Fig. 31.1
Trends in immunosuppression after heart transplantation. Modified from the American Journal of Transplantation, 1998–2012, Volume 14, Issue S1, pages 113–38, 28 DEC 2013
Allograft Rejection
Despite advances in IST , rejection continues to be a major challenge in HT recipients, associated with increased morbidity, mortality, and cost. It is estimated that 25 % of HT recipients experience at least one episode of rejection during the first posttransplant year and 5 % of these rejections are severe and associated with hemodynamic compromise [1]. Rejection can be antibody-mediated, including hyperacute rejection and delayed antibody-mediated rejection (AMR), or cell-mediated. The cumulative effect of repeated episodes of rejection, if recovered, ultimately results in graft failure or re-transplantation.
Although allograft rejection is typically a relatively early phenomenon occurring in the first year of transplant, it is becoming increasingly recognized later after transplant, possibly due to improved monitoring surveillance strategies. In the pediatric HT population, 25 % of those who survive the first year experience rejection greater than 1 year after transplant [2]. Late recurrent rejection in adults appeared to be related to the number of episodes of rejection during the first year and history of cytomegalovirus (CMV) infections [3]. The true incidence of late rejection is unclear since definitions have evolved over time and earlier studies described mainly cellular rejection. Nevertheless, late rejection appears to occur infrequently, in 3.5–5 % of surveillance endomyocardial biopsies (EMBs) after during years 2–7 [4]. In a more contemporary study, Loupy reported that very late rejection, defined as occurring >7 years after transplant, was associated with significant microvascular injury, complement deposition, and CAV [5].
Cardiac allograft rejection is often initially asymptomatic until it presents with graft dysfunction. Therefore, early diagnosis relies on screening and surveillance testing. EMB remains the mainstay for the diagnosis of allograft rejection. Most transplant centers perform frequent scheduled EMBs during the first posttransplant year (weekly during the first month, biweekly at 2–3 months, monthly at 3–6 months, and bimonthly at 6–12 months). While most centers continue annual EMBs at 2–5 years posttransplant, the benefit of continuing surveillance EMBs beyond the first year in asymptomatic patients with no prior history of rejection remains unclear [4, 6]. Noninvasive testing is promising and may prove to be a useful first-line screening tool, reducing the need for repeated EMBs . Gene expression profiling test has been shown to have high negative predictive value for acute cellular rejection and could be used to rule out rejection in low-risk patients with no prior history of rejection [7]. Evaluation of donor-specific antibodies may be useful in evaluating for AMR; however, the significance of asymptomatic elevation of DSAs in the absence of graft dysfunction is unclear.
Treatment is warranted for any degree of AMR or acute cellular rejection associated with graft dysfunction. Treatment should be initiated in acute graft dysfunction when rejection is clinically suspected even before histological diagnosis is established. However, it is unclear whether treating asymptomatic AMR is beneficial despite the evidence linking asymptomatic AMR with the development of CAV and with poor outcome [8, 9]. Treatment of asymptomatic AMR is advised in the presence of positive DSA or CAV [10]. Treatment is recommended for moderate and severe asymptomatic cellular rejection. Protocols used for the treatment of allograft rejection vary across transplant centers and will not be discussed here.
Cardiac Allograft Vasculopathy
CAV is perhaps one of the most vexing medical complications facing transplant recipients. This is in large part due to the fact that there are no reliable means to determine which patients develop clinically significant CAV and the lack of effective treatments for the most malignant forms of CAV. CAV is a distinct entity seen in HT recipients and differs from atherosclerotic coronary artery disease in several aspects. CAV is characterized by diffuse intimal proliferation manifesting in lesions that are diffuse and circumferential and involve distal portions of the coronary artery [11, 12]. CAV is very common in HT recipients. It is estimated that 7.1 % of HT survivors at 1 year and 52.7 % of HT survivors at 10 years have CAV and/or late graft failure [13]. CAV and late graft failure are responsible for one third of death of HT recipients after the fifth transplant year [13].
There are multiple theories regarding the etiology and pathophysiology of CAV. The response–to–injury theory proposes that CAV is a result of an ill response to endothelial injury of the transplanted heart caused by both immunologic and non-immunologic insults [14]. Exposure of foreign major histocompatibility antigens of donor endothelial cells to recipient dendritic cells results in T cell activation, triggering a cascade of inflammatory cytokines and growth factor release and activating macrophages and lymphocytes, which in turn secretes various growth factors leading to vascular smooth muscle cell proliferation and intimal hyperplasia seen in CAV [14]. Non-immunologic insults include, but not limited to, ischemia, reperfusion injury, donor age, donor brain death, cytomegalovirus infection, and recipient traditional risk factors such as diabetes, hypertension, and dyslipidemia [15]. Modifications of these non-immunological factors have shown benefit in reducing the incidence and progression of CAV.
Diagnosis of CAV is challenging. Due to allograft denervation, most patients with CAV do not experience angina. Clinically evident acute myocardial infarction rarely occurs, as CAV preferentially affect medium- and small-size vessels. The first clinical manifestation of CAV is often graft dysfunction and graft failure presenting with congestive heart failure, arrhythmia, or sudden cardiac death [15]. Therefore, it is imperative to establish early diagnosis of CAV before it is clinically evident. Coronary angiography, the gold-standard test for the diagnosis of CAV, is routinely performed in transplant recipients (usually at baseline and annually thereafter). The sensitivity of coronary angiography is limited in detecting CAV lesions due to their diffuse, circumferential nature and their involvement of distal and small vessels [16]. The adjunctive use of intravascular ultrasound (IVUS) to measure the maximum intimal thickness (MIT) significantly improves the sensitivity of coronary angiography in detecting CAV during its early stages [17]. Both coronary angiography and IVUS provide significant prognostic information in HT recipients. The absence of angiographically significant lesions on coronary angiogram is a significant predictor of cardiac event-free survival [18]. IVUS findings that predict poor outcome include MIT > 0.5 mm and mean intimal thickness >0.3 mm, and change in MIT by more than 0.5 mm at 1 year is associated with adverse cardiovascular events even with normal coronary angiogram [19–21]. Other adjunctive measures that have been used during coronary angiography include the fractional flow reserve (FFR), the thrombolysis in myocardial infarction frame count (TFC) , and the index of microcirculatory resistance (IMR) . All these measures are shown to predict the development of CAV and to convey poor outcome [22].
Noninvasive tests with various sensitivities and specificities are often used for screening and surveillance of CAV when contraindications to coronary angiogram are present. Dobutamine stress echocardiography and myocardial perfusion imaging can detect obstructive CAV, and its positivity predicts adverse outcome [23, 24]. Cardiac magnetic resonance (CMR) has evolved in the past decade as a promising tool in detecting myocardial ischemia (stress CMR) as well as myocardial scarring (late gadolinium enhancement (LGE) ) and extracellular fibrosis (T1 mapping). LGE in cardiac allograft is frequently seen in patients with CAV and indicates subclinical myocardial infarctions [25]. Studies are ongoing to determine the prognostic values of LGE on CMR of HT recipients. Multi-slice computed tomography (CT) is evolving as a noninvasive imaging modality for evaluating the presence of obstructive CAV. One study of 102 patients reported a negative predictive value for angiographically significant CAV (lesions with >50 % stenosis) of 96.6–99.7 %. However, the positive predictive value was low (<45 %) when compared to invasive coronary angiography [26].
Several blood markers and tissue markers , reflecting endothelial and vascular injury, are elevated in HT recipients with CAV and those at an increased risk of developing CAV [27]. Among these markers are C-reactive protein (CRP), triglycerides to HDL ratio, brain natriuretic peptide (BNP), high-sensitivity troponin T, circulating micro-ribonucleic acid (micro-RNA) particles, von Willebrand factor (VWF), circulating apoptotic endothelial cells and endothelial microparticles, and high gene expression profile (AlloMap) analysis.
The mainstay in managing CAV is primary and secondary prevention. Several interventions targeting risk factors known to be involved in the pathophysiology of CAV have shown to reduce the incidence and progression of CAV. These interventions must be applied early posttransplant as the majority of intimal thickness occurs in the first year. Optimal management of traditional cardiovascular risk factors including diabetes mellitus, hypertension, and dyslipidemia is associated with survival benefit and is recommended by the ISHLT guidelines [28]. The use of hydroxymethylglutaryl-CoA reductase enzyme inhibitors (statins) provides benefits beyond cholesterol lowering, including anti-inflammatory effects, and inhibition of DNA synthesis and, hence, the replication of CMV [29]. Statins have been shown to reduce the incidence of CAV and to improve long-term outcome of HT recipients [30, 31]. Both treatment of established CMV infection and CMV prophylaxis have been shown to improve endothelial function and reduce the progression incidence and progression of CAV [32, 33]. The use of mammalian target of rapamycin (mTOR) inhibitors (both sirolimus and everolimus) has been shown to reduce the incidence of CMV infection, improve endothelial function (as measured by brachial artery flow-mediated dilation), and reduce the progression of CAV [34, 35]. In addition to above, measures to minimize ischemic and operative injury of the allograft have been shown to improve survival and reduce the incidence of CAV [36].
Revascularization for CAV has not shown any mortality benefit and is done largely on a palliative basis [37]. Percutaneous coronary intervention (PCI) is the mainstay of revascularization. In-stent restenosis rate is significantly higher than what is seen in native coronary artery disease, up to 53 % at 1 year and 69 % at 5 years [38]. This is attributed to the diffuse nature of the disease requiring the use of longer and narrower stents as compared with native coronary artery disease (CAD). Contrary to the evidence in native CAD, it is not clear if the use of drug-eluting stents (DES) reduces the incidence of in-stent restenosis or target lesion revascularization [39]. Surgical revascularization is rarely done due to technical challenges (diffuse disease, prior sternotomies) and prohibitively high operative morality (up to 80 %) [40]. Cardiac re-transplantation remains the only definitive therapy for patients with severe CAV resulting in graft failure.
Posttransplant Malignancy
Malignancy is typically a late manifestation after HT occurring in 2.7 % at 1 year and 28.7 % at 10 years posttransplantation [41]. The incidence of new malignancy among solid organ transplant recipients is increased by two- to fourfold, with cancers of the lymphoid system and skin and cancers linked to viral etiology having the highest excess absolute risk [42]. Overall incidence of new malignancy among HT recipients is reported to be 14.3 per 1000 persons/year, with lung cancer being the most common, followed by prostate cancer, posttransplant lymphoproliferative disorder (PTLD), and skin cancer [43]. Malignancy occurs late posttransplant, with mean time to diagnosis of 1267 days. Age older than 39 years, male sex, hepatitis B virus (HBV)-positive recipient status, and non-Hispanic race were associated with increased risk of posttransplant malignancy [43]. Recipients using cyclosporine A (CsA) also have an increased risk of posttransplant malignancy, while switching to or adding an mTOR inhibitor to their immunosuppression regimen reduces the risk of malignancy [44]. The use of statin in HT recipients lowers the incidence of malignancy based on retrospective data of a single center study of 255 patients (cumulative incidence of malignancy over 8 years (34 % vs. 13 %)) [45]. Cancer is a major cause of late mortality in HT recipients surviving the first year posttransplantation. It accounts for 25 % of all deaths at 5 years [46, 47].
Recurrence of pretransplant malignancy after HT is also common. While patients with active cancer are typically excluded from transplantation, there is no clear consensus on the optimal cancer-free waiting period before a patient with prior history of cancer can be listed and may depend on the type of malignancy. A recent study showed that the rate of posttransplant recurrence of pretransplant malignancy was 63 %, 26 %, and 6 % for patients who were cancer-free for less than 12 months, 12–60 months, and more than 60 months, respectively [48].
Skin Cancer
The incidence of all types of skin cancer is drastically increased in HT recipients. Population studies have shown 84-fold, 65-fold, tenfold, and 3.4-fold increase in the incidence of Kaposi sarcoma, squamous cell carcinoma, basal cell carcinoma, and malignant melanoma, respectively, among all solid organ transplant recipients [49]. The higher incidence of skin cancer among transplant recipients is largely attributed to IST, which results in reduced tumor surveillance by the immune system [49, 50]. In addition, individual immunosuppressants contribute directly to the incidence of skin cancer. Calcineurin inhibitors (CNIs), such as cyclosporine and tacrolimus, increase the incidence of squamous cell carcinoma by increasing the levels of growth factors such as transforming growth factor beta (TGF-beta) and vascular endothelial growth factor (VEGF) [44, 51]. Studies have shown that using mTOR inhibitors, such as sirolimus and everolimus, reduces the incidence of SCC in HT recipients [52]. Azathioprine increases photosensitization to UVA, which causes direct DNA damage [53]. Azathioprine is also associated with increase in the incidence of tumor-suppressor gene p53 mutations [54]. The ISHLT guidelines for the care of HT recipients recommend close surveillance, patient education on preventive measures, and annual dermatological examination (level 1C) [28].
PTLD
PTLD is a wide spectrum of lymphoid disorders, ranging from indolent polyclonal lymphoid hyperplasia to aggressive lymphomas [55]. PTLD occurs in up to 5 % of solid organ transplant recipients and in 0.5–1 % of hematopoietic stem cell transplant recipients [56]. Multiple risk factors of PTLD have been identified, including viral infections and recipient-specific characteristics. Epstein-Barr virus (EBV) -seronegative recipients who seroconvert after transplant due to acute exposure or as a result of receiving allograft from an EBV-seropositive donor have sixfold increase in the risk of developing PTLD [57]. Likewise, CMV-seronegative recipients who seroconvert after transplant have sevenfold increase in the risk of developing PTLD [58]. Hepatitis C virus and human herpes virus-8 infection are also associated with increased risk of PTLD, especially in hosts who have EBV infection [58]. The risk of PTLD is also increased in patients with extreme age (<10 and >60 years), patients with history of pretransplant malignancy, and patients with various genetic variations such as polymorphisms in certain cytokine genes, including interleukin-10 (IL-10), interleukin-6 (IL-6), and interferon-gamma (IFN-γ), and polymorphism in certain donor and recipient human leukocyte antigens (HLAs) [59, 60]. More than 90 % of PTLDs are B cell type. Two thirds of B cell PTLDs are EBV-related, while only 10–15 % of T cell PTLDs are EBV-related. EBV-negative PTLDs tend to be monomorphic, diagnosed late, and less responsive to therapy [55].
Clinical manifestation of PTLD is often nonspecific. In addition to the classic B symptoms, lymphadenopathy can be present in nodal disease, while symptoms related to the organ involved are present in extra-nodal disease. Extra-nodal disease often involves the gastrointestinal tract, lungs, skin, bone marrow, and central nervous system [61].
Reduction in immunosuppression (RI) has remained the first-line therapy for PTLD for the past four decades [62]. However, response to reduction in immunosuppression alone varies, ranging between 0 and 73 %, and sustained response is only seen in 10–20 % of cases [63, 64]. Older age, late posttransplant onset of disease, the presence of B symptoms, multi-organ involvement, and EBV seronegativity are predictors of poor response to RI. Furthermore, 40 % of patients treated with RI alone developed acute graft rejection [60]. Therefore, adjunct therapy is often used in addition to RI, including rituximab, chemotherapy, radiation therapy, antiviral therapy, and immunotherapy [56]. Other therapies have also been tried, including IL-6 and IL-10 monoclonal antibodies and autologous stem cell transplant [65, 66]. Since treatment of PTLD differs from that of de novo lymphomas, the ISHLT guidelines recommend referral to a transplant center with expertise in posttransplant malignancies for the initial evaluation and therapeutic plan of patients with new diagnosis of PTLD .
Posttransplant Infection
Infection is a leading cause of death after HT, second to graft failure [67]. Although the incidence of death due to infection peaks during the first transplant year, afterward, infection accounts for approximately 10 % of deaths up to 15 years after transplant. Much attention is given to viral infections, particularly cytomegalovirus (CMV) and Epstein-Barr virus (EBV), due to the association with CAV and PTLD; however, bacterial infections remain more common than viral infections in solid organ transplant recipients [68]. In a series of 620 HT recipients, bacterial infections caused 43.6 % of infectious episodes, followed by viruses, fungus, Pneumocystis carinii, and protozoa. The most common site of infection was the respiratory tract [68]. Prophylaxis is routinely performed for opportunistic infections.
CMV is a major cause of morbidity and mortality in solid organ transplant. The highest risk of infection occurs when a CMV-seronegative recipient receives an organ from a seropositive donor. CMV may cause a wide spectrum of morbidity including bone marrow suppression and cytopenias, pneumonia, hepatitis, and nephritis, but perhaps most commonly seen is CMV gastrointestinal disease [69]. Furthermore, CMV may contribute to graft failure by its association with CAV [15]. Cytomegalovirus infection is associated with cardiac allograft rejection and atherosclerosis [70]. CMV promotes a pro-inflammatory environment and causes dysregulation of the NO synthase pathway. CMV typically occurs during the first 3 months if prophylaxis is not given. Both valganciclovir and oral ganciclovir have been shown to be efficacious in preventing CMV infection in high-risk seronegative recipients of organs from seropositive donors [71]. Subclinical CMV antigenemia may increase the risk of allograft rejection and CAV, and providing CMV prophylaxis has been shown to reduce the risk of both entities [33, 72].
Adverse Effects of Immunosuppressive Drugs and Comorbid Conditions After Transplant
There are four major classes of immunosuppressive drugs that are routinely used in IST: glucocorticosteroids (GCS) ; calcineurin inhibitors (CNIs), including cyclosporine A (CsA) and tacrolimus; antiproliferative drugs, including azathioprine and mycophenolate mofetil (MMF); and mammalian target of rapamycin (mTOR) inhibitors, including sirolimus and everolimus. Over the past 15 years, there has been significant change in the trend of use of these drugs worldwide (◘ Fig. 31.2). These drugs are used in combination according to standardized IST regimens, which vary across transplant centers, and often modified according to patient-specific circumstances.


Fig. 31.2
Relative incidence of leading causes of death for adult heart transplant recipients, January 2009–June 2014. International Society for Heart and Lung Transplantation Registry. Since only leading causes of death are shown, the sum of percentages for each time period is less than 100 %. CAV cardiac allograft vasculopathy, CMV cytomegalovirus, PTLD posttransplant lymphoproliferative disorder. Modified from JHLT. 2015; 34(10): 1244–54
GCS were used indefinitely in older immunosuppression regimens consisting of CsA, azathioprine, and GCS. However, many centers now discontinue GCS within 6 months after transplantation in the absence of rejection. CNIs remain the backbone of most IST regimens. Tacrolimus-based regimens may be associated with lower rejection rates than a CsA-based regimen, with no difference in overall survival, although tacrolimus is associated with greater incidence of diabetes and hyperlipidemia [73, 74]. MMF has largely replaced azathioprine as first-line antiproliferative drug due to its superiority in allowing GCS taper and its association with better survival and fewer rejection episodes [75]. Immunosuppression regimens containing mTOR inhibitors have not shown any survival or antirejection benefit over MMF-containing regimens. However, mTOR inhibitors inhibit endothelial cell proliferations and have been shown to reduce progression of CAV [34, 35]. In addition, patients treated with mTOR inhibitors are shown to have lower incidence of CMV infections and other opportunistic infections [76].
Renal Dysfunction
The prevalence of renal dysfunction drastically increases after HT. The prevalence of chronic kidney disease (CKD) stages 4 and 5 in HT recipients increases from 3 % at the time of transplant to 11 % at 1 year and to 15 % at 6 years posttransplantation [77]. According to data from the ISHLT registry, renal dysfunction is present in 36.7 % of HT recipients at 1 year and 68.2 % at 10 years posttransplant. By posttransplant year 10, 6.1 % of HT recipients are on chronic dialysis and 3.6 % have had a kidney transplant [78]. Mortality risk is significantly higher in HT recipients with renal dysfunction (hazard ratio 1.66 for CKD stage 4 and 4.07 for CKD stage 5 on dialysis) [78]. The incidence and progression of renal dysfunction are higher in HT recipients receiving CNI (both CsA and tacrolimus) for immunosuppression and correlate with the dose of CNI used [79]. Recent biopsy-based study revealed that the etiology of renal dysfunction in the majority of HT recipients is hypertensive and diabetic nephropathy rather than merely the effect of CNIs, although the latter is shown to accelerate the progression of hypertensive and diabetic nephropathy [80]. This underscores the importance of optimal management of underlying hypertension and diabetes in transplant recipients with renal dysfunction in addition to minimizing exposure to CNI.
CNI-mediated nephrotoxicity is thought to be the end result of CNI-mediated altered glomerular hemodynamics and tubular function and CNI-mediated direct tubular injury [81]. Altered glomerular hemodynamics result from vasoconstriction of the afferent arterioles caused by decrease in nitric oxide and prostaglandin E2 and increase in thromboxane, endothelin, and the renin-angiotensin system [82]. CNI use may also alter tubular function by causing electrolyte disturbances such as hypomagnesemia, hyperkalemia, hyperuricemia, and hyperchloremic metabolic acidosis [83, 84]. Direct CNI toxicity is characterized by arteriolar hyalinosis and nodular hyaline deposits in the media of afferent arterioles, resulting in arteriolar narrowing and leading to the development of interstitial fibrosis, tubular atrophy, and glomerular sclerosis. Interstitial fibrosis and glomerulosclerosis can also result from activation of the renin-angiotensin- aldosterone (RAS) system and upregulation of TGF-ℬ, leading to irreversible renal injury [85].
The use of mTOR inhibitor has allowed for CNI-eliminating or CNI-minimizing immunosuppression regimens that are often adopted in HT recipients who develop renal dysfunction on full-dose CNIs. Significant improvement in renal function with both CNI elimination (conversion from full-dose tacrolimus plus MMF to everolimus plus MMF for HT recipients >1 year posttransplant) and CNI minimization (conversion from full-dose tacrolimus plus MMF to reduced-dose tacrolimus plus everolimus for HT recipients <1 year posttransplant) has been demonstrated [86]. Conversion from tacrolimus or CsA to the mTOR inhibitor, sirolimus, in HT recipients with mild to moderate renal dysfunction (GFR 40–90 ml/min/1.73 m2) is associated with significant improvement in GFR at 1 year, however, with the trade-off of more rejection and discontinuation of sirloins due to side effects [87]. While CNI-minimizing regimens are beneficial in patients with established renal dysfunction, using these regimens in HT recipients with normal renal function did not lower the incidence of CNI-induced renal dysfunction [88].
Neurological Adverse Effects
Approximately one third of solid organ transplant recipients present with neurologic symptoms related to a wide spectrum of pathology including CNS infections, tumors, encephalopathy, stroke, and peripheral neuropathy [89]. These conditions are often related to immunosuppression in general or adverse effect of the specific immunosuppressive medications.
Neurotoxic adverse effects are experienced by 10–28 % of patients receiving CNI and are more severe with tacrolimus than with CsA [90]. Symptoms include tremor, insomnia, nightmares, headaches, vertigo, dysesthesia, photophobia, mood disturbances, akinetic mutism, seizures, cortical blindness, focal neurological deficits, psychosis, and encephalopathy. Treatment often includes switching from tacrolimus to CsA or vice versa, decreasing the dose of CNI by switching to an everolimus-based regimen or, when feasible, eliminating CNI, especially when symptoms are severe and risk of rejection is low.
Other immunosuppressive drugs are also associated with neurological complications. Progressive multifocal leukoencephalopathy has been reported in patients receiving MMF and attributed to the activation of the JC virus [91]. Aseptic meningitis is seen in 5–10 % of patients receiving monoclonal antibodies (such as OKT3 and daclizumab) and polyclonal antibodies (such as antithymocyte globulin (ATG)) for induction therapy and rescue therapy of rejection. This is attributed to the rapid release of pro-inflammatory cytokines, lymphocyte activation, and cell lysis [92, 93]. The use of GCS is often associated with behavioral changes such as confusion, mood disturbance, and manic states and psychosis, especially at higher doses. These effects usually resolve after lowering the dose or discontinuing GCS when feasible.
Non-immunosuppressive drugs often used in HT recipients can also cause neurological complications, either directly or as a result of their interaction with immunosuppressive drugs. For instance, antifungal medications, macrolide antibiotics, and calcium channel blockers interfere with the metabolisms of CNIs affecting their blood level and may precipitate neurological adverse effects.
Finally, neurological symptoms in HT recipients can be manifestations of an underlying central nervous system opportunistic infection or malignancy, especially PTLD . Vigilance must be applied to evaluate for these conditions before attributing symptoms to drugs adverse effects.
Hypertension
Hypertension is common among HT recipients. According to the ISHLT registry 2014 adult HT report, 71.8 % of recipients have hypertension at 1 year and 91.7 % at 5 years posttransplant, largely attributed to aging and the use of IST , especially CNI [67]. The predominant theory for the development of CNI-related hypertension is via dysregulation of renal and systemic vascular tone, either due to increased vasoconstriction or decreased vasodilatation. Tacrolimus may be associated with less systemic hypertension than CsA due to a lesser effect on systemic vascular resistance and is associated with improved cardiovascular risk profile [94, 95]. Treatment goals for HTN after HT are the same as those in the general population. Calcium channel blockers have commonly been used. Diltiazem and verapamil may increase levels of cyclosporine.
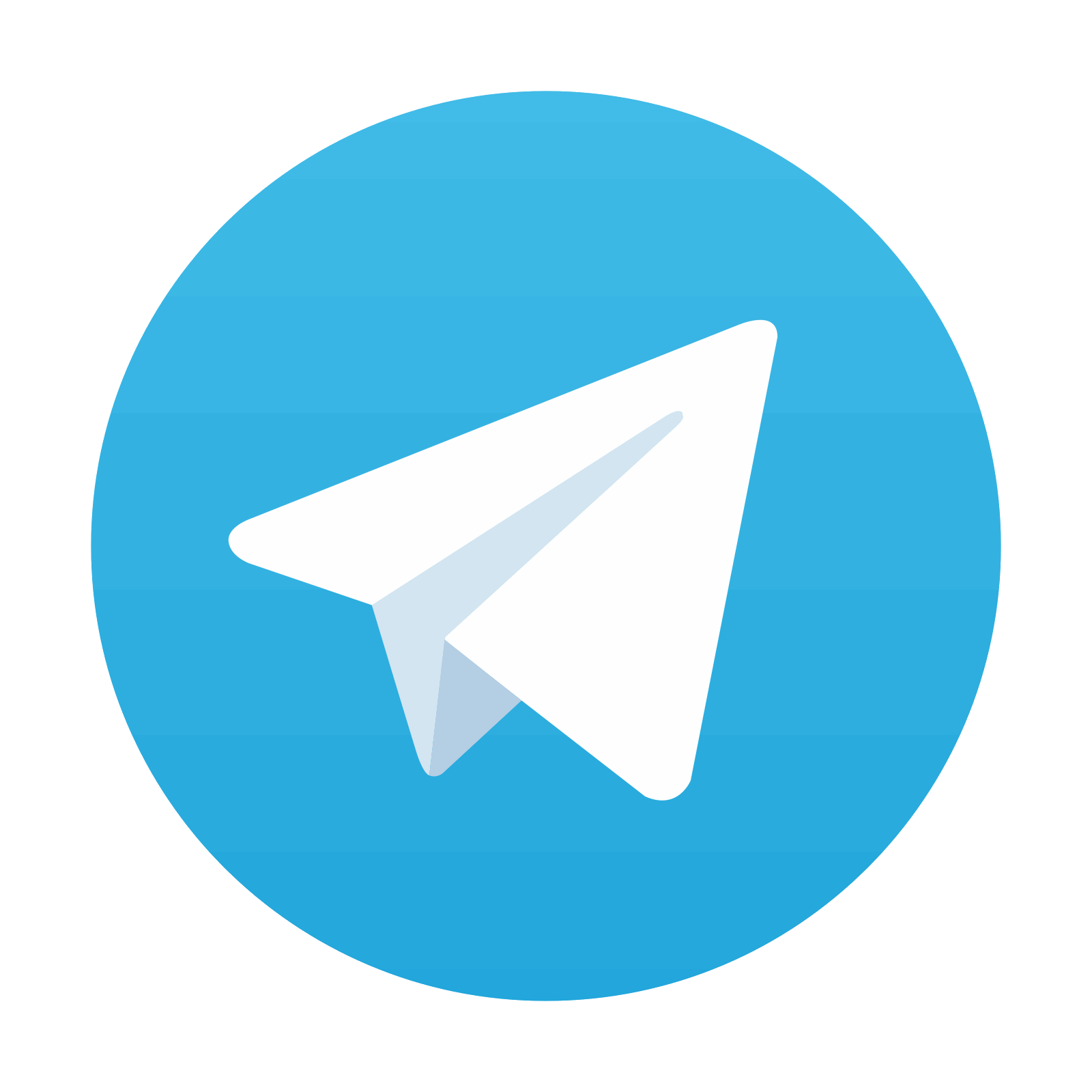
Stay updated, free articles. Join our Telegram channel

Full access? Get Clinical Tree
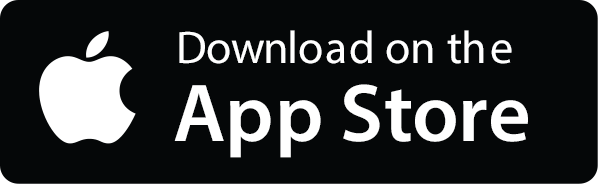
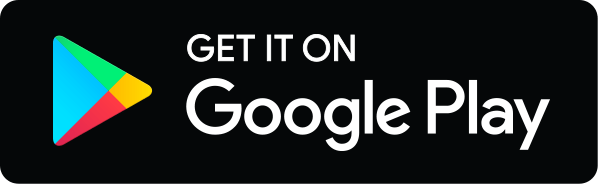