Summary
Background
Diagnosis of hypertrophic cardiomyopathy (HCM) in athletes can be challenging.
Aims
To ascertain parameters that differentiate patients with HCM from athletes with moderate left ventricular (LV) hypertrophy (LVH 13–15 mm).
Methods
We retrospectively included 100 men: 50 elite rugby players (25 with moderate LVH and 25 with no LVH), 25 patients with HCM and moderate LVH and 25 controls. LV dyssynchrony was defined as the standard deviation of time to peak 2D longitudinal strain (16-segment model) and global strain components were computed from two- (2D) and three-dimensional (3D) speckle tracking.
Results
2D global longitudinal strain (GLS) (18 ± 2% vs. 19 ± 2%) and various 3D strain components were similar in athletes with moderate LVH and controls, while LV volumes and dyssynchrony (39 ± 8 vs. 31 ± 9 ms; P < 0.001) were greater in athletes with moderate LVH. The accuracy for differentiating patients with HCM from athletes ranged between 0.57 and 0.92 for various markers, with the best obtained for LV dyssynchrony (AUC = 0.92; > 48 ms had sensitivity = 83%, specificity = 89%). Binary logistic regression showed that accuracy was improved when LV dyssynchrony was combined with 2D GLS. HCM was excluded when 2D GLS was preserved (> 18%) and there was no LV dyssynchrony (> 48 ms) and only patients with HCM had reduced longitudinal strain and LV dyssynchrony.
Conclusions
LV dyssynchrony combined with GLS can be used to differentiate athletes with moderate LVH from patients with HCM.
Résumé
Contexte
Le diagnostic de cardiomyopathie hypertrophique (CMH) chez les athlètes peut être complexe.
Objectifs
Évaluer les paramètres permettant de différencier les CMH des sportifs ayant une hypertrophie ventriculaire gauche (VG) modérée (HVG entre 13 et 15 mm).
Méthodes
Cents hommes ont été inclus de façon rétrospective : 50 joueurs professionnels de rugby (25 avec une HVG modérée), 25 patients avec une CMH modérée et 25 sujets témoins. L’asynchronisme VG était défini par l’écart-type du délai au pic du strain longitudinal 2D (modèle de 16 segments) et les composants du strain global étaient calculés à partir du speckle tracking 2D et 3D.
Résultats
Le strain longitudinal global (SLG) 2D (18 ± 2 % vs. 19 ± 2 %) et les composants du strain 3D étaient similaires entre les athlètes avec HVG modérée et les contrôles, alors que les volumes et l’asynchronisme VG (39 ± 8 vs. 31 ± 9 ms ; p < 0,001) étaient plus importants chez les athlètes avec HVG modérée. La précision des paramètres 2D et 3D pour différencier les patients CMH des athlètes oscillaient entre 0,57 and 0,92. Le meilleur paramètre échocardiographique était l’asynchronisme VG (AUC = 0,92 ; > 48 ms ; Se = 83 %, Sp = 89 %). Selon le model de régression logistique binaire, l’asynchronisme VG associé au SLG 2D avait la meilleure précision. Les patients CMH étaient exclus lorsque le SLG 2D était préservé (valeur absolue >18 %) et qu’il n’y avait pas d’asynchronisme VG (> 48 ms) et seul les patients CMH avaient un SLG 2D altéré et un asynchronisme VG.
Conclusions
L’asynchronisme VG associé au strain longitudinal global 2D permet de différencier les athlètes avec HVG modérée des patients CMH.
Background
Differentiation between physiological and pathological left ventricular hypertrophy (LVH) is essential, as hypertrophic cardiomyopathy (HCM) is the main cause of sudden cardiac death in young athletes . However, the diagnosis of HCM in athletes can be challenging because 1.5–8% of top-level athletes have moderate LVH (defined by a septal wall thickness of 13–15 mm) and some athletes with early stage HCM can excel in sport. Usually, clinical history, electrocardiogram (ECG) abnormalities and standard two-dimensional (2D) echocardiography allow the identification of patients with HCM . However, cardiac remodelling and ECG features can vary widely depending on the sporting activity and the athlete’s ethnicity. This explains the difficulty in differentiating an athlete’s heart with moderate LVH from early stage HCM with conventional echocardiography and ECG. Recent studies have suggested that tissue Doppler imaging and 2D and three-dimensional (3D) speckle tracking-derived strain might be used to better characterize patients with HCM and the hearts of athletes . Strain analysis provides an accurate and reproducible assessment of longitudinal function and left ventricular (LV) dyssynchrony derived from strain curves may be used to assess the consequence of myocardial disarray and hypertrophy on LV function. The purpose of the study was to examine the accuracy of 2D and 3D strain and LV dyssynchrony for differentiating athletes with moderate LVH (13–15 mm) from patients with HCM.
Methods
Subjects
This case-control study included 100 men: 25 competitive top-level athletes (rugby players) with moderate LVH (13–15 mm), 25 competitive top-level athletes (rugby players) without LVH, 25 patients with HCM and moderate LVH (13–15 mm) and 25 sedentary healthy subjects (control group).
Top-level athletes were recruited from professional players of the Top 14 French National Rugby League. All athletes were screened annually by ECG and echocardiography and none had a history of cardiovascular disease. They had all undertaken endurance (running with interval training) as well as power (bodybuilding) training for > 10 hours/week for at least the past 8 months. The only specific criterion for the inclusion of athletes was septal thickness for athletes with moderate LVH.
HCM was defined by a wall thickness of ≥ 13 mm plus one of the following: a family history of HCM, a positive genetic screening or when a systolic anterior movement of the anterior mitral valve leaflet was present (with or without left ventricular outflow tract [LVOT] gradient). Patients with HCM were selected from our local cohort of patients with proven HCM without LVOT obstruction at rest before alcohol septal ablation or myomectomy. According to the Maron classification, 80% of patients with HCM were type 3, 12% were type 4 and 8% were type 2. Patients with atrial fibrillation, wide QRS duration (≥ 120 ms), an implanted pacemaker, with a suspected cause of HCM other than sarcomeric aetiology or with LV dysfunction (left ventricular ejection fraction [LVEF] ≤ 50%) were excluded.
The control group consisted of sedentary healthy subjects without a history of cardiac disease, with no specific criteria for inclusion. All subjects provided written informed consent and the study was approved by our local ethics committee.
Standard echocardiographic examination
Comprehensive 2D and 3D echocardiography was performed using a commercially available ultrasound system (Vivid 9, General Electrics, Horten, Norway). 2D echocardiography assessment included parasternal long- and short-axis views, apical 4-, 3- and 2-chamber views and conventional and tissue spectral Doppler imaging. LV, left atrial and aortic diameters were measured by M-mode from the parasternal long-axis view. Relative wall thickness (RWT) was defined as the sum of the septal and posterior wall thicknesses divided by the LV end-diastolic diameter. LV mass was determined using Devereux’s formula. Ejection fraction and LV volumes were calculated using Simpson’s biplane method. Pulsed mitral flow and tissue spectral Doppler imaging of the lateral and septal walls were used to assess diastolic function.
3D echocardiography assessment included an LV full-volume acquisition using multi-beat modality (four cardiac cycles) from the apical view. LV volumes and LVEF were computed using semiautomated software (3D auto left ventricular quantification, EchoPAC, GE).
Speckle tracking and dyssynchrony measurements
2D and 3D strain components were computed from apical views using speckle-tracking analysis. The temporal resolutions for 2D and 3D data were 53 ± 3 and 22 ± 7 frames per cardiac cycle, respectively. Analysis was performed offline using dedicated 2D and 3D Echo-PAC software (GE, version 110.1.2). Automated function imaging for 2D imaging and auto left ventricular quantification for 3D imaging used a block-matching model to compute strain data. Endocardial delineation was obtained after manual positioning of the mitral valve plane and LV apex. The region of interest was adjusted manually to provide optimal wall tracking and segments that were inadequately tracked were discarded. For strain processing, the peak of the R wave was used as the end-diastolic reference point. 2D global longitudinal strain (GLS) was obtained by averaging the 16 regional longitudinal strain curves computed from the 2D apical views (4 C, 2 C, 3 C) and 3D GLS and circumferential strain components by averaging the 17 regional strain curves separately. 3D global radial and area strain components were derived by the software from longitudinal and circumferential curves. For better comprehensibility, all strain data are expressed as absolute values. LV dyssynchrony was defined as the standard deviation (16-segment model) (“2D-16SD”) of time to peak 2D longitudinal strain as previously described . Time to peak strain was automatically computed using Excel from exported regional strain curves.
Statistical analysis
Continuous variables with a normal distribution are expressed as mean ± standard deviation (SD), those with a non-normal distribution as median (interquartile range [IQR]). Dichotomous data are expressed as numbers and percentages. To compare numerical data between different groups, paired Student’s t test, analysis of variance (ANOVA), Mann–Whitney and Kruskal–Wallis tests were used as appropriate. Nominal variables were compared using either the χ 2 or Fisher tests. Differentiation between athletes and patients with HCM was performed in the subgroup of subjects with septal or posterior wall thickness 13–15 mm. Receiver operator characteristic (ROC) analysis was performed to evaluate the ability of different echocardiographic parameters to identify patients with HCM and the area under the curve (AUC) expressed accuracy. During echocardiographic analysis in the subgroup of patients with moderate LVH, the investigator (CB) was blinded to the aetiology. Reproducibility of 2D and 3D GLS and LV dyssynchrony was performed in 10 random patients by an independent observer (JT). Reproducibility is expressed as the absolute difference and the absolute difference divided by the mean value (reported as a percentage). Two-tailed P -values < 0.05 were considered statistically significant.
Results
Athletes were younger, taller and heavier than patients with HCM and controls ( Table 1 ). 2D speckle tracking was analysable in all subjects, but 9% of subjects had nonanalysable 3D strain (poor image quality).
Athletes with moderate LVH ( n = 25) | Athletes without LVH ( n = 25) | Patients with HCM ( n = 25) | Controls ( n = 25) | P (athletes with LVH vs. HCM patients) | P (athletes with LVH vs. controls) | |
---|---|---|---|---|---|---|
Age (years) | 27 ± 6 | 26 ± 4 | 55 ± 11 | 33 ± 11 | 0.0001 | 0.001 |
Height (m) | 1.84 ± 0.10 | 1.86 ± 0.10 | 1.70 ± 0.10 | 1.76 ± 0.10 | 0.0001 | 0.0001 |
Weight (kg) | 97 ± 15 | 98 ± 17 | 78 ± 17 | 73 ± 9 | 0.0004 | 0.0001 |
Body surface area (m 2 ) | 2.2 ± 0.2 | 2.2 ± 0.2 | 1.9 ± 0.2 | 1.9 ± 0.1 | 0.0001 | 0.0001 |
Heart rate (bpm) | 80 ± 15 | 72 ± 18 | 74 ± 14 | 70 ± 16 | 0.2 | 0.2 |
IVS (mm) | 14 ± 0.8 | 11 ± 1.1 | 14 ± 0.8 | 10 ± 1.2 | 0.1 | 0.0001 |
IVSi (mm/m 2 ) | 6.2 ± 0.6 | 4.9 ± 0.8 | 7.5 ± 0.9 | 5.2 ± 0.7 | 0.0001 | 0.0001 |
RWT | 0.45 ± 0.05 | 0.35 ± 0.04 | 0.54 ± 0.07 | 0.37 ± 0.06 | 0.0001 | 0.0001 |
LVEDD < 51 mm | 7 (28) | 1 (4) | 18 (71) | 10 (40) | 0.02 | 0.0001 |
LVEDD < 54 mm | 15 (60) | 4 (16) | 20 (79) | 19 (76) | 0.2 | 0.0001 |
Left atrial diameter (mm) | 39 ± 4 | 37 ± 5 | 41 ± 5 | 37 ± 6 | 0.3 | 0.1 |
Stroke volume index (mL/m 2 ) | 43 ± 8 | 44 ± 9 | 37 ± 13 | 43 ± 10 | 0.07 | 0.3 |
E wave (cm/s) | 78 ± 15 | 92 ± 22 | 70 ± 19 | 83 ± 17 | 0.2 | 0.2 |
A wave (cm/s) | 57 ± 12 | 51 ± 11 | 77 ± 22 | 56 ± 12 | 0.002 | 0.4 |
E/A ratio | 1.4 ± 0.4 | 1.9 ± 0.6 | 0.9 ± 0.3 | 1.5 ± 0.4 | 0.0001 | 0.06 |
E time deceleration (ms) | 164 ± 38 | 193 ± 19 | 236 ± 72 | 179 ± 43 | 0.0009 | 0.2 |
s’ lateral (cm/s) | 8 ± 2 | 9 ± 2 | 7 ± 2 | 12 ± 3 | 0.08 | 0.0008 |
s’ septal (cm/s) | 7 ± 1 | 7 ± 1 | 7 ± 2 | 9 ± 3 | 0.9 | 0.0007 |
e’ lateral (cm/s) | 12 [5.5] | 14 [1.7] | 7 [3.1] | 18 [10.0] | 0.0001 | 0.0001 |
e’ septal (cm/s) | 10 [3.3] | 10 [2.5] | 7 [2.5] | 12 [4.0] | 0.0001 | 0.0001 |
E/e’ lateral ratio | 6.7 ± 2.0 | 6.5 ± 1.5 | 9.5 ± 2.8 | 5.3 ± 1.1 | 0.003 | 0.02 |
2D LVEDV (mL) | 163 ± 36 | 174 ± 29 | 111 ± 34 | 125 ± 30 | 0.0001 | 0.0001 |
2D LVESV (mL) | 68 ± 17 | 70 ± 15 | 42 ± 16 | 44 ± 12 | 0.0001 | 0.0001 |
3D LVEDV (mL) | 184 ± 35 | 182 ± 31 | 129 ± 32 | 135 ± 31 | 0.0001 | 0.0001 |
3D LVESV (mL) | 80 ± 15 | 74 ± 13 | 52 ± 17 | 51 ± 13 | 0.0001 | 0.0001 |
2D LVEDVi (mL/m 2 ) | 75 ± 15 | 80 ± 12 | 61 ± 21 | 66 ± 14 | 0.01 | 0.003 |
2D LVESVi (mL/m 2 ) | 32 ± 7 | 33 ± 6 | 23 ± 9 | 23 ± 6 | 0.002 | 0.0001 |
3D LVEDVi (mL/m 2 ) | 85 ± 13 | 82 ± 12 | 73 ± 19 | 72 ± 15 | 0.03 | 0.003 |
3D LVESVi (mL/m 2 ) | 37 ± 6 | 34 ± 6 | 30 ± 10 | 27 ± 7 | 0.007 | 0.0001 |
2D LVEF (%) | 58 ± 4 | 60 ± 5 | 62 ± 7 | 64 ± 3 | 0.02 | 0.0001 |
3D LVEF (%) | 56 ± 3 | 59 ± 4 | 60 ± 6 | 62 ± 4 | 0.007 | 0.0001 |
2D GLS (%) | 18 ± 2 | 19 ± 2 | 15 ± 3 | 19 ± 2 | 0.0001 | 0.2 |
3D GLS (%) | 18 ± 2 | 18 ± 3 | 14 ± 3 | 19 ± 3 | 0.0006 | 0.1 |
3D GRS (%) | 48 ± 18 | 47 ± 11 | 40 ± 10 | 50 ± 8 | 0.05 | 0.7 |
3D GCS (%) | 16 ± 2 | 17 ± 4 | 16 ± 3 | 17 ± 3 | 0.9 | 0.3 |
3D GAS (%) | 30 ± 3 | 30 ± 5 | 26 ± 5 | 31 ± 4 | 0.01 | 0.3 |
2D-16SD longitudinal (ms) | 39 ± 8 | 42 ± 9 | 66 ± 20 | 31 ± 9 | 0.0001 | 0.0001 |
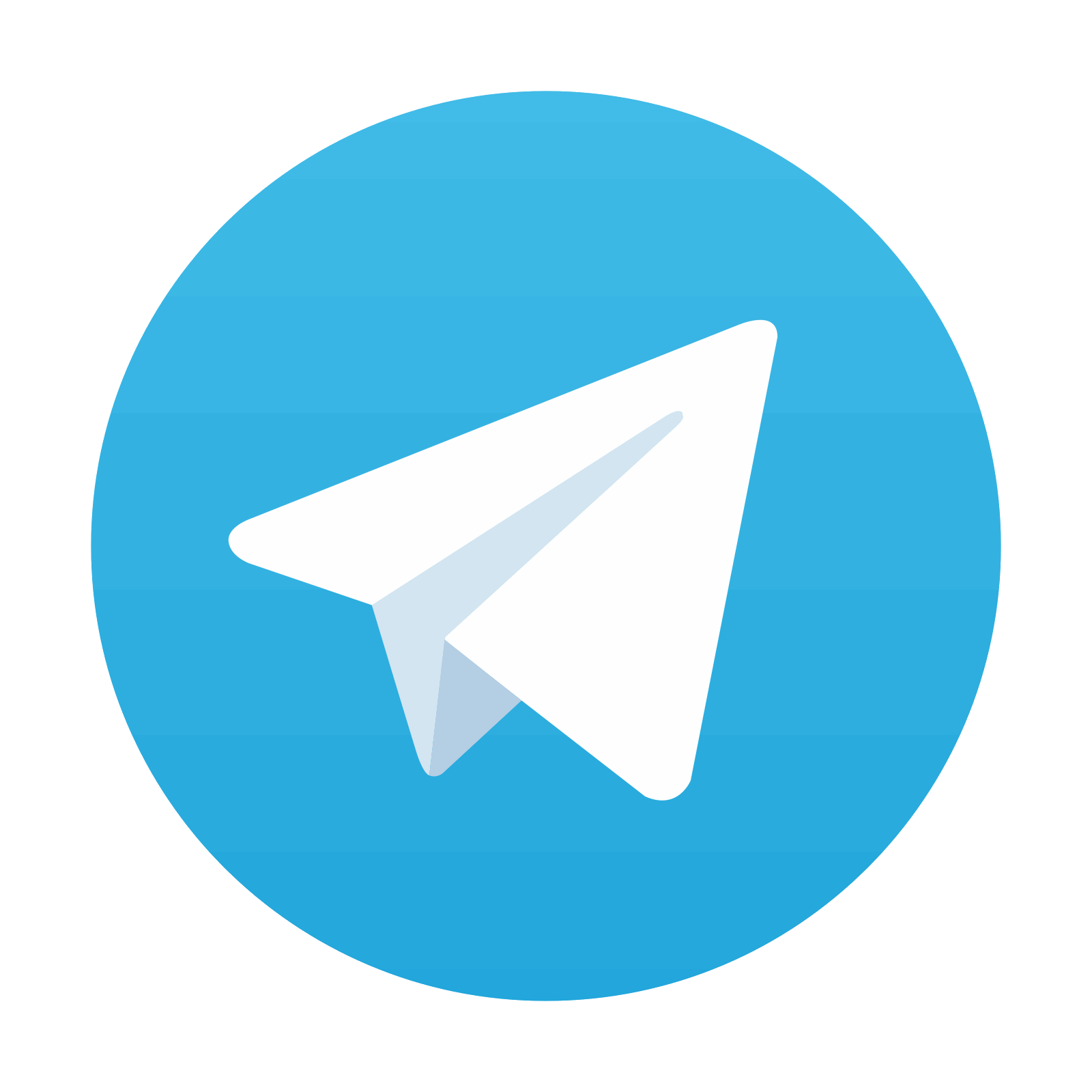
Stay updated, free articles. Join our Telegram channel

Full access? Get Clinical Tree
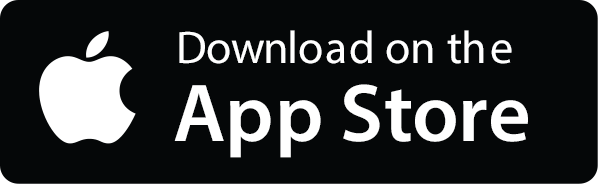
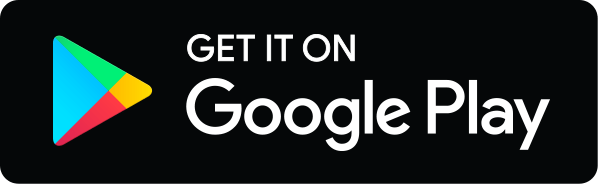
