Background
Left atrial (LA) strain (ϵ) and ϵ rate (SR) analysis by two-dimensional speckle tracking can represent a new tool to evaluate LA function. To assess its potential value, the authors addressed whether LA ϵ and SR measured in normal subjects correlates with other Doppler echocardiographic parameters that evaluate LA function and left ventricular function.
Methods
Sixty-four healthy subjects were studied. LA ϵ and SR were calculated with the reference point set at the P wave, which enabled the recognition of peak negative ϵ (ϵ neg peak ), peak positive ϵ (ϵ pos peak ), and the sum of those values, total LA ϵ (ϵ tot ), corresponding to LA contractile, conduit, and reservoir function, respectively. Similarly, peak negative SR (LA SR late neg peak ) during LA contraction, peak positive SR (LA SR pos peak ) at the beginning of LV systole, and peak negative SR (LA SR early neg peak ) at the beginning of LV diastole were identified.
Results
Global LA ϵ pos peak , ϵ neg peak , and ϵ tot were 23.2 ± 6.7%, −14.6 ± 3.5%, and 37.9 ± 7.6%, respectively. Global LA SR pos peak , SR early neg peak , and SR late neg peak were 2.0 ± 0.6 s −1 , −2.0 ± 0.6 s −1 , and −2.3 ± 0.5 s −1 , respectively. The above-described variables derived from analysis of global LA ϵ and LA SR correlated significantly with Doppler echocardiographic indexes that evaluated the same phase of the cardiac cycle or the same component of the LA function, including indexes derived from mitral inflow, pulmonary vein velocities, tissue Doppler, and LA volumes. Global LA ϵ pos peak , LA ϵ tot , and LA SR early neg peak also correlated significantly with age or body mass index. Global LA SR late neg peak also correlated significantly with age.
Conclusions
LA ϵ analysis is a new tool that can be used to evaluate LA function. Further studies are warranted to determine the utility of LA ϵ in disease states.
The left atrial (LA) volume index is a recognized prognostic marker in diverse conditions, such as heart failure, myocardial infarction, and atrial fibrillation. Moreover, LA function has also been described as a prognostic indicator. As we improve the evaluation of LA function, it may emerge as an important component in the evaluation of a number of diseases, such as atrial arrhythmias, heart failure, and mitral valve disease.
Invasive measurements of LA function are not feasible in most patients. Therefore, the components of LA function (contractile, conduit, and reservoir function) are traditionally estimated using two-dimensional (2D) echocardiography and Doppler analysis of transmitral and pulmonary vein flows. However, the evaluation of LA function is still challenging. The evaluation of LA volumes by 2D echocardiography is limited by the use of geometric models to determine the volume of a nonsymmetric chamber and by errors due to foreshortening. Thus, 2D echocardiography may underestimate LA volumes compared with three-dimensional methods. The evaluation of LA function by Doppler analysis of transmitral and pulmonary vein flows is indirect and therefore also limited.
Two-dimensional speckle tracking is a new echocardiographic tool that tracks the speckle pattern frame by frame in standard B-mode images to calculate left ventricular (LV) strain (ϵ). This analysis may allow a more direct assessment of LA endocardial contractility and passive deformation and has been recently proposed, but data on normal values for LA ϵ and ϵ rate (SR) are still scarce, and the values found have not been correlated with traditional 2D Doppler echocardiographic parameters.
Therefore, our aims were to evaluate the components of LA function by 2D speckle tracking in normal subjects and to correlate the values found with traditional 2D Doppler echocardiographic measurements of LA function and LV function.
Methods
Patients
We retrospectively examined images from healthy volunteers who were examined in our echocardiographic laboratory from 2005 to 2006. Subjects were declared healthy after undergoing thorough medical histories and physical examinations. All subjects had normal findings on resting electrocardiography and baseline echocardiography. All subjects gave written informed consent before their participation. A previous work describing LV ϵ using this same database was recently published.
We identified 97 normal subjects in our database. Of these, 33 subjects were excluded from analysis for inadequate electrocardiograms (n = 6) or inadequate imaging quality due to acquisition with low frame rates (n = 13), LA foreshortening (n = 7), or inadequate acoustic windows (n = 7). The final study population consisted of 64 individuals. Among them, 10 patients did not have adequate 3-chamber views for analysis and had only 4-chamber and 2-chamber views analyzed.
Echocardiography
Studies were performed using phased-array ultrasound systems (Vivid 7; GE Medical Systems, Milwaukee, WI) equipped with 3S phased-array transducers. Cardiac dimensions were measured in accordance with the recommendations of the American Society of Echocardiography. Echocardiograms were stored digitally and reviewed offline with software (ProSolv Cardiovascular Analyzer; Problem Solving Concepts, Indianapolis, IN). The values for 2D echocardiographic parameters were obtained after averaging 3 consecutive cycles.
M-mode echocardiography was used to measure LA diameter and LV end-diastolic and end-systolic diameters. LV and LA volumes were determined using the modified Simpson’s rule with images obtained from apical 4-chamber and 2-chamber views. Pulsed-wave Doppler was obtained in the apical 4-chamber view. From transmitral recordings, the peak early (E) and late (A) diastolic filling velocities, E/A ratio, E-wave deceleration time, E-wave velocity-time integral (VTI E ), A-wave VTI (VTI A ), and LA filling fraction ([(VTI A /(VTI E + VTI A )] × 100) were obtained. From pulmonary vein velocities obtained at the right upper pulmonary vein, the following measurements were taken: peak S-wave inflow velocity during ventricular systole, peak D-wave inflow velocity during the early phase of ventricular diastole and the corresponding S/D ratio, peak reversed atrial wave (Ar) velocity during LA contraction, S-wave VTI, D-wave VTI, and Ar-wave VTI.
Doppler tissue imaging of the mitral annular level was obtained at the septal and lateral positions. Values shown for peak early (E′) and late (A′) diastolic annular velocities are averages of the values obtained at septal and lateral positions.
The following indexes of LA function were calculated according to previous study. Total LA stroke volume was calculated as maximum LA volume − minimum LA volume. Active LA stroke volume was calculated as precontraction LA volume − minimum LA volume. Passive LA stroke volume was calculated as maximum LA volume − precontraction LA volume. The total LA emptying fraction was calculated as (total LA stroke volume/maximum LA volume) × 100. The active LA emptying fraction was calculated as (active LA stroke volume/precontraction LA volume) × 100. The passive LA emptying fraction was calculated as (passive LA stroke volume/maximum LA volume) × 100. The LA expansion index was calculated as (total LA stroke volume/minimum LA volume) × 100.
Longitudinal ϵ Analysis
The images used for LV ϵ analysis were acquired focusing the LV by adjusting the depth and frame rate. LV longitudinal ϵ was calculated as previously described.
The clips used for LA ϵ and SR analysis were different from those used for LV ϵ analysis but were acquired during the same exam. Only clips with good quality images, enough depth to include the whole LA and acquired with high frame rates were used for analysis. The average frame rate of the clips used for LA ϵ analysis was 64.1 ± 6.6 frames/s.
In contrast to the assessment of LV ϵ, in which the R-wave onset of the electrocardiogram is used as a reference point, we used the onset of the P wave as the reference point for the calculation of LA ϵ and SR, as previously proposed. We used this point because it most relevantly represents the LA cavity just prior to its contraction. The use of the P wave as the reference point enabled the recognition of peak positive global LA ϵ (ϵ pos peak ), which corresponded to LA conduit function; peak negative global LA ϵ (ϵ neg peak ), which corresponded to LA contractile function; and the sum of these values (total global LA ϵ [ϵ tot ]), which corresponded to LA reservoir function ( Figure 1 ). Similarly, we identified peak negative global LA SR (LA SR late neg peak ) during LA contraction, peak positive global LA SR (LA SR pos peak ) at the beginning of LV systole, and peak negative global LA SR (LA SR early neg peak ) at the beginning of LV diastole.

To calculate LA ϵ and SR, we used 2D speckle-tracking software (EchoPAC; GE Medical Systems), as previously described, using images obtained in apical 4-chamber, 2-chamber, and 3-chamber views. Regarding the 3-chamber view, we included only the inferoposterior wall, as the opposing wall includes the ascending aorta. This software was previously validated for the measurement of LV ϵ and detects and tracks the ultrasonic interference pattern (speckle) inherent to standard 2D echocardiography. This software has been previously used for measuring LA ϵ with high feasibility and good agreement. Briefly, 2D images at one specific cardiac cycle were selected, and the LA endocardial surface was manually traced by a point-and-click approach. An epicardial surface tracing was automatically generated by the system, creating a region of interest, which was manually adjusted to cover the full thickness of the myocardium. Before processing, a cine loop preview was used to confirm if the internal line of the region of interest followed the LA endocardial border throughout the cardiac cycle. The software divided the LA endocardium into 6 segments, resembling the approach used when studying LV ϵ. Segments in which no adequate image quality could be obtained were rejected by the software and excluded from the analysis. Last, the software calculated average ϵ for 6 LA segments for each apical view, and the LA ϵ and SR values for each view were the averages of the values obtained for the LA segments at each view, excluding the 3 LA segments of the anteroseptal wall of the 3-chamber view. The final LA ϵ and SR values were the averages of the values obtained for each apical view.
Statistical Analysis
Calculations were done using commercially available statistical software (GraphPad Prism 3.02 [GraphPad Software Inc, La Jolla, CA] and MedCalc 9.2.0.2 [MedCalc Software, Mariakerke, Belgium]). Continuous variables are expressed as mean ± SD and discrete variables as percentages. All echocardiographic variables passed standard tests of normality (Kolmogorov-Smirnov test), allowing the use of parametric tests. Correlations between variables were tested by simple linear regression analysis (Pearson’s correlation). Data between age subgroups were compared using one-way analysis of variance followed by Student-Newman-Keuls post hoc analysis. Intraobserver and interobserver reproducibility were assessed using Bland-Altman analysis. P values ≤ .05 were considered significant.
Results
Subject Characteristics
Population characteristics are summarized in Table 1 . Subjects presented with normal blood pressures and heart rates. The 2D echocardiographic characteristics are depicted in Table 1 , including chamber dimensions and measurements of LV diastolic and systolic function. All were within normal reference values. LA volumes and indexes of LA function are described in Table 2 .
Variable | Value |
---|---|
Clinical | |
Age (y) | 40.2 ± 13.6 |
Body mass index (kg/m 2 ) | 25.6 ± 4.4 |
Women | 44 (69%) |
Arterial systolic blood pressure (mm Hg) | 118 ± 12 |
Arterial diastolic blood pressure (mm Hg) | 69 ± 8 |
Heart rate (beats/min) | 69.4 ± 9.7 |
Echocardiographic | |
LA diameter (cm) | 3.47 ± 0.45 |
LA area (cm 2 ) | 15.9 ± 3.3 |
LV end-diastolic diameter (cm) | 4.72 ± 0.48 |
LV end-systolic diameter (cm) | 2.86 ± 0.39 |
LV fractional shortening (%) | 39.3 ± 6.6 |
LV end-diastolic volume (mL/m 2 ) | 54.7 ± 11.8 |
LV end-systolic volume (mL/m 2 ) | 14.3 ± 4.6 |
LV ejection fraction (%) | 73.8 ± 6.6 |
LV longitudinal ϵ (%) | −19.8 ± 2.4 |
E (cm/s) | 82.0 ± 20.1 |
A (cm/s) | 58.6 ± 13.7 |
E/A ratio | 1.47 ± 0.50 |
LA filling fraction (%) | 30.8 ± 7.0 |
E-wave deceleration time (ms) | 176 ± 42 |
E′ average (cm/s) | 13.2 ± 2.8 |
E/E′ ratio average | 6.4 ± 1.6 |
A′ average (cm/s) | 9.7 ± 2.1 |
Ar (cm/s) | 28.3 ± 6.3 |
S (cm/s) | 58.9 ± 12.4 |
D (cm/s) | 53.0 ± 10.4 |
S/D ratio | 1.15 ± 0.31 |
Variable | Value |
---|---|
Maximum LA volume index (mL/m 2 ) | 21.9 ± 5.1 |
Minimum LA volume index (mL/m 2 ) | 7.3 ± 5.0 |
Precontraction LA volume index (mL/m 2 ) | 12.1 ± 4.4 |
Total LA stroke volume (mL) | 28.0 ± 7.7 |
Total LA emptying fraction (%) | 70.3 ± 9.2 |
Active LA stroke volume (mL) | 10.6 ± 5.0 |
Active LA emptying fraction (%) | 46.6 ± 11.7 |
Passive LA stroke volume (mL) | 17.5 ± 6.0 |
Passive LA emptying fraction (%) | 44.3 ± 12.1 |
LA expansion index (%) | 271.5 ± 126.4 |
LA ϵ and SR Values and Correlations With Age
Adequate tracking of LA wall was possible in 872 of 930 analyzed segments (93.8%). Global LA ϵ pos peak was 21.4 ± 6.7% and presented significant negative correlations with both age ( Figure 2 ) and body mass index ( r = −0.40, P = .001). Global LA ϵ tot was 35.6 ± 7.9% and presented significant negative correlations with both age ( Figure 2 ) and body mass index ( r = −0.32, P = .01). Global LA ϵ neg peak was −14.2 ± 3.3% and did not present a significant correlation with age ( Figure 2 ) or body mass index. Global LA SR pos peak was 2.0 ± 0.6 s −1 and correlated negatively with body mass index ( r = −0.28, P = .03) but not with age. Global LA SR early neg peak was −2.0 ± 0.6 s −1 and correlated significantly with both age ( r = 0.45, P = .0002) and body mass index ( r = 0.40, P = .001). Global LA SR late neg peak was −2.3 ± 0.5 s −1 and correlated significantly with age ( r = −0.28, P = .02) but not with body mass index.

The interobserver and intraobserver agreement for global LA ϵ and SR were determined after offline reanalysis of recorded clips of 12 randomly selected subjects. The mean differences for intraobserver agreement for LA ϵ were −0.6 % (95% confidence interval [CI], −1.9% to 0.7%), 0.5% (95% CI, −0.3% to 1.2%), and −1.1 % (95% CI, −3.1% to 0.9%) for global LA ϵ pos peak , LA ϵ neg peak , and LA ϵ tot , respectively. The mean differences for interobserver agreement for LA ϵ were 1.8% (95% CI, 0.04% to 3.5%), −0.7% (95% CI, −1.8% to 0.4%), and 2.8% (95% CI, 0.3% to 5.3%) for global LA ϵ pos peak , LA ϵ neg peak , and LA ϵ tot , respectively. The mean differences for intraobserver agreement for LA SR were 0.07 s −1 (95% CI, −0.06 to 0.20 s −1 ), −0.03 (95% CI, −0.24 to 0.18 s −1 ), and −0.06 s −1 (95% CI, −0.14 to 0.02 s −1 ) for global LA SR pos peak , SR late neg peak , and SRearly neg peak , respectively. The mean differences for interobserver agreement for LA SR were 0.18 s −1 (95% CI, 0.09 to 0.28 s −1 ), −0.21 (95% CI, −0.42 to −0.01 s −1 ), and −0.23 s −1 (−0.38 to −0.09 s −1 ) for global LA SR pos peak , SR late neg peak , and SR early neg peak , respectively.
Global LA ϵ pos peak and LA SR pos peak Versus 2D Doppler Echocardiographic Parameters of LA Conduit Function and LV Function
Global LA ϵ pos peak correlated significantly with parameters of LV diastolic function and LA indexes of conduit function. Global LA ϵ pos peak presented positive correlations with E-wave velocity, E/A ratio, VTI E , E′ velocity, and D-wave velocity and a negative correlation with S/D ratio ( Table 3 , Figures 3 A and 3 B). Global LA ϵ pos peak also correlated positively with the passive LA emptying fraction and the LA expansion index and negatively with maximum LA volume index ( Table 3 , Figure 3 C). Global LA ϵ pos peak did not correlate with LV systolic parameters, including LV ϵ. Global LA SR pos peak did not correlate with LV diastolic parameters but correlated negatively with maximum LA volume index and positively with the passive LA emptying fraction ( Table 3 ). Global LA SR pos peak correlated negatively with LV end-systolic diameter ( r = −0.34, P = .006) but did not correlate with other LV systolic parameters.
Global LA ϵ pos peak | Global LA SR pos peak | |||
---|---|---|---|---|
Variable | r | P | r | P |
E | 0.47 | <.0001 | −0.04 | NS |
E/A ratio | 0.54 | <.0001 | −0.09 | NS |
E-wave deceleration time | −0.17 | NS | −0.01 | NS |
VTI E | 0.30 | .02 | 0.03 | NS |
E′ average | 0.55 | <.0001 | 0.07 | NS |
E/E′ ratio | −0.06 | NS | −0.14 | NS |
S | −0.06 | NS | 0.21 | NS |
D | 0.30 | .01 | 0.01 | NS |
S/D ratio | −0.33 | .008 | 0.13 | NS |
Maximum LA volume index | −0.26 | .04 | −0.47 | <.0001 |
Passive LA stroke volume | 0.18 | .14 | −0.11 | NS |
Passive LA emptying fraction | 0.54 | <.0001 | 0.36 | .003 |
LA expansion index | 0.32 | .009 | 0.21 | .09 |

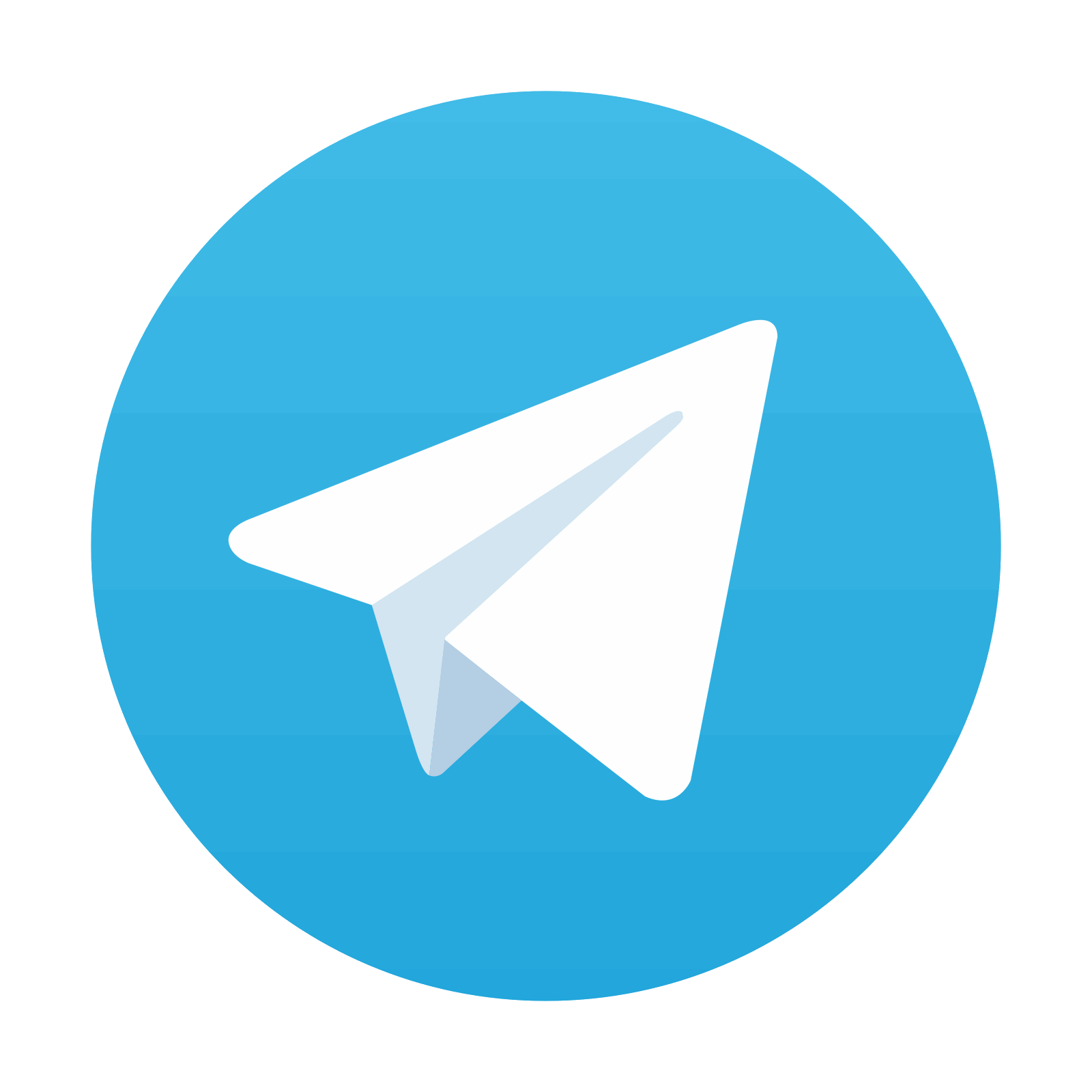
Stay updated, free articles. Join our Telegram channel

Full access? Get Clinical Tree
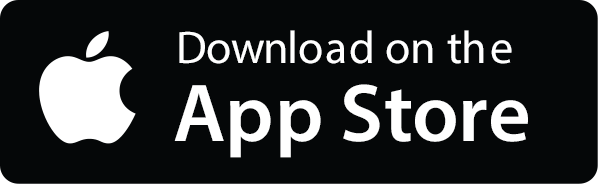
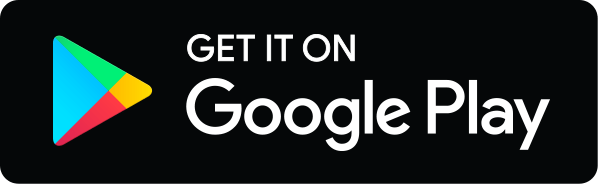
