Veins amenable to EVLA
Great saphenous vein
Small saphenous vein
Anterior accessory saphenous vein
Anterior thigh circumflex
Posterior thigh circumflex
Intersaphenous vein
Perforators
Indications favoring EVLA
Large diameter veins (>10 mm)
Increased vein tortuosity
Recanalized veins following prior treatment
Recanalized veins following superficial vein thrombosis
With regard to the theoretical advantages to the utilization of the EVLA modality specifically, it may be particularly useful for the treatment of larger refluxing veins, as well as tortuous refluxing veins, such as the anterior thigh circumflex, or veins that have recanalized, whereby the intraluminal lesions may increase the complexity of traversing the entire vein. Much of the versatility of EVLA may be attributed to the fact that EVLA utilizes a low-profile device in an over-the-wire procedure that may also be guided by ultrasound. Consequently, the ability to routinely traverse the vein first with the wire prior to advancing with the sheath/catheter system offers a distinct advantage as compared to radiofrequency ablation, for example. However, both modalities are indicated for all vein types, and the practice patterns and indications for these procedures are ultimately left to the discretion of the operator.
EVLA Procedure
As stated previously, success of the procedure is predicated on adequate patient preparation. To maintain maximum distension of the refluxing truncal vein, the patient should be well hydrated, warm, and relaxed. Certain environmental manipulations like keeping a warm procedure room, using warmed ultrasound gel, and administering a single dose of a relaxing agent such as a benzodiazepine (e.g., alprazolam) may help to mitigate against venospasm. The area of treatment may also be mapped with a surgical marking pen. The latter helps especially during the early phase of the learning curve when performing ultrasound-based procedures.
The nuances of treatment vary slightly depending on the target vein; however, the principles remain the same. The procedure begins with obtaining access. Local anesthetic may be administered, and using a micropuncture set with a 21-gauge needle, the great saphenous vein (GSV) or small saphenous vein (SSV) , for example, may be accessed. With regard to the GSV, this is performed at or slightly below the knee area and typically central to where the vein becomes epifascial [6]. This prevents thermal skin injury and by not venturing too peripherally down the calf, this reduces the risk of nerve injury resulting in paresthesias in the saphenous nerve distribution. Similar precautions should be taken with treatment of the SSV and the potential injury that may occur to the sural nerve [9, 10]. Following intraluminal confirmation, the inner dilator and microwire are withdrawn, and a 0.035 inch wire is advanced to the saphenofemoral (SFJ) or saphenopopliteal junction (SPJ) . The sheath is advanced over the wire to a point of 2 cm to greater than 2.5 cm from the respective deep venous junction [11]. After confirming and recording appropriate positioning using ultrasound, the dilator and wire are withdrawn, and the laser fiber is advanced to the same point. At this point, the laser fiber is connected to the generator , and the aiming beam is used to help localize the fiber’s location subcutaneously. The sheath is withdrawn over the laser fiber and confirmed to remain at 2 cm or greater than 2.5 cm peripheral to the respective deep venous junction. The sheath and the laser fiber are connected using a Luer-lock mechanism. Given that EVLA is a form of endothermal ablation, tumescent anesthesia is administered perivenously with the goal of creating a diameter of fluid around the vein of approximately 10 mm [12, 13]. This helps to reduce the risks of thermal injury to the adjacent tissues resulting in skin burns, pigmentation, paresthesias, etc. Additional procedural modifications exist that may slightly alter the technical aspects of the procedure. Examples of procedural modifications include the utilization of a 0.018 inch system in order to eliminate the use of the original micropuncture catheter or the use of a short sheath while advancing the laser fiber to position in a “bare-back” fashion.
Once in position, the EVLA procedure may be performed. Following confirmation of laser positioning, the laser is switched to the ready mode, and the foot pedal is engaged. The laser fiber and sheath are withdrawn simultaneously, and the vein is treated up to 1–3 cm from the access site in order to minimize the risk for thermal injury to the skin. The supporting data is presented later in the chapter, but the average LEED that is utilized should range between 60–100 J/cm and 30–50 J/cm depending on the technology being used in order to maximize treatment efficacy while minimizing the procedural complications as they relate to thermal injury, such as pain bruising [6, 14]. Compression is not applied during the treatment in order to minimize post-procedural pain and bruising. Ultrasound is used to confirm successful ablation of the treated vein and the absence of an associated deep vein thrombosis.
Procedural Variables
There are many variables that can be adjusted in order to effect the desired outcome. Some procedural technique variables that can be manipulated include the pullback time, the power setting, and the linear endovenous energy density (LEED). Moreover, the laser catheter itself comes in varied iterations of fiber type and wavelength.
Laser Wavelength
The use of different laser wavelengths has evolved over time. The progression of technology has moved steadily along the wavelength spectrum. The initial lasers comprised the lower end of the wavelength spectrum (810, 940, 980, and 1064 nm), represented by the hemoglobin-specific laser wavelengths (HSLWs) [15] (Fig. 8.1). The name is derived from the target chromophore, which is hemoglobin. In vitro studies have demonstrated that as the hemoglobin within the red blood cell absorbs the energy from the laser, this results in a combination of heat and steam bubble formation [7]. Endothelial destruction ensues resulting in a thrombotic occlusion.


Fig. 8.1
Graph delineating laser wavelength (nm) versus absorption (Modified from http://venacure-evlt.com/endovenous-laser-vein-treatment/angiodynamics/products/laser/venacure-1470nm-laser/)
Of note, the mechanism of action does not require direct contact between laser fiber and the vein wall. With regard to the treatment itself, this has implications. Initially, the thought was that vein wall contact was necessary in order to effect a technically successful ablation. Therefore, direct pressure was applied during the procedure traditionally, and this resulted in increased pain and bruising. In vitro studies identified a direct correlation between fiber/vein wall contact, vein wall perforation, and pain and bruising [7]. As a result compression is no longer a part of the standard procedure. Moreover, pulsed energy lasers have fallen out of favor for similar reasons , given that the bursts of increased thermal injury may have resulted in increased vein wall perforations , manifested by increased pain and bruising. Therefore, continuous energy lasers are now used predominantly [12, 16, 17].
Progressing further with laser technology, longer wavelength lasers were found to have a greater affinity for water as the chromophore (1320, 1470, and 1510 nm) (Fig. 8.1). These are known as the water-specific laser wavelengths (WSLWs) . Water, as compared to hemoglobin, functions more efficiently as a chromophore resulting in a 40-fold improvement in energy absorption when the comparison is made. The mechanism of action is thought to be more selective as compared to the HSLW, because water within the vein wall is targeted directly, resulting in direct damage to the intima [8]. Consequently, lower power settings are required in order to achieve the same effective LEED [6, 18, 19].
The data supporting the varying laser wavelengths have evolved over time, and the general progression has been from the lower wavelengths (HSLWs) to the higher wavelengths (WSLWs). As an example, one of the original randomized studies compared the 810 and 980 nm lasers, both HSLWs, using the same LEED. Fifty-one patients underwent treatment, and technical success for the treatment did not differ between the two groups (one treatment failure per group); however, pain and bruising were less in the 980 nm group [6]. Continuing with the progression, longer wavelength lasers underwent pairwise comparisons. Proebstle et al. compared the 940 nm HSLW laser to the 1320 nm WSLW laser. Care was taken to maintain the same LEED across the comparison groups, approximately 60 J/cm for the 940 nm group (power = 30 W) and 60 J/cm for the 1320 nm group (power 8 W) [20]. Corroborating the increased specificity for the vein wall when using the WSLW laser, the amount of pain and bruising was less in the 1320 nm group while maintaining the same level of treatment efficacy. This relationship held true even when the same patient underwent treatment in one leg with the 810 nm HSLW laser while undergoing treatment in the other leg with the 1320 nm WSLW laser [15]. Use of the 1470 nm WSLW laser has also contributed to the trend of maintaining treatment efficacy while further decreasing post-procedural symptomatology. Shutze et al. evaluated the treatment of 1439 veins, where the 1470 nm laser was used in 295 procedures and the 810 nm laser was used in 1144 procedures [21]. Pain and bruising as well as quality of life scores were improved in the 1470 nm cohort. Moreover, the incidence of endothermal heat-induced thrombosis (EHIT) was diminished in the 1470 nm group as compared to the 810 nm group (2.4 vs 6.0%, P = 0.0122). In a three-way comparison of the 810, 980, and 1470 nm fibers, the pain and bruising scores improved progressively with each successive increase in wavelength while maintaining equal efficacy [22]. An in vitro adjunct was performed as part of the same study, and thermal injury depths were found to be less in the 1470 nm laser as compared to the 810 nm laser, which is consistent to the results that are identified clinically.
Fiber Tip
In addition to research being applied to laser wavelength in order to improve the specificity of treatment to the vein wall, mechanical factors have also been manipulated in order to enhance the specificity of treatment to the vein wall. This has been best exemplified by the modifications to the fiber tip. The original laser fibers were bare-tip fibers. Along with the original procedural methodology to apply manual compression to the site of treatment, the factors worked in concert to promote contact between the fiber tip and the vein wall. This resulted in an increased incidence of vein wall perforation and increased post-procedural pain and bruising [7]. Consequently, jacket-tip fibers were developed, and they come in various iterations, including ceramic and metallic types. The jacket-tip functions to act as a mechanical barrier preventing direct contact between the laser fiber and the vein wall, and some jacket-tip fibers are configured to reduce the energy density supplied by diverting and dispersing the laser energy. As a physical barrier, the metallic jacket tip results in a 0.010 inch physical barrier between the laser fiber and the vein wall. Moreover, the configuration of the jacket tip results in a 15° divergence of the emitted laser light, increasing the effective diameter of emitted energy from 600 to 905 μm [23] (Fig. 8.2). With regard to dispersing the laser-emitted energy and taking this concept to its fullest extent, a radial-emitting fiber was created that results in 360° dispersion by deflecting the laser energy orthogonally out of the side of the catheter [24].


Fig. 8.2
Jacket-tip fiber as illustrated by the NeverTouch gold-tip laser fiber which results in an increased effective diameter of 905 μm as compared to the 600 μm of the standard bare-tip laser fiber (Reference: http://venacure-evlt.com/endovenous-laser-vein-treatment/angiodynamics/products/kits/nevertouch-procedure-kit/)
The theoretical advantages behind the evolution in fiber-tip design have translated to improvements in clinical outcomes. One of the initial studies to evaluate fiber-tip design in a randomized fashion compared patients treated using a 980 nm laser using either a bare-tip or a jacket-tip fiber [23]. The LEED was normalized to 100 J/cm in both groups, and technical success was achieved in all treated patients. The pain scores improved significantly in the jacket-tip group as compared to the bare-tip group. The relationship was further evaluated using the 1470 nm WSLW laser. Three different kinds of fiber tips were compared, including a bare tip, jacket tip, and radial-emitting tip [24]. Once again, treatment efficacy was found to be equivalent between all groups. Pain scores improved successively when transitioning from the bare tip to the jacket tip and then to the radial-emitting tip.
More recently, investigators have attempted to tease out the variety of wavelength/fiber-tip combinations and their relative importance in affecting procedural outcomes [22]. The study was a combination of an in vitro as well as a clinical analysis comparing the 810, 980, and 1470 nm fibers using bare-tip or jacket-tip fibers . As alluded to previously, the pain and bruising scores improved in direct correlation with increasing wavelength, and this was corroborated by the in vitro data. When evaluating bare-tip versus jacket-tip fibers, the depth of thermal injury was worse in the bare-tip group as compared to the jacket-tip group in the 810 nm group (1.05 mm ± 0.34 mm vs 0.36 mm ± 0.26 mm; P < 0.0005) and in the 1470 nm group (0.71 mm ± 0.31 mm vs 0.20 mm ± 0.16 mm; P < 0.0005). Moreover, a multivariate analysis was performed to compare all variables. Both the in vitro data and the clinical data demonstrated that the fiber type was a more dominant variable, as compared to the laser wavelength, in improving outcomes. The results were additive, with the best outcomes obtained in the 1470 nm laser with the use of a jacket-tip fiber [22].
Technique Variables
Once the procedural equipment is in place, there are myriad procedural variables that can be manipulated to alter safety and efficacy outcomes. Moreover, the variables are often dependent on the type of laser being used, and this will be delineated further below. Briefly, the procedural variations are related to the pullback time and to the power settings, both of which interact to produce the linear endovenous energy density (LEED) .
Initial modes of treatment were performed using a pulse mode, and over time this has evolved to a continuous mode due to decreased pain and bruising [12]. With further collective experience, the pullback rate increased from 3 mm/s to 1 cm every 3–5 s. The pullback rates in general would equate to a range of 12–20 cm/min. Furthermore, the accuracy and reproducibility of the pullback rate have improved over time; the original sheaths did not exhibit markers as compared to the newer sheaths that are available today, which exhibit distance markers. With regard to the technique , this has implications because the user performs a continuous pullback gauging the 1 cm markers on the catheter relative to the amount of energy used, and this has been more accurate than gauging the timing of the pullback. This technique is also less dependent on the power setting, which can vary significantly based on the laser wavelength being used.
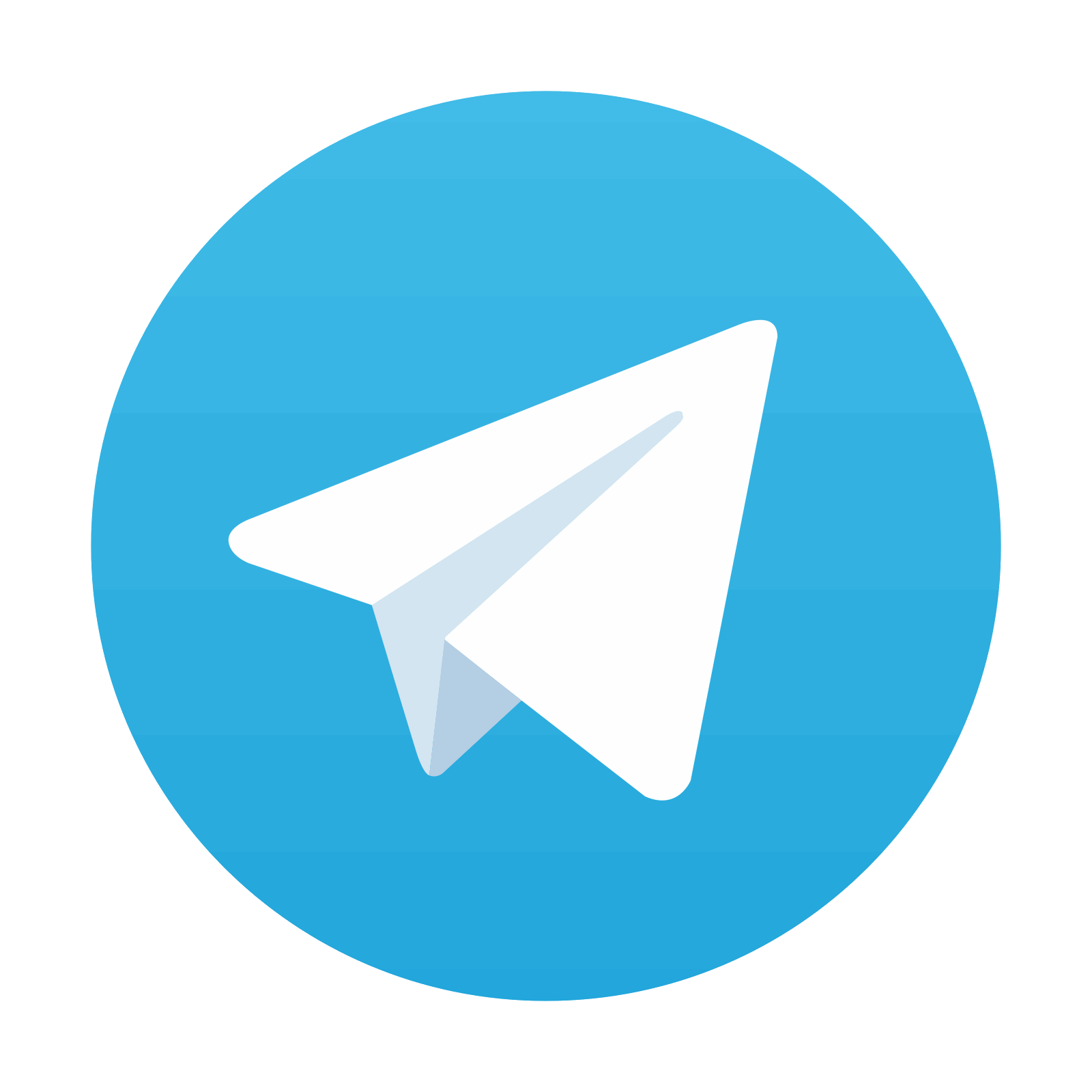
Stay updated, free articles. Join our Telegram channel

Full access? Get Clinical Tree
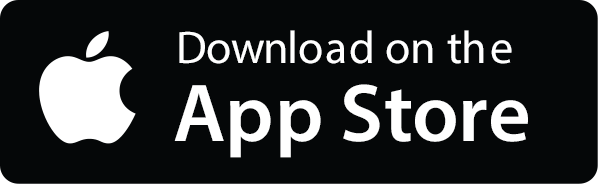
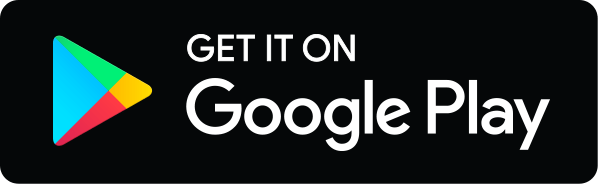