Background
The role of speckle-tracking echocardiography in the assessment of chronic aortic regurgitation (AR) is not established. Load dependency may encumber the interpretation of strain measurements in the chronically overloaded left ventricle. The aim of this study was to investigate the mechanisms of left ventricular (LV) contraction patterns in asymptomatic patients with moderate to severe AR and preserved ejection fractions.
Methods
In this prospective, cross-sectional study, 31 patients with moderate to severe AR, 15 elite endurance athletes, and 17 healthy control subjects were examined using three-dimensional speckle-tracking echocardiography. Global circumferential strain (GCS), global longitudinal strain (GLS), end-systolic circumferential wall stress (ESSc), end-systolic meridional wall stress (ESSm), and the wall stress ratio (ESSc/ESSm) were measured.
Results
LV end-diastolic volumes were similar in athletes and patients with AR and significantly larger than in healthy control subjects. Values of GLS in control subjects, athletes, and patients with AR were −18.8 ± 1.9%, −17.3 ± 2.0%, and −16.4± 2.0%, respectively (control subjects vs athletes and patients, P < .05), whereas values of GCS were −16.9 ± 2.0%, −15.5 ± 1.9%, and −17.9 ± 2.6%, respectively (athletes vs control subjects and patients, P < .01). The ESSc/ESSm ratio was lower in patients with AR ( P < .01). When adjusted for ESSm, GLS remained impaired in patients compared with control subjects and athletes ( P = .015). On the other hand, GCS was better in patients with AR when adjusted for ESSc ( P = .003).
Conclusions
In compensated AR, relatively high GCS compensates for reduced GLS in a manner consistent with the preserved ejection fractions observed in these patients.
Highlights
- •
Three-dimensional speckle-tracking echocardiography enables the detection of subtle alterations in LV contractions in asymptomatic patients with chronic AR.
- •
LV longitudinal strain is impaired in these patients.
- •
Relatively augmented circumferential strain compensated for the impaired longitudinal strain.
- •
Myocardial strain in volume-loaded left ventricles must be interpreted in relation to LV geometry and fiber stress distribution.
- •
Even after adjusting for the relatively increased longitudinal wall stress, longitudinal strain is decreased in patients with chronic AR.
Aortic regurgitation (AR) is associated with volume and pressure overload. The left ventricle responds to the increased load with a series of compensatory mechanisms, including eccentric hypertrophy and increased end-diastolic volume (EDV). This remodeling is at least partly adaptive, enabling the left ventricle to maintain a normal net forward cardiac output. With increasing left ventricular (LV) size, however, the risk of myocardial failure increases. Once LV dysfunction is established, the long-term outcome may be significantly impaired. However, subtle LV dysfunction may develop long before it can be detected by conventional two-dimensional (2D) echocardiography.
Over the past decade, measurements of myocardial deformation have been applied to detect early changes in LV function. A consistent observation has been that a reduction in longitudinal deformation velocities may occur while LV ejection fraction (LVEF) is still in the normal range. The mechanisms behind these early changes are not fully understood. When examining patients with AR, one must be able to discern incipient LV dysfunction, possibly heralding the onset of myocardial failure, from adaptive, physiologic changes as observed in endurance athletes. The increased LV volume in patients with chronic AR induces eccentric hypertrophy. These factors affect myocyte afterload as measured by wall stress, and myocardial deformation must be interpreted with due regard to wall stress.
This study was designed to analyze multidirectional LV strain in patients with AR using state-of-the-art, real-time three-dimensional (3D) echocardiography. We aimed to show that subtle changes in contraction patterns are present in asymptomatic patients with hemodynamically significant AR. We hypothesized that in these patients, there would be a disproportionate reduction in LV longitudinal strain and strain rate, related to a relative increase in end-systolic meridional (longitudinal) wall stress. To account for the confounding effect of LV dilation, we compared our results with measurements obtained in elite endurance athletes with similar-sized, volume-loaded ventricles as well as with healthy control subjects.
Methods
Study Population
We prospectively recruited patients with AR from the outpatient cardiac clinic at Oslo University Hospital and from referring hospitals in the South-Eastern Norway Regional Health Authority and Haukeland University Hospital (Bergen, Norway). The patients were invited to participate in the β-Blocker for Aortic Regurgitation study, investigating the effect of controlled-release metoprolol on LV remodeling in AR. We included symptomatic patients with moderate to severe AR who did not meet the prevailing criteria for aortic valve replacement. Patients with aortic stenosis (valve area < 1.5 cm 2 ), cardiac arrhythmia, or other cardiovascular diseases were excluded. The size of the AR was assessed using an integrative approach combining clinical evaluation, valvular morphology, and Doppler and volumetric measurements. All measurements were obtained before treatment with metoprolol or placebo, and no patient received β-receptor antagonists at baseline.
Healthy, age-matched control subjects were recruited through local announcements among employees and students at Oslo University Hospital. We selected 15 age-matched elite endurance athletes through the Norwegian Olympic Sports Centre (Oslo, Norway). Among the athletes there were three cross-country skiers, six triathlon athletes, five long-distance runners, and one cyclist, all competing at an international level and with a training load >800 h/yr. Exclusion criteria for athletes and healthy control subjects were a history of cardiovascular disease, the presence of electrocardiographic abnormalities, and hypertension. Blood pressure was measured in the supine position after a minimum of 5 min of rest, using an oscillometric device and is reported as the average of three recordings. All participants provided written informed consent. The study protocol was approved by the regional committee for medical and health research ethics (REC South-East).
Echocardiography
Echocardiographic examinations were performed at two Norwegian centers (Oslo University Hospital, Rikshospitalet, and Haukeland University Hospital, Bergen). Recordings (2D and 3D) were obtained using Vivid E9 ultrasound scanners (GE Vingmed Ultrasound AS, Horten, Norway). We obtained 3D full-volume data from the apical position (4V probe; GE Vingmed Ultrasound AS). All echocardiograms were analyzed in a blinded manner at the echocardiography core laboratory at Oslo University Hospital using EchoPAC SW 12 (GE Vingmed Ultrasound AS). For all measurements, we used the average of three heart cycles. We obtained LV internal diameters from M-mode recordings or anatomic M-mode if the angle of the ultrasound beam deviated >30° from an axis perpendicular to the interventricular septum. LV mass was calculated from 2D echocardiography by M-mode. LV volumes and LVEF were assessed from 3D full-volume recordings. The regurgitant vena contracta (jet width) was measured just below the aortic valve. The regurgitant volume was calculated as the difference between the LV stroke volume by 3D echocardiography and the estimated right ventricular stroke volume, assuming no intracardiac shunt. We estimated right ventricular stroke volume by calculating right ventricular outflow area using the right ventricular outflow diameter and multiplying this area by the velocity-time integral. The regurgitant fraction was calculated as the percentage of the regurgitant volume to the LV stroke volume by 3D echocardiography. Pulsed-wave tissue Doppler velocities were obtained in the basal segments of the septal, lateral, anterior, and inferior walls. For assessment of reproducibility, 20 examinations were independently reviewed by two echocardiographers, who were blinded to the results of each other’s analyses.
Measures of LV remodeling included the sphericity index (SI = EDV/[4/3 × π × ( L i /2)] 3 ), where EDV was assessed using the Simpson biplane method and L i is LV internal diameter from the atrioventricular plane to the apex at end-diastole; the LV short axis/long axis ratio at end-diastole; and the LV mass/EDV ratio. We also applied a fourth index, assuming a prolate ellipsoid ventricle ( Figure 1 ): the ratio between the LV short-axis diameter at the “equator” and the distance ( B i ) between the “equator” and LV apex.

Three-dimensional strain analysis was conducted using automated LV quantification (version BT12; GE Vingmed Ultrasound AS). After manual alignment of the three apical views and the short-axis view, points were placed in the mitral annular plane and the apex in end-diastole and end-systole. End-systole, defined at the frame with the smallest LV cavity, was automatically detected by the software, but manually corrected if necessary. End-diastolic and end-systolic volumes were measured by semiautomatic detection of the endocardial surface, with manual adjustment, and 3D LVEF was calculated. The software automatically detected the epicardial border, but we made manual adjustments to delineate the region of interest for the analysis of end-systolic 3D longitudinal and circumferential strain. The strain values were calculated using a midwall tracking algorithm based on frame-to-frame block matching in three dimensions in a 17-segment model. Finally, the tracking of each segment was manually controlled, and segments with suboptimal tracking were rejected. Three-dimensional global longitudinal strain (GLS) and global circumferential strain (GCS) were calculated by averaging the end-systolic segmental values when ≥12 segments had acceptable tracking. We exported 3D strain data derived from the full-volume data sets to Excel (Microsoft, Redmond, WA) for further analysis. Global strain rates were calculated as the time derivatives of the strain curves (1/s). End-systole was defined as the time frame at aortic valve closure as estimated by pulsed-wave Doppler.
LV end-systolic pressures in healthy control subjects and athletes were estimated using the formula (2 × BP syst + BP diast )/3, where BP syst is systolic brachial pressure and BP diast is diastolic brachial pressure. In patients with AR, we measured end-systolic pressures by applanation tonometry. Brachial and carotid artery pulse waves were recorded with the use of a high-fidelity applanation tonometric device (Millar SPT-301; Millar Instruments, Houston, TX). The pulse curves were calibrated to the brachial cuff pressure, and the dicrotic notch in the carotid pressure curve was taken to represent LV end-systolic pressure.
Wall Stress
LV afterload can be assessed as wall stress, an expression of the stress supported by myocardial fibers, excluding the intramyocardial pressure. Assuming a homogenous, isotropic, and ellipsoid ventricle, we calculated circumferential (σ u ) and meridional (σ v ) wall stress according to Regen :
σ u = p LV a m 2 h e ( 2 − a m 2 b m 2 ) and σ v = p LV a m 2 h e ,
a m = ( a o − a i ) ln a o − ln a i and b m = ( b o − b i ) ln b o − ln b i ,
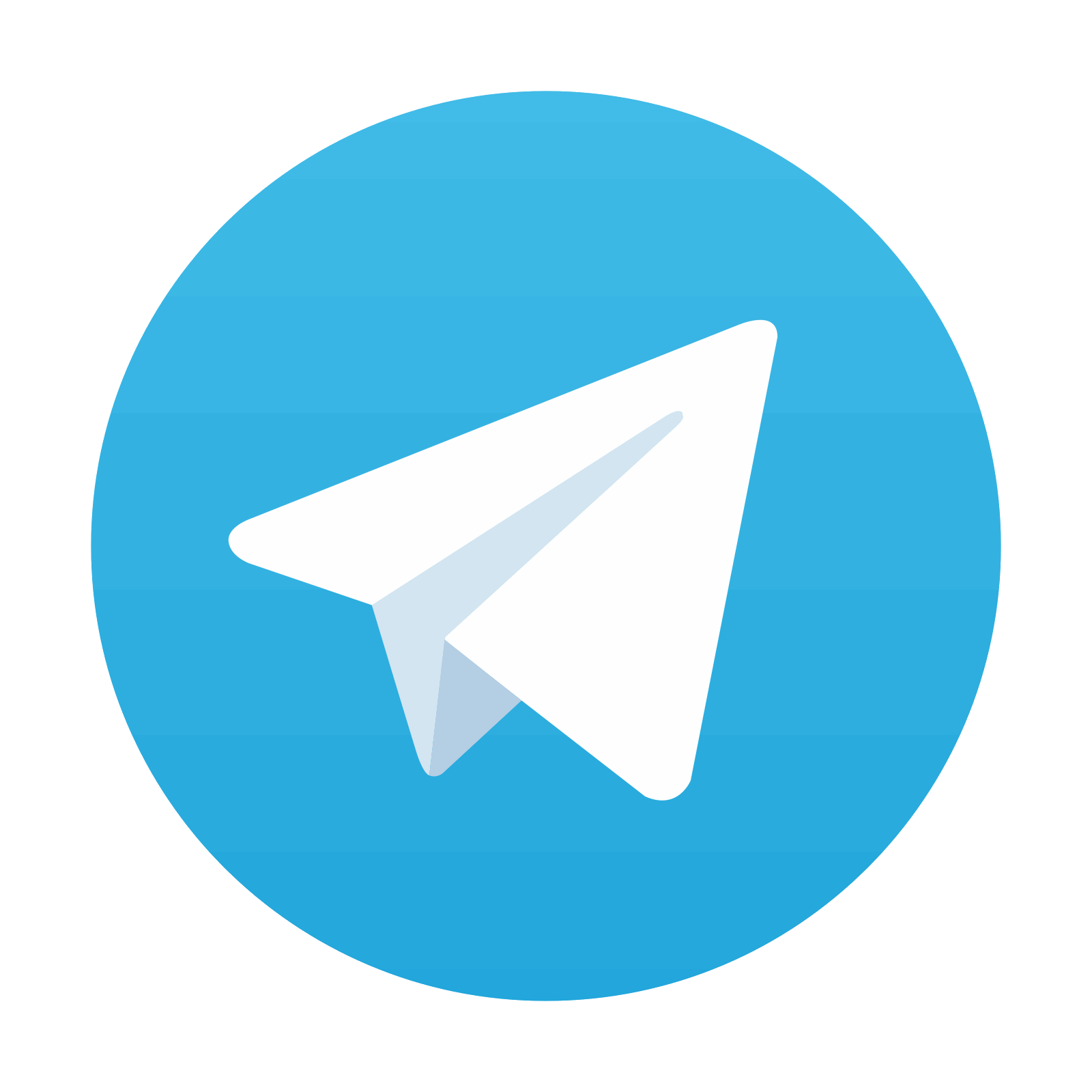
Stay updated, free articles. Join our Telegram channel

Full access? Get Clinical Tree
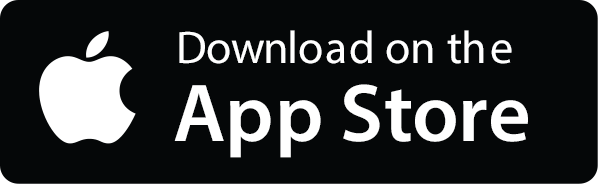
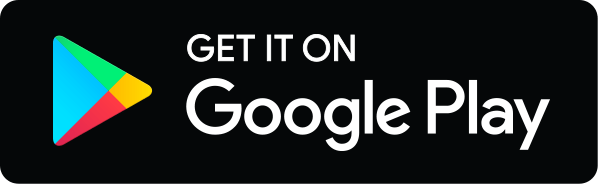