Abstract
Background
Fractional flow reserve (FFR), the hyperemic ratio of distal (P d ) to proximal (P a ) coronary pressure, is used to identify the need for coronary revascularization. Changes in left ventricular end-diastolic pressure (LVEDP) might affect measurements of FFR.
Methods and Materials
LVEDP was recorded simultaneously with P d and P a during conventional FFR measurement as well as during additional infusion of nitroprusside. The relationship between LVEDP, P a , and FFR was assessed using linear mixed models.
Results
Prospectively collected data for 528 cardiac cycles from 20 coronary arteries in 17 patients were analyzed. Baseline median P a , P d , FFR, and LVEDP were 73 mmHg, 49 mmHg, 0.69, and 18 mmHg, respectively. FFR < 0.80 was present in 14 arteries (70%). With nitroprusside median P a , P d , FFR, and LVEDP were 61 mmHg, 42 mmHg, 0.68, and 12 mmHg, respectively. In a multivariable model for the entire population LVEDP was positively associated with FFR such that FFR increased by 0.008 for every 1-mmHg increase in LVEDP (beta = 0.008; P < 0.001), an association that was greater in obstructed arteries with FFR < 0.80 (beta = 0.01; P < 0.001). P a did not directly affect FFR in the multivariable model, but an interaction between LVEDP and P a determined that LVEDP’s effect on FFR is greater at lower P a .
Conclusions
LVEDP was positively associated with FFR. The association was greater in obstructive disease (FFR < 0.80) and at lower P a . These findings have implications for the use of FFR to guide revascularization in patients with heart failure.
Summary for Annotated Table of Contents
The impact of left ventricular diastolic pressure on measurement of fractional flow reserve (FFR) is not well described. We present a hemodynamic study of the issue, concluding that increasing left ventricular diastolic pressure can increase measurements of FFR, particularly in patients with FFR < 0.80 and lower blood pressure.
1
Introduction
More than 16.3 million Americans have coronary artery disease (CAD), and more than 6.6 million have heart failure . CAD and heart failure may co-exist in patients who present with chest discomfort. Coronary angiography may be helpful in such patients, but the functional significance of angiographically identified coronary stenoses is sometimes uncertain. Invasively measured fractional flow reserve (FFR) is currently the gold standard for assessment of the functional significance of CAD and is used to identify the need for coronary revascularization . Calculation of myocardial FFR by the originally derived equation requires the subtraction of post-arteriolar coronary pressure (P v ) . However, the inclusion of P v may be unnecessary , and recent, landmark clinical trials of the clinical value of FFR have ignored P v .
High left ventricular end-diastolic pressure (LVEDP) may impair myocardial blood flow by increasing coronary zero flow pressure , affecting measurement of FFR. Previous studies have not included substantial numbers of patients with significant left ventricular (LV) dysfunction . Thus, the impact of changes in LVEDP on FFR measurement is largely unknown. We examined the relationship between changes in LVEDP and FFR. We hypothesized that LVEDP would be positively associated with FFR, and that the association would be greater in obstructive disease (FFR < 0.80).
2
Methods
The study protocol was approved by the local Institutional Review Board, and all patients provided informed consent. Procedures were performed in the cardiac catheterization laboratories at the Medical University of South Carolina. The study population consisted of 17 of 37 consenting patients who had at least one vessel with at least intermediate (≥ 40%) disease in whom adequate FFR tracings could be obtained both before and during nitroprusside infusion. FFR measurement was performed according to recommended standards using pressure wires (RadiMedical Systems, Inc., Uppsala, Sweden), which were externally calibrated. Guiding catheters were purged of contrast material and filled with saline, and pressure tracings from the guiding catheter and pressure wire were equalized. Maximal hyperemia was induced by intravenous infusion of adenosine at 140 μg/kg/min. Using a pigtail catheter placed from a second arterial access site into the left ventricle, LVEDP was recorded simultaneously with P d and P a . To reduce LVEDP sodium nitroprusside was then infused intravenously at 1 μg/kg/min during continued adenosine infusion. The nitroprusside infusion was rapidly titrated in increments of 0.5 to 1 μg/kg/min until the LVEDP was reduced to < 10 mmHg or the systolic systemic arterial pressure was reduced to < 80 mmHg. FFR was measured as the ratio of mean P d to mean P a during infusion of adenosine at baseline as well as during infusion of nitroprusside. Attention was paid to avoid pressure damping related to deep engagement of the guiding catheter. Wire pullback was used to assess for signal drift, and repeated measurement was completed as needed.
Simultaneously recorded pressures were reviewed offline by two investigators at scales of 200 mmHg, 100 mmHg, and 40 mmHg to facilitate accurate assessment of both systolic and diastolic pressures, which were assessed on a beat-to-beat basis starting from the R wave on the co-registered electrocardiographic tracing. Premature ventricular beats and post-premature beats were excluded from analysis. Mean P d and P a were calculated for every cardiac cycle by doubling the minimal diastolic pressure, adding the systolic pressure, and dividing by 3. FFR was calculated as P d /P a . FFR < 0.80 was considered to be physiologically obstructive. LV diastolic pressures were measured at end-diastole, which was defined electrocardiographically by the onset of the next cycle’s R wave.
The relationships between LVEDP, P a , and FFR were examined using linear mixed models including a random subject effect to account for correlation among measurements on the same subject. Compound symmetry and variance component covariance structures were considered, and the latter was selected due to better fit. Univariate models as well as a multivariable model including LVEDP, P a , and the interaction between LVEDP and P a as covariates were examined. Sub-group analysis was completed for patients with FFR < 0.80. All analyses were done in SAS version 9.2 (SAS Institute, Cary, NC).
2
Methods
The study protocol was approved by the local Institutional Review Board, and all patients provided informed consent. Procedures were performed in the cardiac catheterization laboratories at the Medical University of South Carolina. The study population consisted of 17 of 37 consenting patients who had at least one vessel with at least intermediate (≥ 40%) disease in whom adequate FFR tracings could be obtained both before and during nitroprusside infusion. FFR measurement was performed according to recommended standards using pressure wires (RadiMedical Systems, Inc., Uppsala, Sweden), which were externally calibrated. Guiding catheters were purged of contrast material and filled with saline, and pressure tracings from the guiding catheter and pressure wire were equalized. Maximal hyperemia was induced by intravenous infusion of adenosine at 140 μg/kg/min. Using a pigtail catheter placed from a second arterial access site into the left ventricle, LVEDP was recorded simultaneously with P d and P a . To reduce LVEDP sodium nitroprusside was then infused intravenously at 1 μg/kg/min during continued adenosine infusion. The nitroprusside infusion was rapidly titrated in increments of 0.5 to 1 μg/kg/min until the LVEDP was reduced to < 10 mmHg or the systolic systemic arterial pressure was reduced to < 80 mmHg. FFR was measured as the ratio of mean P d to mean P a during infusion of adenosine at baseline as well as during infusion of nitroprusside. Attention was paid to avoid pressure damping related to deep engagement of the guiding catheter. Wire pullback was used to assess for signal drift, and repeated measurement was completed as needed.
Simultaneously recorded pressures were reviewed offline by two investigators at scales of 200 mmHg, 100 mmHg, and 40 mmHg to facilitate accurate assessment of both systolic and diastolic pressures, which were assessed on a beat-to-beat basis starting from the R wave on the co-registered electrocardiographic tracing. Premature ventricular beats and post-premature beats were excluded from analysis. Mean P d and P a were calculated for every cardiac cycle by doubling the minimal diastolic pressure, adding the systolic pressure, and dividing by 3. FFR was calculated as P d /P a . FFR < 0.80 was considered to be physiologically obstructive. LV diastolic pressures were measured at end-diastole, which was defined electrocardiographically by the onset of the next cycle’s R wave.
The relationships between LVEDP, P a , and FFR were examined using linear mixed models including a random subject effect to account for correlation among measurements on the same subject. Compound symmetry and variance component covariance structures were considered, and the latter was selected due to better fit. Univariate models as well as a multivariable model including LVEDP, P a , and the interaction between LVEDP and P a as covariates were examined. Sub-group analysis was completed for patients with FFR < 0.80. All analyses were done in SAS version 9.2 (SAS Institute, Cary, NC).
3
Results
Patient characteristics are summarized in Table 1 . A total of 20 coronary arteries were interrogated, and 528 cardiac cycles were analyzed. In 10 of these vessels, the infusion was stopped as LVEDP decreased to ≤ 10 mm Hg while in the remaining 10 vessels, the infusion was stopped for SBP ≤ 80 mmHg. In two patients the infusion was stopped due to hypotension, and these patients were not included in the analysis. During baseline FFR measurement the median (interquartile range) P a , P d , FFR, and LVEDP for all cardiac cycles in all arteries were 73 (63–85) mmHg, 49 (45–57) mmHg, 0.69 (0.60–0.84), and 18 (13–24) mmHg, respectively. FFR averaged for all beats in each artery was < 0.80 in 14 of 20 arteries (70%). During nitroprusside infusion the median P a , P d , FFR, and LVEDP were 61 (58–65) mmHg, 42 (38–49) mmHg, 0.68 (0.64–0.83), and 12 (8–18) mmHg, respectively. Aggregate arterial characteristics at angiography are summarized in Table 2 , while individual arterial hemodynamic characteristics before and during nitroprusside infusion are summarized in Table 3 . Beat-to-beat associations of LVEDP with FFR in representative arteries are shown in Fig. 1 .
Characteristic | |
---|---|
Age (years ± SD) | 65.3 ± 11.5 |
Male (n (%)) | 14 (82.4%) |
ST-elevation Myocardial Infarction (n (%)) | 0 (0%) |
Non-ST-elevation Myocardial Infarction (n (%)) | 2 (11.7%) |
Unstable Angina (n (%)) | 8 (47.1%) |
Stable Angina (n (%)) | 7 (41.2%) |
Left Ventricular Ejection Fraction (% ± SD) | 57.5 ± 14.6 |
Hypertension (n (%)) | 16 (94.1%) |
Diabetes (n (%)) | 6 (35.3%) |
Hemoglobin (g/dL ± SD) | 13.3 ± 1.9 |
Prior Q-wave Myocardial Infarction in Studied Territory (n (%)) | 1 (5.9%) |
Characteristic | |
---|---|
Left Main Coronary Artery (n (%)) | 1 (5%) |
Left Anterior Descending Coronary Artery (n (%)) | 7 (35%) |
Left Circumflex Coronary Artery (n (%)) | 8 (40%) |
Ramus Intermedius Coronary Artery (n (%)) | 1 (5%) |
Right Coronary Artery (n (%)) | 3 (15%) |
Mean Proximal Reference Diameter (mm ± SD) | 2.9 ± 0.5 |
Mean Distal Reference Diameter (mm ± SD) | 2.6 ± 0.3 |
Mean % stenosis ((% ± SD), range) | 71 ± 14, 50-95 |
Mean Lesion Length (mm ± SD) | 20.0 ± 16.2 |
Lesion Type A (n (%)) | 3 (15%) |
Lesion Type B (n (%)) | 9 (45%) |
Lesion Type C (n (%)) | 8 (40%) |
Mild Calcification (n (%)) | 6 (30%) |
Moderate–severe Calcification (n (%)) | 3 (15%) |
Tortuosity (n (%)) | 3 (15%) |
Serial Lesions (n (%)) | 3 (15%) |
Artery | Before Nitroprusside | During Nitroprusside | ||||||||
---|---|---|---|---|---|---|---|---|---|---|
Beats | P a | P d | FFR | LVEDP | Beats | P a | P d | FFR | LVEDP | |
LCx | 14 | 86 | 41 | 0.48 | 21 | 14 | 66 | 26 | 0.39 | 8 |
LCx | 12 | 58 | 46 | 0.79 | 20 | 14 | 48 | 34 | 0.71 | 15 |
LCx | 12 | 76 | 44 | 0.58 | 11 | 13 | 61 | 42 | 0.68 | 10 |
LAD | 12 | 70 | 49 | 0.70 | 9 | 14 | 71 | 54 | 0.76 | 12 |
LIMA-LAD | 12 | 72 | 51 | 0.70 | 24 | 15 | 63 | 43 | 0.68 | 24 |
LCx | 13 | 73 | 48 | 0.66 | 22 | 14 | 60 | 42 | 0.70 | 20 |
RI | 12 | 94 | 94 | 1.00 | 21 | 11 | 99 | 92 | 0.93 | 22 |
LAD | 14 | 59 | 34 | 0.58 | 17 | 14 | 58 | 38 | 0.66 | 22 |
LCx | 14 | 64 | 56 | 0.87 | 8 | 13 | 57 | 48 | 0.83 | 6 |
RCA | 18 | 94 | 58 | 0.62 | 20 | 19 | 75 | 48 | 0.64 | 13 |
LAD | 15 | 71 | 45 | 0.64 | 14 | 19 | 61 | 39 | 0.64 | 10 |
LCx | 13 | 60 | 50 | 0.83 | 16 | 12 | 62 | 54 | 0.87 | 9 |
LAD | 11 | 73 | 56 | 0.76 | 25 | 16 | 60 | 41 | 0.67 | 11 |
LAD | 14 | 77 | 51 | 0.66 | 7 | 16 | 65 | 43 | 0.67 | 6 |
LCx | 14 | 88 | 19 | 0.22 | 24 | 13 | 64 | 13 | 0.21 | 8 |
LAD | 15 | 62 | 59 | 0.96 | 14 | 14 | 43 | 39 | 0.92 | 4 |
LCx | 8 | 66 | 46 | 0.70 | 18 | 7 | 58 | 41 | 0.70 | 16 |
LAD | 11 | 83 | 78 | 0.94 | 22 | 8 | 52 | 49 | 0.95 | 9 |
LCx | 12 | 86 | 45 | 0.52 | 28 | 11 | 60 | 29 | 0.48 | 10 |
LCx | 12 | 60 | 59 | 0.97 | 28 | 13 | 54 | 52 | 0.98 | 26 |
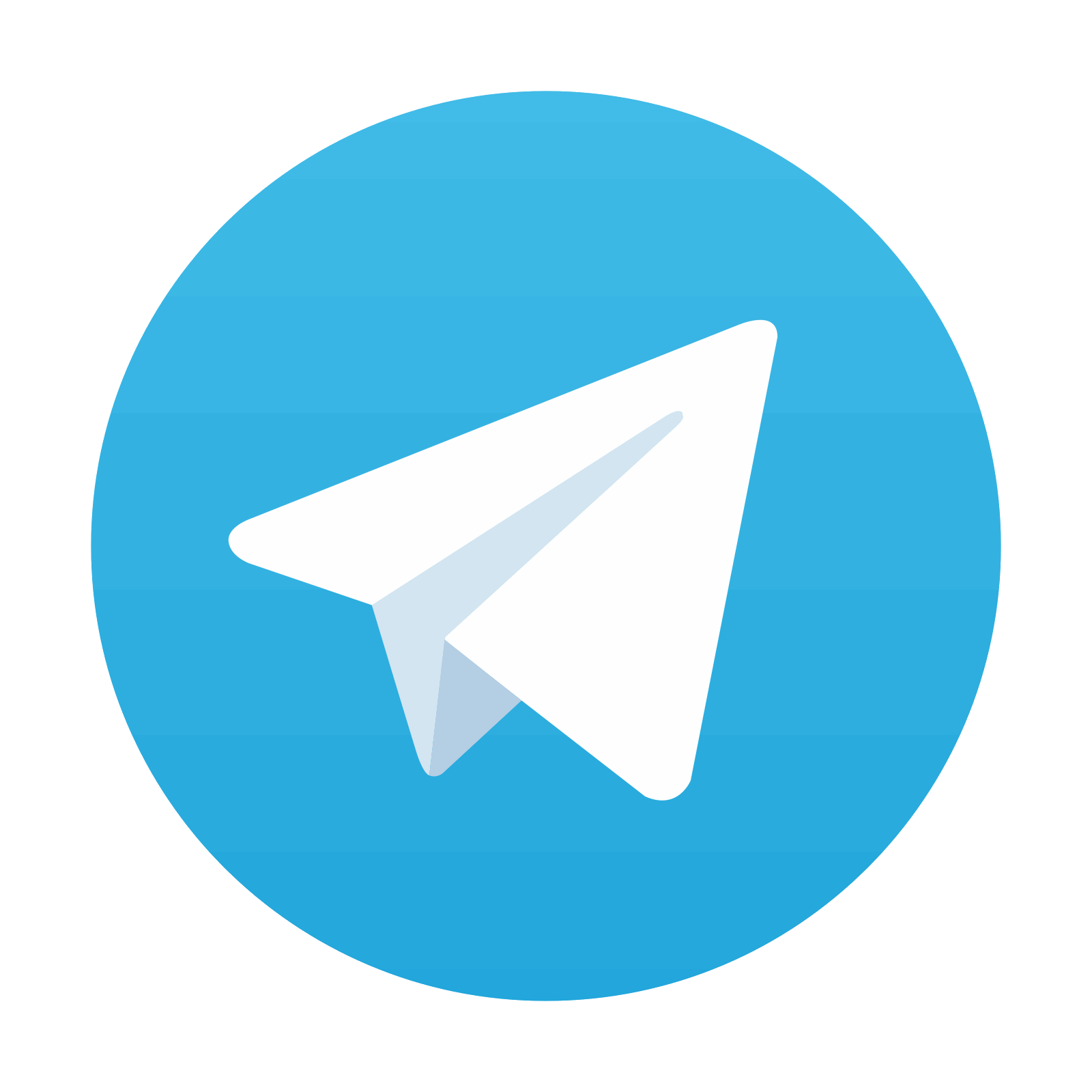
Stay updated, free articles. Join our Telegram channel

Full access? Get Clinical Tree
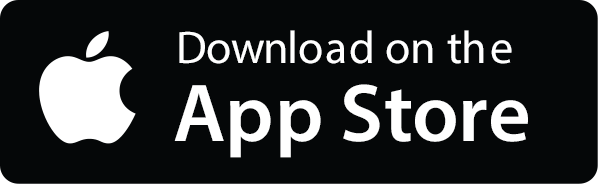
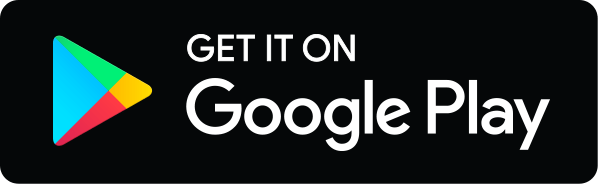
