Background
There is still a significant proportion of patients with heart failure who fail to improve after cardiac resynchronization therapy (CRT). The investigators used parametric two-dimensional speckle-tracking echocardiography with polar plots of the amplitude and timing of left ventricular (LV) longitudinal strain to guide implantation of the LV lead, with the aim of increasing CRT response.
Methods
Sixty-four patients who underwent LV lead implantation guided by two-dimensional speckle-tracking echocardiography (study group) and 64 patients treated with standard CRT implantation (control group) were retrospectively analyzed in this study. A positive response to CRT was defined as a reduction of ≥15% in LV end-systolic volume 6 months after implantation. Parametric two-dimensional speckle-tracking echocardiographic evaluation was associated with myocardial end-diastolic wall thickness assessment for recognition of nonviable or irreversibly damaged myocardial tissue.
Results
Compared with the control group, the number of responders increased in the study group (75% vs 48%, P = .002) and in the subgroups of nonischemic (85% vs 59%, P = .022) and ischemic (65% vs 38%, P = .032) patients. The magnitude of echocardiographic LV response also increased in the overall study group and subgroups. In the ischemic patients, the size of transmural scar area correlated with LV reverse remodeling ( r = 0.693, P < .001).
Conclusions
Echocardiography-guided LV lead implantation on the basis of parametric polar plots of LV longitudinal myocardial strain increases both the number of responders and the magnitude of echocardiographic response to CRT. In ischemic patients, size of transmural scar tissue negatively affects CRT response, even when LV lead position is optimized.
Highlights
- •
Stimulating an adequate site of the left ventricle is essential for the best response to cardiac resynchronization therapy.
- •
Left ventricle lead targeting using parametric polar plots of longitudinal myocardial strain by two-dimensional speckle-tracking echocardiography is feasible and effective.
- •
An individualized approach to left ventricle lead placement based on parametric plots of longitudinal strain is suggested for all patients undergoing cardiac resynchronization therapy in routine practice.
Cardiac resynchronization therapy (CRT) is established as a treatment for patients with heart failure (HF) who are refractory to optimized pharmacologic therapy and have reduced left ventricular (LV) ejection fraction (LVEF) and increased QRS duration. However, despite the proven effectiveness of this treatment, there is still a significant proportion of nonresponders. Nonresponse to CRT is multifactorial, and one of the most important negative determinants is stimulation of transmural scar tissue. Therefore, optimizing LV lead implantation site can be a strategy to improve CRT response. The recent Targeted Left Ventricular Lead Placement to Guide Cardiac Resynchronization Therapy (TARGET) and Speckle Tracking Assisted Resynchronization Therapy for Electrode Region (STARTER) studies reported that the effects of CRT may be optimally facilitated when the left ventricle is paced at the most delayed contracting site, avoiding nonviable myocardial tissue. In those studies, baseline two-dimensional (2D) speckle-tracking echocardiographic (STE) imaging based on analysis of radial strain was performed to guide LV lead positioning.
Recently, a different 2D STE approach to the study of nonviable myocardial tissue based on the use of longitudinal strain imaging was validated by our group. This approach relies on a parametric polar plot presentation for display of systolic deformation amplitude data and can be further integrated with evaluation of end-diastolic myocardial wall thickness (EDWT) to recognize those segments unsuitable for pacing stimulation. Also, a 2D STE parametric polar plot presentation can be used to display information about the timing of regional longitudinal myocardial deformation and has been recently applied to guide CRT implantation in association with contrast cardiac magnetic resonance imaging (MRI) for recognition of myocardial scar areas. So far, a combined 2D STE parametric approach (amplitude and timing) associated with EDWT assessment has not been tested to guide LV lead positioning. This approach would have, among others, the important advantage of facilitated visual recognition of the optimal LV pacing site on parametric polar plots, favoring communication with the electrophysiologist and avoiding both measurements on strain curves, whose interpretation may be less intuitive, and the need for contrast cardiac MRI in many patients.
The primary aim of this study was to verify whether optimized LV lead positioning on the basis of the approach described above increases the number of CRT responders. As secondary aims, we sought to verify whether this approach (1) increases the magnitude of the echocardiographic response (reductions in LV volumes and increase in LVEF) and (2) is equally effective in ischemic and nonischemic patients.
Methods
Patient Groups and Protocol
This is an observational retrospective cohort study comparing a study group with a historical control group. A total of 140 patients with advanced HF, eligible for CRT on the basis of cardiac pacing guidelines, were considered for study inclusion. Inclusion criteria were New York Heart Association (NYHA) functional class II to IV, LVEF ≤ 35%, and QRS duration > 120 msec. All patients were symptomatic despite maximally tolerated optimal medical treatment. Ischemic etiology was defined in all patients as the presence of coronary stenoses of >50% by invasive coronary angiography and/or history of myocardial infarction or coronary revascularization.
Study Group Definition
From April 2013 to July 2016, 70 consecutive patients were considered at our institution to receive CRT devices with implantation guided by 2D STE imaging. In two patients, echocardiographic image quality was inadequate, thus CRT implantation was not echocardiographically guided. In two other cases, implantation of the left catheter failed. Two patients were lost to follow-up. Therefore, 64 patients were ultimately included in the study group.
Control Group Definition
From February 2010 to March 2013, 70 consecutive patients were scheduled to receive CRT devices according to standard clinical practice (i.e., without echocardiographic guidance for positioning the LV lead). In one patient CRT implantation failed, and in four echocardiographic image quality was considered inadequate for the assessment of LV volumes. One patient was lost to follow-up. The control group therefore included 64 patients. Before implantation, all patients of both groups underwent detailed clinical assessment and echocardiographic imaging. The same evaluations were scheduled 6 months after device implantation. Patient characteristics are reported in Table 1 .
Variable | Study group patients ( n = 64) | Control group patients ( n = 64) | P |
---|---|---|---|
Age (y) | 68.4 ± 9.0 | 68.4 ± 11.1 | .986 |
Sex (male/female) | 49 (77)/15 (23) | 56 (86)/8 (14) | .239 |
BSA (m 2 ) | 1.92 ± 0.22 | 1.93 ± 0.16 | .680 |
Heart failure etiology (nonischemic/ischemic) | 33/31 (52/48) | 32/32 (50/50) | .860 |
Hypertension | 55 (86) | 47 (74) | .079 |
Diabetes | 14 (22) | 22 (34) | .116 |
Smoking | 20 (31) | 28 (44) | .144 |
One-vessel disease | 7 (11) | 9 (14) | .090 |
Two-vessel disease | 14 (22) | 8 (13) | |
Three-vessel disease | 10 (16) | 15 (23) | |
LBBB | 46 (72) | 44 (69) | .699 |
No LBBB | 18 (28) | 20 (31) | |
QRS duration (msec) | 153.4 ± 23.6 | 155.3 ± 16.1 | .575 |
Sinus rhythm | 53 (83) | 46 (72) | .132 |
Atrial fibrillation | 8 (12) | 14 (22) | |
Pacing | 3 (5) | 4 (6) | |
Class of indication I | 42 (66) | 44 (69) | .100 |
Class of indication IIa | 10 (16) | 14 (22) | |
Class of indication IIb | 12 (18) | 6 (9) | |
NYHA class II, n (%) | 47 (74) | 37 (58) | .060 |
NYHA class III | 15 (23) | 23 (36) | |
NYHA class IV | 2 (3) | 4 (6) | |
β-blockers | 57 (89) | 54 (84) | .435 |
ACE inhibitors/ARBs | 57 (89) | 51 (80) | .144 |
Diuretics | 55 (86) | 58 (90) | .410 |
LV EDV (mL) | 193.0 ± 48.2 | 199.4 ± 55.2 | .482 |
LV ESV (mL) | 138.4 ± 41.8 | 140.5 ± 43.1 | .785 |
LVEF (%) | 29.1 ± 5.9 | 29.8 ± 5.0 | .400 |
Study End Point
Patients were classified as responders if 6 months after pacemaker implantation the LV end-systolic volume (ESV) had decreased by ≥15% with respect to baseline. This volumetric end point was selected because it signifies clinically relevant reverse remodeling. Patients were defined as nonresponders if they did not reach this prespecified echocardiographic change at 6 months or died of cardiac causes. The study was approved by the local ethics committee.
Standard Echocardiography
Standard 2D echocardiography was performed using commercially available ultrasound systems (Vivid 7 and E9; GE Healthcare, Milwaukee, WI) equipped with a 3.5-MHz phased-array transducer. Grayscale and color Doppler data were acquired in a cine-loop format at held end-expiration and digitally stored for offline postprocessing (EchoPAC PC version 112, revision 1.3; GE Healthcare). The three apical views (four-chamber, two-chamber, and long-axis views) were acquired according to standard criteria, and sector size and depth were adjusted to achieve optimal visualization of all LV myocardium with a frame rate between 60 and 100 frames/sec. LV end-diastolic volume (EDV), ESV, stroke volume (SV), and LVEF were calculated using the biplane Simpson method according to current guidelines. EDWT was measured, as previously described, at the eight myocardial basal and midventricular segments of the LV free wall that could potentially be paced ( Figure 1 ). In brief, EDWT was measured at the center of each myocardial segment from the leading endocardial edge to the leading epicardial edge in the parasternal long- and short-axis views. Measurements for each segment were performed in triplicate and averaged. Irreversibly damaged tissue was defined as follows: (1) a reduction in EDWT of ≤5 mm and (2) an abnormal increase in acoustic reflectance characterized by (a) loss of the fine granular reflection of myocardial tissue, which is substituted by highly echo-reflective linear echoes, and (b) lack of distinction between epicardial and endocardial echoes and myocardial tissue. Segments with nonviable or irreversibly damaged tissue were displayed in black color in a white polar plot presentation of the left ventricle for comparison with the same presentation of strain data.

Two-Dimensional STE Imaging
Assessment of LV Longitudinal Myocardial Strain
A region of interest was manually outlined at end-systole by marking the endocardial borders of the left ventricle in each of the three standard apical views. A manual adjustment was performed if the automated tracking was suboptimal. End-systole was defined as aortic valve closure in the apical long-axis view. After tracing, an average time-strain curve was generated by the software for each myocardial segment in each LV apical view, for a total of six curves per view. Time-strain curves were used to calculate global longitudinal strain (GLS), defined as the mean peak systolic longitudinal strain of all segments.
Generation of Strain Amplitude Polar Diagrams
Peak systolic longitudinal myocardial strains calculated throughout the myocardium for each LV apical view were reported spatially, from base to apex and circumferentially, in a polar diagram using a color-coded parametric representation. Because the color coding is triggered from the time-strain curves, these curves were carefully examined by the operator, paying attention to the correct identification of the peak systolic longitudinal myocardial strain on each curve. Manual adjustment was performed if necessary. If curve noise did not allow a precise identification of peak systolic strain on a time-strain curve, that curve was excluded from the subsequent analysis.
Assessment of Myocardial Tissue Unsuitable for Pacing
Peak systolic longitudinal myocardial strain ≤ −5% identifies severely decreased myocardial contractility but can also be an expression of nonviable myocardium in LV segments with transmural healed myocardial infarction. In other words, extremely low contractility and transmural scar cannot be reliably discriminated by very low strain values, so additional information is needed for this purpose. To recognize the transmural scar tissue, we integrated the amplitude strain data from the polar plots with other echocardiographic evaluations. Specifically, EDWT and acoustic reflectance of the LV segments were assessed, on the basis of our previous validation study. On the polar plots, the areas with peak systolic longitudinal strain ≤ −5% are identified by a clear pink color or any shade of blue color ( Figure 1 A). Therefore, the LV myocardial segments unsuitable for pacing were defined as those with clear pink color (or any shade of blue color) on the polar plots and EDWT ≤ 5 mm with an abnormal increase in acoustic reflectance. The areas with longitudinal strain values ≤ −5% on the polar plots were also contoured and expressed as a percentage of the total myocardial area. Areas with longitudinal strain values ≤ −5% and EDWT > 5 mm were excluded from contouring. The reproducibility of parametric strain analysis and EDWT measurement has been previously reported.
Assessment of Timing of Myocardial Contraction
Using the same longitudinal myocardial strain information, the software automatically generates a different color-coded polar map on the basis of time to peak longitudinal strain calculated from the onset of the QRS complex ( Figure 1 B). In this map the green color indicates the earliest time-to-peak values, that is, those preceding or at aortic valve closure; the red color indicates the latest time-to-peak values, that is, those at or after aortic valve closure +250 msec; and colors from yellow to orange represent intermediate time-to-peak values ( Figure 1 B). The latest contracting segment was identified as the one with the most delayed peak strain using the color coded map.
Identification of Optimal Site for LV Pacing
For the study group, parametric 2D STE analysis was made available to the CRT implanter to guide LV lead placement. The target pacing site was defined using both amplitude and timing parametric polar maps in three steps. First, a segmental model of the left ventricle was overlaid on both maps ( Figure 1 C). In this model the eight echocardiographic free wall segments from the basal and mid-LV levels corresponded to the regions of the coronary venous anatomy as follows: anterior = anterolateral, lateral = lateral, posterior = posterolateral, and inferior = posterior. Second, unsuitable (as defined above), septal and apical myocardial areas were excluded from analysis. Third, among the remaining areas, the segment with the latest contraction delay was identified as the optimal site for LV lead position (target site, Figure 2 ). When more than one segment was equally delayed, each segment was considered optimal. Suitable segments adjacent to the optimal site were considered suboptimal. All remaining segments were considered inadequate as pacing sites.

CRT Device Implantation
Implantation Procedure
All patients received biventricular pacemakers with cardioverter-defibrillator function (Boston Scientific, Natick, MA; Medtronic, Minneapolis, MN; Biotronik, Berlin, Germany; or St. Jude Medical, St. Paul, MN). The right atrial and ventricular leads were positioned conventionally. In particular, the right ventricular lead was placed according to operator preference at either the right ventricular septum or right ventricular apex. An extensive coronary sinus venogram documenting all collateral veins was obtained. The use of a balloon catheter for coronary sinus venography was at physician discretion. After venography, insertion of the LV pacing lead was performed. An 8-Fr guiding delivery catheter was used to place the LV lead (Boston Scientific, Medtronic, Biotronik, or St. Jude Medical) in the coronary sinus. In the study group, an attempt was made to place the LV lead at the predefined optimal target site. In the control group, the LV lead was placed in the routine manner, targeting posterolateral or lateral LV regions. In both the study and control groups, due consideration was given to standard pacing parameters such as threshold, sensing, and stability.
Postimplantation Analysis and Programming
In the study group patients, the final position of the LV lead was determined by an independent assessor blinded to the echocardiographic data using a biplane fluoroscopic classification and categorized as basal, mid, or apical in the right anterior oblique projection and as anterolateral, lateral, posterolateral, or posterior in the left anterior oblique view. The final LV lead position was described as optimal if concordant with the optimal target site, suboptimal if adjacent within one segment with stimulation of viable tissue, or inadequate when any other segment was stimulated. A fixed 120-msec atrioventricular delay was programmed according to guidelines. Devices were programmed in DDD mode (apart from patients with atrial fibrillation) to achieve atrial synchronous biventricular pacing.
Statistical Analysis
The sample size calculation was based on the primary hypothesis. It assumed a 50% of CRT responders in the control group. To demonstrate 75% of CRT responders in the study (echocardiography-guided) group, at least 58 patients were needed in both groups, for a total of 116 patients, assuming an α value of 5% and power of 80%. Continuous variables are expressed as mean ± SD and categorical variables as percentages. A paired or unpaired Student’s t test was used to compare continuous values. Percentages were compared using χ 2 analysis. QRS width and the size of transmural scar area were tested as predictors of CRT response. The strength of the association between these two variables at baseline and the variation in ESV at 6 months was evaluated using the Pearson correlation coefficient, r (univariate analysis). Parameters with significant correlations were entered in a bivariate regression analysis (stepwise method), and the capability to discriminate responders and nonresponders was assessed using receiver operating characteristic curve analysis. Interobserver variability for ESV measurement was calculated on the 64 patients of the study group as the SD of the differences between the measurements of the two observers and was 5 mL, or 4% of the mean. Statistical analysis was performed using MedCalc version 11.2.1.0. P values < .05 were considered to indicate statistical significance.
Results
At the 6-month evaluation, two patients had died of cardiac causes in the study group and five in the control group. Therefore, 62 patients in the study group and 59 in the control group were actually assessed at the scheduled clinical and echocardiographic follow-up evaluation. No patient underwent percutaneous coronary intervention or mitral valve surgery or percutaneous procedures during the follow-up period.
Overall Patient Study and Control Groups
Compared with the control group, the number of responders was significantly higher in the study group: 75% (48 of 64) versus 48% (31 of 64; P = .002), thus reaching the primary end point of the study. Both in the study and in the control groups, there were significant decreases in LV ESV and significant increases in SV and LVEF, with greater average variations in the study group ( Table 2 ). LV EDV decreased in the study group, whereas variation was not statistically significant in the control group ( Table 2 ). GLS significantly improved in both groups, as well as QRS width, NYHA class, and mitral regurgitation ( Table 2 ).
Study group: overall patients | Control group: overall patients | ||||||||
---|---|---|---|---|---|---|---|---|---|
Baseline ( n = 64) | 6 months ( n = 62) | Baseline ( n = 64) | 6 months ( n = 59) | ||||||
Mean ± SD or frequency | Mean ± SD or frequency | Average variation or frequency difference | P ∗ | Mean ± SD or frequency | P † | Mean ± SD or frequency | Average variation or frequency difference | P ‡ | |
QRS width (msec) | 153.4 ± 23.6 | 133.0 ± 20.1 | −11.6% | <.001 | 155.3 ± 16.1 | .589 | 143.4 ± 22.0 | −7.9% | .001 |
EDV (mL) | 193.0 ± 48.2 | 170.7 ± 48.7 | −12.2% | .011 | 199.4 ± 55.2 | .507 | 189.4 ± 54.0 | −5.0% | .311 |
ESV (mL) | 138.4 ± 41.8 | 107.5 ± 43.8 | −24.0% | <.001 | 140.5 ± 43.1 | .785 | 124.4 ± 45.4 | −11.4% | .046 |
Global SV (mL) | 54.6 ± 12.7 | 63.2 ± 13.5 | 18.0% | <.001 | 58.8 ± 16.6 | .119 | 64.9 ± 14.8 | 10.4% | .035 |
LVEF (%) | 29.1 ± 5.9 | 39.0 ± 9.9 | 36.1% | <.001 | 29.8 ± 5.0 | .477 | 35.3 ± 6.7 | 17.9% | <.001 |
GLS (%) | −9.0 ± 2.2 | −11.5 ± 3.6 | 27.8% | <.001 | −8.7 ± 1.8 | .413 | −10.3 ± 1.9 | 20.2% | <.001 |
MR grade 1–4 | 23/19/20/2 | 32/18/10/2 | 9/1/−10/0 | .028 | 14/25/13/3 | .079 | 24/23/7/1 | 10/−2/−6/−2 | .019 |
NYHA class I–IV | 0/47/15/2 | 41/20/1/0 | 41/−27/−14/−2 | <.001 | 0/28/22/5 | .057 | 19/16/19/1 | 19/−12/−3/−4 | <.001 |
∗ Six months versus baseline study group.
† Baseline control versus baseline study group.
Analysis of Responder and Nonresponder Patient Subgroups
Responders
Responders were similar in the study and control groups in terms of age (67.4 ± 9.0 vs 66.6 ± 11.5 years, respectively, P = .742), sex (34 and 14 vs 27 and four men and women, respectively, P = .092), etiology (20 and 28 vs 12 and 19 ischemic and nonischemic, respectively, P = .794), baseline QRS width, LV volumes, and LVEF ( Table 3 A). GLS was slightly lower in the control group ( Table 3 A). In both groups LV EDV and ESV were significantly reduced and SV and LVEF significantly increased at 6 months, with average variations greater in the study group ( Table 3 A). GLS significantly improved in both groups as well as QRS width, NYHA class, and mitral regurgitation ( Table 3 A).
A | Mean ± SD or frequency | Mean ± SD or frequency | Average variation or frequency difference | P ∗ | Mean ± SD or frequency | P † | Mean ± SD or frequency | Average variation or frequency difference | P ‡ |
---|---|---|---|---|---|---|---|---|---|
Study group: responder patients | Control group: responder patients | ||||||||
Baseline ( n = 48) | 6 mo ( n = 48) | Baseline ( n = 31) | 6 mo ( n = 31) | ||||||
QRS width (msec) | 155.6 ± 23.4 | 131.2 ± 21.7 | −14.8% | <.001 | 154.2 ± 13.6 | .759 | 129.6 ± 14.1 | −15.9% | <.001 |
EDV (mL) | 189.8 ± 47.8 | 158.5 ± 42.8 | −16.0% | <.001 | 203.4 ± 55.3 | .250 | 176.0 ± 42.1 | −12.7% | <.001 |
ESV (mL) | 134.9 ± 41.1 | 94.5 ± 35.7 | −30.4% | <.001 | 143.7 ± 45.5 | .375 | 109.0 ± 34.1 | −24.0% | <.001 |
Global SV (mL) | 54.9 ± 12.9 | 64.0 ± 13.7 | 20.4% | <.001 | 59.7 ± 14.1 | .126 | 67.0 ± 12.4 | 14.0% | <.001 |
LVEF (%) | 29.7 ± 6.0 | 41.9 ± 8.8 | 44.1% | <.001 | 29.9 ± 4.9 | .853 | 39.0 ± 6.2 | 30.9% | <.001 |
GLS (%) | −9.3 ± 2.2 | −12.4 ± 3.4 | 35.7% | <.001 | −8.3 ± 1.6 | .044 | −10.8 ± 1.5 | 31.4% | <.001 |
MR grade 1–4 | 17/16/13/2 | 27/13/7/1 | 10/−3/−6/−1 | .030 | 9/14/6/2 | .207 | 19/6/5/1 | 10/−8/−1/−1 | .007 |
NYHA class I–IV | 0/34/12/2 | 34/14/0/0 | 34/−20/−12/−2 | <.001 | 0/22/8/1 | .825 | 16/11/4/0 | 16/−11/−4/−1 | <.001 |
B | Study group: nonresponder patients | Control group: nonresponder patients | |||||||
---|---|---|---|---|---|---|---|---|---|
Baseline ( n = 16) | 6 mo ( n = 14) | Baseline ( n = 33) | 6 mo ( n = 28) | ||||||
QRS width (msec) | 146.7 ± 23.6 | 139.4 ± 11.7 | −0.5% | .305 | 156.4 ± 18.3 | .119 | 158.7 ± 18.8 | 0.9% | .638 |
EDV (mL) | 202.6 ± 49.4 | 212.9 ± 45.8 | 0.9% | .585 | 195.6 ± 55.8 | .673 | 204.1 ± 62.1 | 3.5% | .574 |
ESV (mL) | 148.8 ± 43.5 | 151.9 ± 40.6 | −1.9% | .841 | 137.5 ± 41.3 | .383 | 141.6 ± 50.5 | 2.6% | .733 |
Global SV (mL) | 53.8 ± 12.4 | 60.3 ± 13.1 | 9.9% | .172 | 58.1 ± 18.9 | .411 | 62.6 ± 17.0 | 6.4% | .336 |
LVEF (%) | 27.2 ± 5.5 | 29.0 ± 6.3 | 8.7% | .408 | 29.6 ± 5.1 | .140 | 31.2 ± 4.6 | 3.5% | .218 |
GLS (%) | −8.2 ± 2.2 | −8.0 ± 2.0 | −3.0% | .791 | −9.0 ± 1.9 | .153 | −9.5 ± 2.0 | 4.8% | .382 |
MR grade 1–4 | 6/3/7/0 | 5/4/4/1 | −1/1/−3/1 | .165 | 11/7/13/2 | .293 | 11/7/7/3 | 0/0/−6/1 | .206 |
NYHA class I–IV | 0/13/3/0 | 7/6/1/0 | 7/−7/−2/0 | .001 | 0/15/15/3 | .046 | 3/9/15/1 | 3/−4/0/2 | .024 |
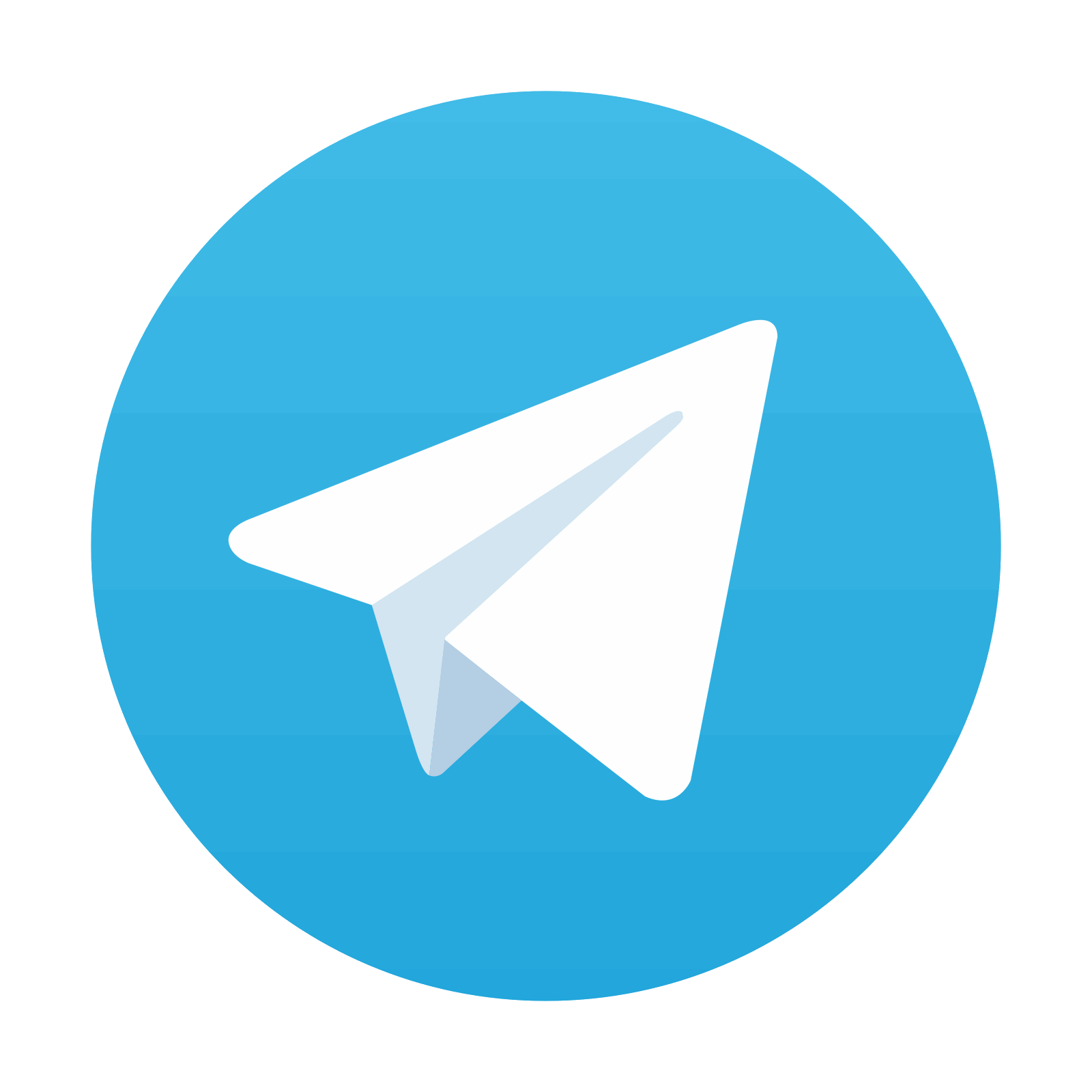
Stay updated, free articles. Join our Telegram channel

Full access? Get Clinical Tree
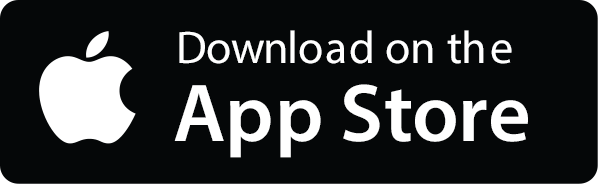
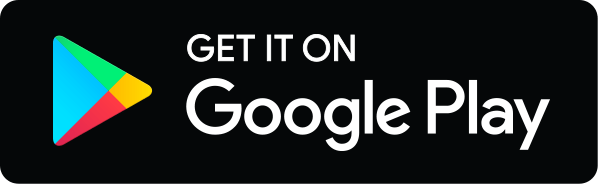
