceding studies have been performed under optimized conditions, and whether these methods can be transferred to the fast-paced environment of the operating theater has not been tested.
The intraoperative measurement of LVOT dimensions is of clinical importance for the determination of appropriate aortic valve prosthesis, especially in combined mitral and aortic valve procedures. Furthermore, LVOT cross-sectional area serves to calculate stroke volume and cardiac output to determine the severity of valvular dysfunction in aortic stenosis and mitral regurgitation using the continuity equation.
To differentiate between methodologic errors, which are generated through deviations of the real LVOT from simplified shapes (circle and ellipse), and variations caused by measurement errors or measurements at different cross-sections of the sometimes funnel-shaped LVOT, we repeated 3D measurements to determine intraobserver and interobserver variability.
In the present study, we assessed LVOT properties in MV surgery with 2D and real-time 3D transesophageal echocardiography (TEE), with the following aims: (1) to evaluate whether 3D TEE is feasible in routine intraoperative practice, (2) to compare LVOT cross-sectional area measurements performed with 2D and 3D TEE, (3) to assess LVOT size and eccentricity before and after MV surgery, and (4) to identify other factors influencing LVOT shape.
Methods
Study Population
The study protocol conformed to the ethical guidelines of the 1975 Declaration of Helsinki as reflected by the institution’s human research committee. After approval of the human research committee, consecutive patients with mitral regurgitation scheduled for MV reconstruction or replacement and concomitant monitoring with 3D TEE were enrolled in the study. An interim analysis after data were collected from 30 patients revealed graded effects depending on the size of the implanted devices. Subsequently, five more patients and 15 controls undergoing coronary artery bypass grafting (CABG) were included in the study, therefore making it a retrospective observational study. Exclusion criteria were contraindications to TEE or failure to obtain consent.
Image Acquisition
Intraoperative TEE was performed approximately 30 min after the induction of anesthesia ( t 1 ) and after weaning from cardiopulmonary bypass, as soon as stable hemodynamics were achieved ( t 2 ). A Philips iE33 ultrasound system with an X7-2t matrix-array transducer (Philips Medical Systems, Bothell, WA) was used. The thorough 2D examination of the MV included a midesophageal long-axis view of the LVOT. A full-volume 3D transesophageal echocardiographic recording of the MV and the LVOT served for 3D image analysis. Four physicians certified in echocardiography (T.M., H.R., C.S., T.S.) alternately acquired images.
Two-Dimensional Image Analysis
All stored 2D loops were viewed and analyzed by two independent observers (C.R., M.D.H.), blinded to each other’s findings and to 3D images. All measurements were performed offline and in randomized order. In the midesophageal long-axis view, 2D measurement of the diameter was performed at the narrowest point of the LVOT (approximately 0.5–1 cm upstream of the aortic valve) from trailing to leading edge in mid-systole, at the moment of the greatest aortic valve opening ( Figure 1 ). The cross-sectional area was calculated as A 2D = π r 2 . In patients with arrhythmias, diameter was averaged from three measurements.

Three-Dimensional Image Analysis
Loops were viewed separately by two different observers (C.R., M.D.H.) using a digital review and analysis platform (QLAB version 7.1; Philips Medical Systems). The observers were unaware of the other observer’s results and of 2D information.
We sought to align three standardized planes within the heart. The first longitudinal plane was moved within the 3D data set to cut in a single long axis through the left ventricle, the LVOT, and the center of the aortic valve. The second longitudinal plane was set to again cut longitudinally through the center of the aortic valve and the LVOT, but orthogonal to the first longitudinal plane. A third transverse plane was positioned to be orthogonal to the longitudinal planes, cutting through the narrowest part of the LVOT. The transverse plane was used to measure the longest (LVOT width) and shortest (LVOT height) possible diameters. It was also used for planimetry of the LVOT ( A plan ) ( Figure 2 ). Measurements were performed in early and mid-systole, at the moment of the greatest aortic valve opening. The LVOT area estimate on the basis of an elliptic shape was calculated as A ellip = π × width × height/4. To measure the potential error of assuming a circular shape of the LVOT, the areas A min = π × height/2 2 and A max = π × width/2 2 were calculated.

Analysis of LVOT Shape
An eccentricity index was calculated as 1 − (height/width). An eccentricity index of 0 applies for a perfect circle, with higher eccentricity being represented by increasing numbers, approaching 1 for a line segment.
Aberrations of circular and elliptic shapes from planimetry are demonstrated by providing percentage errors e.g., E = 1 − A min / A plan .
Statistical Analysis
Data are expressed as mean ± SD. A paired two-tailed t test served to compare means at t 1 and t 2 . To compare the differently measured areas ( A 2D , A min , A max and A ellip ) with the reference ( A plan ), Pearson’s correlation coefficient was used.
Single-measurement intraclass correlation coefficients were used to analyze interobserver variability by comparing measurements of the first observer in the first 30 patients with measurements in the same 30 patients performed by a second observer. A single observer repeated measurements with six randomly chosen patients within a period of several weeks. Coefficients of variation of the different parameters were used as a measure for intraobserver variability.
For the subgroup evaluation of different ring and prosthesis sizes and for the comparison of eccentricity in the groups with high and compromised ejection fraction, we used the exact Wilcoxon’s signed-rank tests. Relationships between eccentricity, demographic parameters and LV properties were assessed using Pearson’s correlation coefficient. All tests were two sided. P values < .05 were considered significant.
Results
Patient Population
Thirty-five patients with moderate and severe MV regurgitation scheduled for MV repair were enrolled in the study. MV prolapse and clefts caused regurgitation in 30 patients; the remaining five patients presented with regurgitation due to ischemic or dilated cardiomyopathy. Furthermore, 15 patients with coronary artery disease CAGB were included and served as controls for the evaluation of the impact of the implanted prostheses on LVOT size and shape. In 28 patients, the valve was reconstructed using annuloplasty rings, whereas seven patients received valve replacements. Baseline characteristics are presented in Table 1 .
Variable | Value | |
---|---|---|
Women/men | 11 (22%)/39 (78%) | |
Age (y) | 63.8 ± 10.8 | |
BMI (kg/m 2 ) | 26.5 ± 3.9 | |
Surgery | ||
MV repair | 28 annuloplasty, thereof 19 with triangular resection of PML prolapse | |
MV replacement | 7 | |
CABG | 15 | |
t 1 ( n = 50) | t 2 ( n = 50) | |
LVEDD (mm) | 51.4 ± 10.0 | 46.1 ± 5.3 |
LV ejection fraction (%) | 52.6 ± 16.7 | 48.9 ± 12.5 |
Cross-Sectional Area Through Calculations and Planimetry
The calculated LVOT area on the basis of the assumption of an elliptical shape ( A ellip ) showed the smallest deviation from the planimetric area ( A plan ), which served as reference. There was substantial aberration from the reference, when the smallest and largest diameters of A plan were used to calculate the area of LVOT on the basis of a circular shape. From all single-diameter measurements, the 2D long axis–derived area ( A 2D ) showed acceptable agreement and the least discrepancy with the reference method ( Figure 3 ). All methods correlated significantly with the planimetric area ( Table 2 ).

Variable | Mean (cm/cm 2 ) ± SD P (∆ t 1 / t 2 ) | Method Error (Aberration From A plan ) | Correlation ‡ With A plan | |||
---|---|---|---|---|---|---|
t 1 | t 2 | t 1 | t 2 | t 1 | t 2 | |
A plan | 4.48 ± 0.90 | 4.19 ± 1.09 † | — | — | — | — |
A 2D | 3.59 ± 0.77 | 3.20 ± 0.85 ∗ | ±20% ∗ | ±24% ∗ | 0.690 ∗ | 0.736 ∗ |
A min | 3.26 ± 0.86 | 3.13 ± 0.88 | −27% ∗ | −24% ∗ | 0.872 ∗ | 0.855 ∗ |
A max | 5.98 ± 1.44 | 5.20 ± 1.68 ∗ | +33% ∗ | +24% ∗ | 0.827 ∗ | 0.862 ∗ |
A ellip | 4.38 ± 0.97 | 3.99 ± 1.09 ∗ | ±2% † | ± 5% ∗ | 0.974 ∗ | 0.970 ∗ |
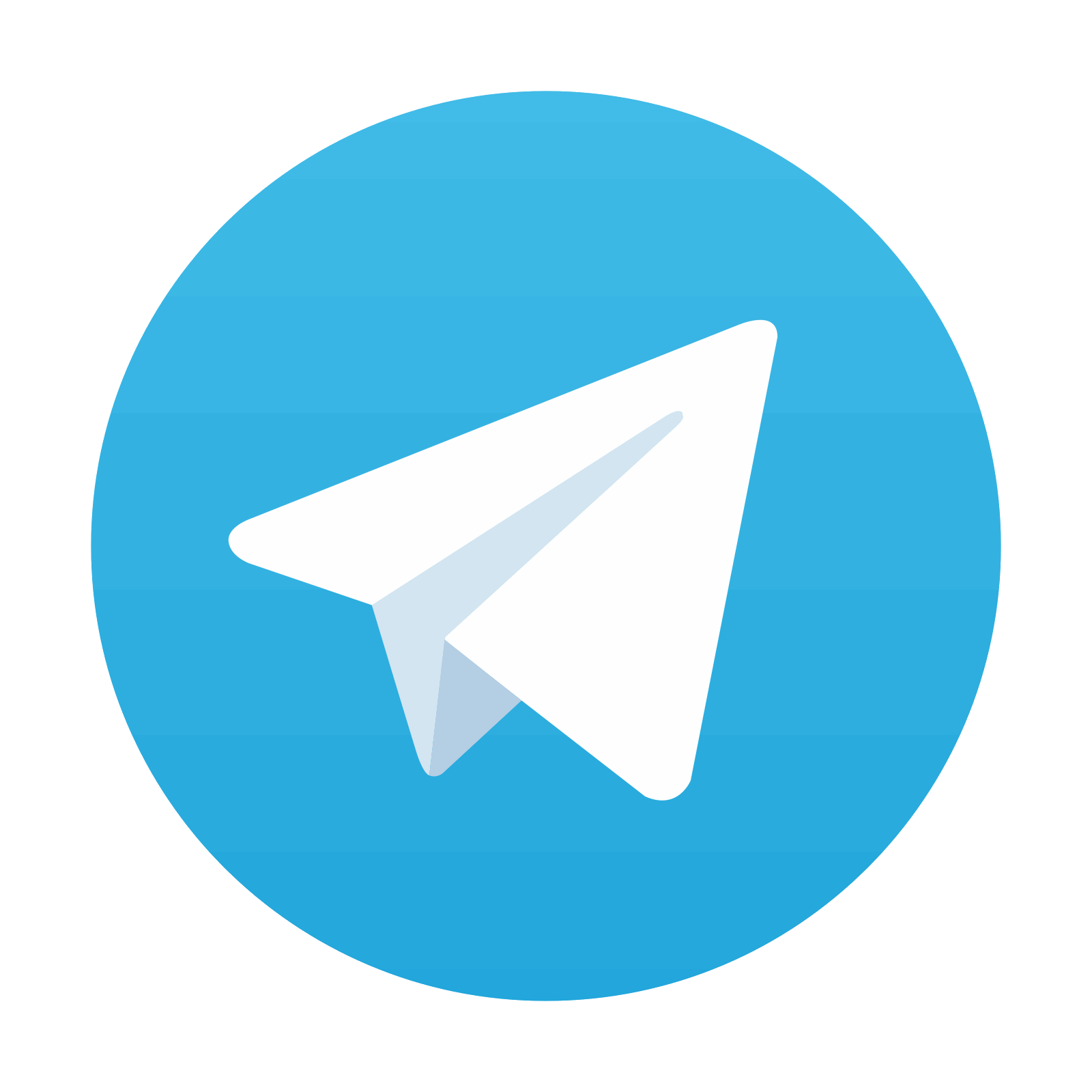
Stay updated, free articles. Join our Telegram channel

Full access? Get Clinical Tree
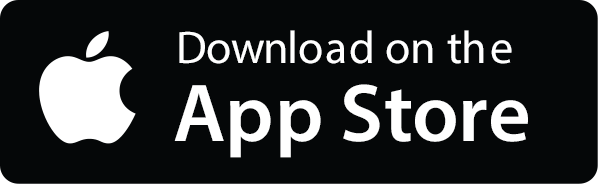
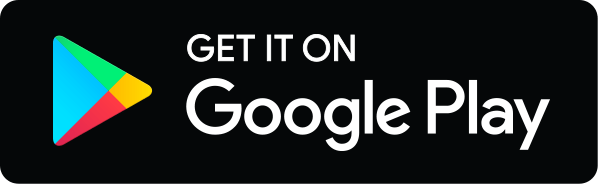