Background
Ventricular-ventricular interactions may affect left ventricular (LV) and right ventricular (RV) function but have not been well characterized in chronic LV afterload in children. The aim of this study was to assess RV myocardial strain in children with aortic stenosis before and after aortic balloon valvuloplasty.
Methods
Two-dimensional echocardiographic images from children aged > 1 month were with aortic stenosis and preserved LV ejection fractions were retrospectively studied using vector velocity imaging. LV and RV strain were compared before and after balloon valvuloplasty and in comparison with normal controls.
Results
Twenty-six children were studied. Aortic valve gradient decreased after balloon valvuloplasty. LV ejection fraction, wall thickness, circumferential strain, and basal and mid longitudinal strain were unchanged after valvuloplasty (−18.09 ± 6.97% vs −16.43 ± 6.30%, P = .40, and −14.11 ± 5.011% vs −13.12 ± 5.52%, P = .50, respectively). LV strain tended to be lower than in controls after valvuloplasty (basal, −16.43 ± 6.30% vs −19.77 ± 5.82%, P = .05). RV strain was unchanged at the basal and apical segments but increased at the mid RV segment after valvuloplasty (−21.34 ± 6.55% vs −24.97 ± 8.54%, P = .02). Change in RV strain was not correlated with change in aortic gradient or change in LV strain after valvuloplasty ( P = .60). LV and RV strain at baseline and their changes after valvuloplasty were variable between patients.
Conclusions
RV strain was normal or reduced in compensated aortic stenosis and was not correlated with LV strain. The change in RV strain was variable among patients after valvuloplasty, with improvement in RV midwall longitudinal strain.
Ventricular-ventricular interactions may profoundly influence cardiac function through several mechanisms, including the position of the interventricular septum and shared myofibers, which run obliquely in the superficial layers of the right and left ventricles between the two ventricles. Consequently, in experimental models, as well as in vivo experiments, developed pressure in the right ventricle depends to a large extent on left ventricular (LV) contraction. In clinical settings, the function of the contralateral ventricle is an important determinant of clinical outcomes. For example, in LV dilated cardiomyopathy, right ventricular (RV) function is an important determinant of outcomes. Conversely, in right-sided disease, LV function determines morbidity and mortality.
Although experimentally, the entire RV free wall can be removed and pressure will still develop after LV contraction, it is to a large extent through the shared myofibers that LV contraction affects RV function. In an experimental model of severe RV afterload and failure, aortic constriction led to improved RV function independent of changes in right coronary artery flow. We recently demonstrated in a rabbit model of acute RV afterload and failure that moderately increased LV afterload induced by aortic constriction or epinephrine and norepinephrine leads to improved RV contractility. This presumably occurs because the left ventricle, provided it has sufficient reserve, increases its contractility to overcome the additional afterload. The increased contractility may be transferred to the right ventricle through the shared myofibers between the left and right ventricles. Additional mechanisms may also be at play. However, these interactions have not been well described in chronic clinical disease in children.
Aortic stenosis (AS) is characterized by increased LV afterload and usually preserved LV chamber performance until late in the clinical course. Therefore, compensated AS provides a clinical model to study effects of LV afterload on RV myocardial performance. Although LV myocardial deformation and performance have been extensively researched in this condition, there are scant data published on RV myocardial strain in patients with AS. The objective of this study was to study RV myocardial strain in children with AS before and after balloon valvuloplasty as a clinical model of ventricular-ventricular interactions in chronic stable LV afterload. Given the aforementioned increase in RV contractility after aortic constriction, we hypothesized that RV strain is increased in AS and decreases after aortic balloon valvuloplasty.
Methods
A sample of consecutive children aged > 1 month who had undergone echocardiography before and after balloon valvuloplasty for AS between 2007 and 2009 was studied. We analyzed the last echocardiogram before aortic valvuloplasty and the first echocardiogram after valvuloplasty. Patients had to have preserved LV ejection fractions (>55%) to be included. Children aged < 1 month were excluded to exclude neonatal critical AS, which has distinct pathophysiology, often with decreased LV function and often dependent on the patency of the arterial duct. Controls were healthy volunteers or healthy children undergoing echocardiography for evaluation of innocent murmurs. Controls had normal histories and normal results on physical examination and echocardiography. Controls were matched to patients for age and, when possible, by gender.
Echocardiographic Image Acquisition
Children were scanned using a Vivid 7 (GE Healthcare, Wauwatosa, WI), iE33 (Philips Medical Systems, Andover, MA), or ATL ultrasound system (ATL, Seattle, WA) using transducer frequencies appropriate for body size. Echocardiography was performed at rest in the left lateral decubitus position during sinus rhythm, and images were acquired during shallow respiration. M-mode imaging from the parasternal short-axis or long-axis views was used to calculate LV dimensions and shortening fraction. Continuous-wave Doppler in the LV outflow tract from the apical, high parasternal, or suprasternal notch views was used to calculate the peak and mean gradients across the aortic valve. Sweep speed was 50 to 100 cm/sec. Two-dimensional apical four-chamber and short-axis images at the papillary muscle level stored in Digital Imaging and Communications in Medicine (DICOM) format were acquired for offline analysis of LV and RV longitudinal strain and LV circumferential strain. DICOM images were routinely stored at 30 frames/sec.
Offline Analysis
Indices of RV and LV Function
LV chamber and myocardial function were assessed using end-diastolic dimension and Z score, and ejection fraction and septal and posterior wall thickness were assessed from a parasternal short-axis or long-axis M-mode acquisition. RV end-diastolic dimension and its corresponding Z score, fractional area of change ([RV end-diastolic area−RV end-systolic area]/RV end-diastolic area), RV basal systolic displacement, and RV longitudinal strain were used as parameters of RV chamber and myocardial function.
Cardiac Performance Analysis
Echocardiographic DICOM images were uploaded into analysis software (Cardiac Performance Analysis Systems version 1.0, TomTec Medical Systems, Unterschleissheim, Germany) and analyzed as previously described. The endocardial border was manually traced at end-systole (minimal volume) in the apical four-chamber view for longitudinal LV and RV strain and in the parasternal short-axis view for LV circumferential strain. Septal strain was measured from the LV tracing. Tracking of the endocardial border was visually inspected over the cardiac cycle and manually adjusted if necessary. After obtaining satisfactory endocardial tracking, the epicardial border was added to incorporate the entire myocardial wall. Tracking was reinspected throughout the cardiac cycle and adjusted as necessary. Lagrangian strain curves were then rendered by the software. The cardiac cycle with the best tracking and visually most credible strain curves was selected for analysis. We previously reported on the feasibility and reliability of this technique in children for the assessment of myocardial strain.
Statistical Analysis
Demographic data are expressed as medians and interquartile ranges. Hemodynamic data are expressed as mean ± SD. LV and RV strain was compared between controls, patients before, and patients after valvuloplasty using one-way analysis of variance with the post hoc Bonferroni test. Echocardiographic parameters including strain were compared before and after valvuloplasty in individual patients using two-way paired Student’s t tests. Correlations between change in valve gradient and change in myocardial strain and between LV and RV longitudinal strain were assessed using Pearson’s linear regression. GraphPad Prism version 5.04 (GraphPad Software Inc., La Jolla, CA) was used for analysis. The study was approved by the institutional review board of the Hospital for Sick Children in Toronto.
Intraobserver and Interobserver Reliability Analysis
Intraobserver and interobserver reliability analysis of peak global LV (average of LV and basal segments) and RV (average of RV lateral wall segments) longitudinal strain was performed in 10 randomly chosen patients. Curves were traced anew, and strain curves generated anew, on the same cardiac cycle by the same observer and independently by a second observer for intraobserver and interobserver reliability, respectively. The coefficient of variation (absolute difference in percentage of the mean of repeated measurements) was calculated to analyze intraobserver and interobserver reliability.
Results
Study Population and Hemodynamics
Twenty-six children were studied before and after balloon aortic valvuloplasty. Their demographics, as well as those of 26 age-matched controls, are shown in Table 1 . All patients except one had either bicuspid ( n = 23 [88%]) or unicuspid ( n = 2 [8%]) aortic valves. One patient had a tricuspid dysplastic valve. The indications for aortic balloon valvuloplasty were standard clinical indications, mainly clinical status, ventricular hypertrophy, or valve gradient on echocardiography. Patients and controls were well matched in terms of age, size, and gender ( Table 1 ). In patients, echocardiography was performed at a median interval of 25 days (range, 1–176 days) before and 12 days (range, 1–114 days) after aortic balloon valvuloplasty. Echocardiographic hemodynamic and ventricular function data are shown in Table 2 . After balloon valvuloplasty, aortic valve peak and mean gradient decreased significantly. LV ejection fraction, dimensions, and septal and posterior wall thickness were unchanged after the procedure. RV dimensions, fractional area change, and RV basal longitudinal displacement were unchanged after the procedure. Before the procedure, 17 patients had no or trivial aortic insufficiency (AI), seven had mild AI, and two had moderate AI. None had severe AI. After the procedure, AI increased from none or trivial to mild in eight patients, increased from none to moderate in one, and decreased from mild to none in one. AI severity was unchanged in the remainder.
Characteristic | Patients | Controls | P |
---|---|---|---|
Age (y) | 10 (0.55–13.00) | 10 (0.56–13.25) | .90 |
Height (cm) | 147 (77.00–163.00) | 137.5 (69.25–158.3) | .54 |
Weight (kg) | 40 (7.15–59.55) | 33.9 (8.07–44.48) | .63 |
Body surface area (m 2 ) | 1.3 (0.35–1.63) | 1.18 (0.37–2.09) | .71 |
Male sex | 18 (69%) | 16 (62%) |
Variable | Before valvuloplasty | After valvuloplasty | P |
---|---|---|---|
Peak aortic valve gradient (mm Hg) | 113 ± 24 | 54 ± 17 | <.0001 |
Mean aortic valve gradient (mm Hg) | 60 ± 12 | 29 ± 11 | <.0001 |
LV end-diastolic dimension (cm) | 3.7 | 3.9 | .008 |
LV end-diastolic dimension Z score | 0 | 0.5 | .60 |
LV ejection fraction (%) | 77 ± 5 | 76 ± 7 | .97 |
Septal thickness (cm) | 0.79 ± 0.27 | 0.79 ± 0.30 | .85 |
LV posterior wall thickness (cm) | 0.84 ± 0.40 | 0.74 ± 0.25 | .20 |
RV end-diastolic dimension (cm) | 1.68 | 1.63 | .60 |
RV end-diastolic dimension Z score | 0.6 | 0.5 | .36 |
RV fractional area change (%) | 43 ± 13 | 48 ± 12 | .13 |
RV basal displacement (mm) | 11 ± 4 | 12 ± 5 | .31 |
LV Strain
Figure 1 A depicts LV longitudinal strain before and after balloon valvuloplasty in patients with AS and in controls. On average, LV longitudinal strain at the basal and mid lateral walls was unchanged after the procedure (−18.09 ± 6.97% vs −16.43 ± 6.30%, P = .40, and −14.11 ± 5.011% vs −13.12 ± 5.52%, P = .50, respectively). Averaged LV basal longitudinal strain was not different between patients with AS and controls before valvuloplasty (−18.09 ± 6.97% vs −19.77 ± 5.82%, P = .35), but there was a trend toward reduced strain after valvuloplasty compared with controls (−16.43 ± 6.30% vs −19.77 ± 5.82%, P = .05). LV circumferential strain was unchanged after the procedure (−25.47 ± 5.65% vs −24.88 ± 5.99%, P = .60). Figure 1 B depicts septal longitudinal strain at the basal and mid segments before and after valvuloplasty in patients with AS and in controls. On average, septal longitudinal strain at the basal and mid levels was unchanged after the procedure (−13.21 ± 4.371% vs −11.87 ± 4.726%, P = .20, and −12.99 ± 3.66% vs −12.40 ± 4.92%, P = .5). Septal basal and mid strain were lower than in controls before and after valvuloplasty. Global longitudinal LV strain (average of lateral wall and septal segments) was not significantly changed before and after the procedure (−14.5 ± 3.2% vs −13.2 ± 4.3%, P = .20). The change in longitudinal LV strain (mid segment) was not correlated with the change in peak or mean aortic valve gradient.
RV Strain
Figure 1 C depicts RV longitudinal strain before and after the procedure. RV strain at the mid segment increased after balloon valvuloplasty (−21.34 ± 6.55% vs −24.97 ± 8.54%, P = .02). RV strain at the basal (−26.04 ± 7.79% vs −24.77 ± 7.10%, P = .49) and apical (−16.64 ± 5.48% vs −18.74 ± 8.78%, P = .33) segments were not different before and after balloon valvuloplasty. Global RV strain in patients with AS was significantly lower than in controls (−22.64 ± 4.1%) before (−17.86 ± 3.63%, P < .001) and after (−17.36 ± 4.62%, P < .001) valvuloplasty but not significantly changed in patients before and after the procedure ( P > .05). The average change in lateral wall RV strain after valvuloplasty was not correlated with the average change in LV strain after valvuloplasty ( P = .60). When assessing regional effects, there was no correlation between change in either septal or LV lateral wall longitudinal strain before and after valvuloplasty with the change in RV lateral wall longitudinal strain. The change in longitudinal lateral wall RV strain (mid segment) was not correlated with the change in peak or mean aortic valve gradient.
Change in Strain in Individual Patients
Figure 2 depicts the change in LV, septal, and RV strain before and after valvuloplasty in individual patients. Observation of the before and after strain values shows variable baseline values between patients, as well as a variable response between patients in LV and RV strain response after aortic valvuloplasty.
The change in LV strain was linearly related to preprocedural LV strain. The lower the preprocedural LV longitudinal strain, the larger the improvement in strain after aortic valvuloplasty ( Figure 3 A). The relation between preprocedural RV strain and change in RV strain was weaker than in the left ventricle and not statistically significant, in part because of one outlier ( r = −0.40, P = .09; Figure 3 B). When analyzing the correlation in the right ventricle without this patient, the correlation remained weak, with considerable scatter, but was statistically significant ( r = −0.54, P = .02). Likewise, when analyzed as a relation between baseline RV strain and the percentage change in strain after aortic valvuloplasty compared with baseline, the relation was statistically significant ( r = 0.49, P = .03). Consequently, as can be observed in Figure 2 , although not universal, patients with the highest strain in the LV and RV free walls before valvuloplasty experienced decreases in strain after the procedure, whereas patients with lower strain before the procedure commonly experienced improved LV and RV longitudinal deformation. As Figure 2 suggests that after aortic valvuloplasty, there were patients with increased LV and RV strain responses compared with those with reduced or unchanged strain response, we further compared the characteristics of these two subgroups ( Tables 3 and 4 ). The only significant difference between patients with improved versus unchanged or decreased strain flowing balloon valvuloplasty was that preprocedural LV strain was lower in patients who experienced increased LV strain after the procedure. This is consistent with the previously described correlation between preprocedure LV strain and the change in LV strain ( Figure 3 A).
Variable | Increased LV lateral wall strain | Unchanged or decreased LV lateral wall strain | P |
---|---|---|---|
Age (y) | 9.6 ± 6.0 | 6.7 ± 6.4 | .28 |
Male sex | 58% | 78% | |
Interval between preprocedural echocardiography and catheterization (d) | 41 ± 61 | 53 ± 63 | .63 |
Interval between postprocedural echocardiography and catheterization (d) | 25 ± 24 | 38 ± 48 | .44 |
Preprocedural LV ejection fraction (%) | 75.43 ± 6.80 | 77.16 ± 4.77 | .48 |
Preprocedural peak aortic gradient (mm Hg) | 120.38 ± 15.75 | 110.16 ± 26.51 | .32 |
Preprocedural mean aortic gradient (mm Hg) | 64.08 ± 11.24 | 58.04 ± 12.22 | .24 |
Postprocedural peak aortic gradient (mm Hg) | 60.88 ± 14.89 | 50.42 ± 17.32 | .15 |
Postprocedural mean aortic gradient (mm Hg) | 32.70 ± 7.44 | 27.14 ± 11.87 | .23 |
Preprocedural interventricular septal thickness (cm) | 0.97 ± 0.20 | 0.72 ± 0.27 | .03 |
Preprocedural LV lateral basal longitudinal strain (%) | −12.46 ± 5.43 | −20.74 ± 6.06 | .003 |
Preprocedural LV lateral mid longitudinal strain (%) | −11.68 ± 3.41 | −15.26 ± 5.31 | .09 |
Preprocedural septal basal longitudinal strain (%) | −10.59 ± 4.23 | −14.38 ± 4.01 | .03 |
Preprocedural septal mid longitudinal strain (%) | −13.46 ± 3.10 | −12.78 ± 3.96 | .60 |
Preprocedural RV lateral basal longitudinal strain (%) | −23.52 ± 7.22 | −27.62 ± 8.73 | .31 |
Preprocedural RV lateral mid longitudinal strain (%) | −21.88 ± 4.83 | −22.50 ± 8.37 | .86 |
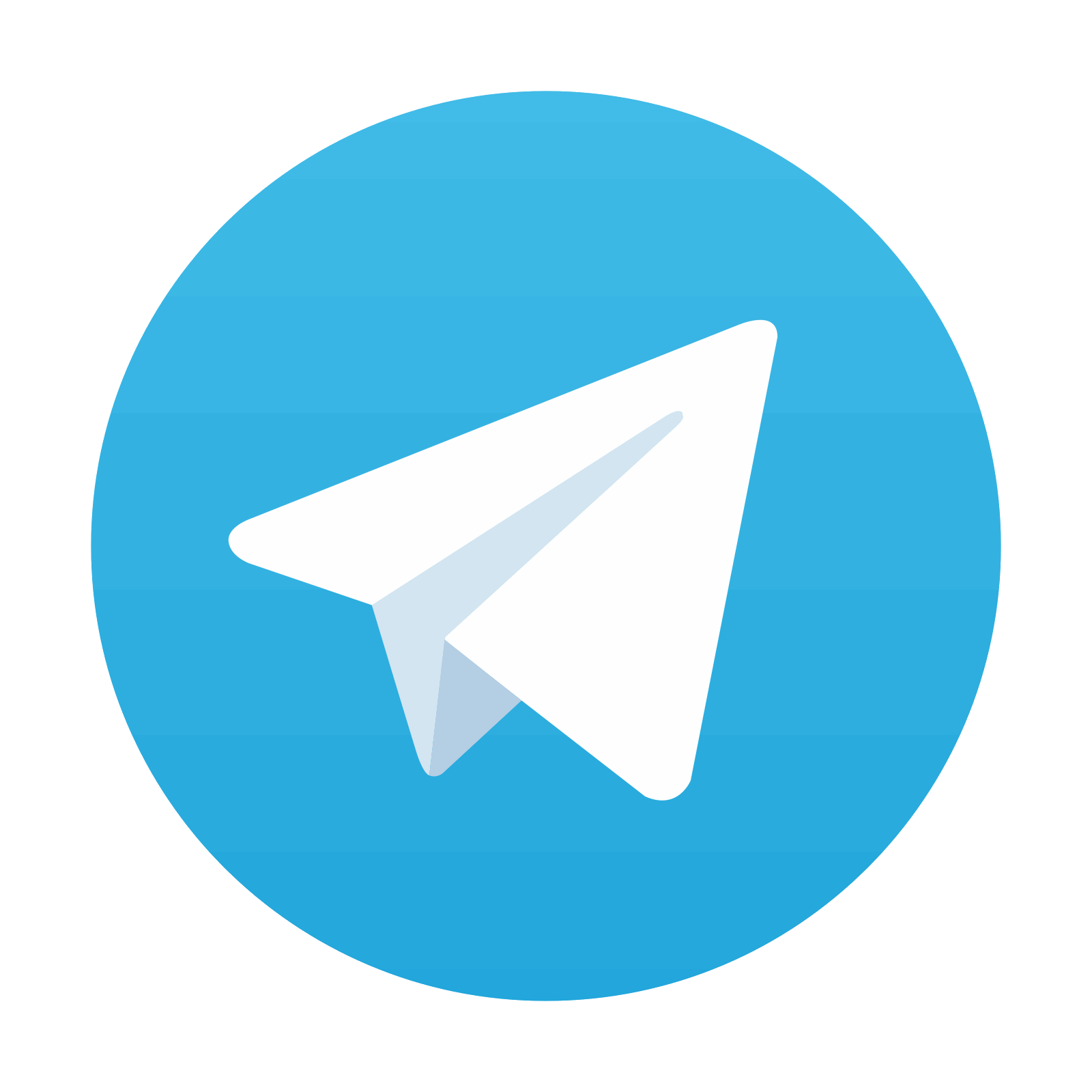
Stay updated, free articles. Join our Telegram channel

Full access? Get Clinical Tree
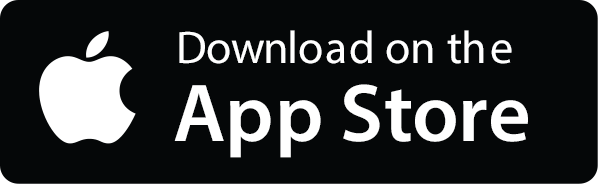
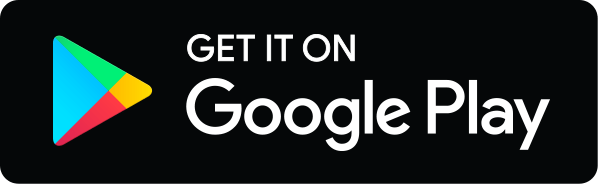
