Background
Right ventricular volume quantification using real-time three-dimensional echocardiographic (RT3DE) imaging is limited by technical shortcomings of acquisition and quantification. In this study, a two-step approach was used to overcome these limitations. First, a modified acquisition technique for RT3DE imaging was applied, and second, a software tool using knowledge-based reconstruction (KBR) was used. The approach was validated against the gold standard, cardiac magnetic resonance (CMR) imaging, using CMR and RT3DE data sets from healthy children and from patients with congenital heart disease.
Methods
Sixty individuals (20 healthy persons, 40 with congenital heart defects; age range, 2.3–43.9 years; median age, 11.3 years) consecutively underwent investigation by CMR and RT3DE imaging. CMR data sets were first quantified by the method of disks (MOD) as the standard. Then CMR and RT3DE data sets were quantified using KBR software and compared with the MOD.
Results
CMR was more feasible than echocardiography (100% vs 88%). Compared with the MOD (CMR MOD ), there were trivial volume overestimations of KBR for CMR data (CMR KBR ), of end-diastolic volume (EDV) (−1.3 ± 8.6%, r = 0.984) and end-systolic volume (ESV) (−3.4 ± 13.3%, r = 0.985), resulting in a 0.7 ± 8.7% difference in ejection fraction (EF) ( r = 0.882). Comparing CMR MOD and RT3DE imaging, EDV (1.1 ± 7.4%, r = 0.990) and EF (0.8 ± 9.2%, r = 0.871) were slightly underestimated by RT3DE imaging, with a slight overestimation of ESV (−1.5 ± 13.3%, r = 0.977). Intraobserver variability was excellent for KBR of CMR and RT3DE data, with interclass coefficients of correlation of 0.995 and 0.997 for EDV, 0.995 and 0.994 for ESV, and 0.915 and 0.912 for EF. Interobserver variability provided intraclass correlation coefficients of 0.992 and 0.990 for EDV, 0.997 and 0.992 for ESV, and 0.953 and 0.933 for EF. The KBR analysis required a mean time of 5 min.
Conclusions
KBR is an accurate, versatile, and time-saving method for right ventricular three-dimensional volumetry; it shows excellent reproducibility for RT3DE and CMR data sets. These results suggest that this tool is clinically valuable.
Assessment of right ventricular (RV) function is essential in patients with congenital heart disease (CHD) because RV volume load, dysfunction, and eventually failure are important issues in this population. In patients with corrected tetralogy of Fallot (TOF) and patients with transposition of the great arteries (TGA) after palliative surgery, progressive RV dilatation, hypertrophy, and the development of arrhythmias are significant clinical problems leading to high morbidity figures, including heart failure in young patients. The prevalence of RV dysfunction is high, even in asymptomatic individuals with systemic right ventricles.
A recent consensus panel report states that cardiac magnetic resonance (CMR) imaging is the gold standard for the assessment of RV volumes and function. Reasons include the method’s high accuracy, reproducibility, and independence from geometric assumptions. However, CMR cannot be used routinely in all clinical settings: it is expensive and time-consuming, it may require anesthesia, and it may even be contraindicated in some patients. In comparison, echocardiography is quick, inexpensive, generally available, and does not in most cases require sedation, not even in small children.
The complex crescent shape of the right ventricle is challenging for echocardiographic assessment because it cannot be properly visualized in single two-dimensional (2D) views. Therefore, multiple acoustic windows must be used. Furthermore, standard 2D echocardiography (2DE) has been shown to be inaccurate for the estimation of RV size and function. Real-time three-dimensional (3D) echocardiographic (RT3DE) imaging has emerged as a promising clinical alternative. Body constitution, the individual cardiac axis, and the limitations of maximal full-volume angles make it challenging to depict the entire RV cavity for accurate volume estimation. This is especially true for enlarged ventricles. Furthermore, the underlying strategy of the particular quantification technique is important. In general, manual tracing techniques seem to be more accurate than semiautomated border detection algorithms, but they are more time-consuming. Recent studies of RV volumetry by RT3DE imaging in comparison with CMR suggest that RT3DE imaging underestimates RV volumes and ejection fraction (EF), particularly in more dilated ventricles. In contrast, older patient age was associated with overestimation of volumes and underestimation of EF.
Recently, a new technique has become available for reconstructing the RV surface. It uses a knowledge-based reconstruction (KBR) database, which can identify various shapes of healthy and diseased right ventricles. This technique has already shown promising results calculating RV volumes from CMR and 2D echocardiographic data sets.
Study Aims
We evaluated a new software tool designed to process CMR and RT3DE data sets. The hypothesis of this study was that KBR would provide accurate and reproducible RV volumes and EFs compared with a CMR reference standard based on the method of disks (MOD). The analysis included an evaluation of the differences between the volumetric results with RT3DE versus CMR imaging, an assessment of the method’s reproducibility, and an analysis of whether KBR can be used for right ventricles with different volumes and pathologies with a specific focus on left ventricular (LV)–to–RV interactions and CHD.
Methods
We prospectively enrolled 40 patients with different cardiac defects and RV pathologies. All patients had been referred to our CMR department for diagnostic clinical examination. The majority of investigations were performed in awake and cooperative individuals. In seven younger children unable to hold their breath, CMR and RT3DE imaging were carried out one after the other under anesthesia. Echocardiography was performed in the same laboratory immediately after the CMR examination. To account for RV shapes varying with different diseases, the KBR software provides different references for RV reconstruction in specific malformations. Twenty patients were suitable for the reference “postoperative TOF,” and five patients with dilated right ventricles due to left-to-right shunts were investigated with the tool “pulmonary hypertension” as recommended by the manufacturer. Ten patients had pathologic LV-to-RV interactions due to LV disease, resulting in abnormal LV shapes. This group included patients with cardiomyopathies as well as those with aortic valve disease and impaired LV function. In these patients, we used the “right ventricle” tool for normal right ventricles. Five patients who had undergone Mustard procedures were assessed with the “d-TGA – atrial switch” reference. Additionally, a group of 20 healthy children and young adults was recruited. Their ventricles were assessed with the tool “right ventricle” for right ventricles with normal shape. In addition to a good ultrasound window, we used a modified RV apical view for RT3DE imaging. Mandatory for the inclusion of these healthy individuals were normal results on standard echocardiography as well as sinus rhythm. The study was approved by the local institutional ethics review committee (local registration no. 20/2011) and conforms to the principles of the Declaration of Helsinki and to German law. Informed written consent was obtained from all participants and/or their legal guardians before participation.
RT3DE Imaging
A single sonographer with 7 years of experience conducted all RT3DE studies with an iE33 system with an X5-1 transducer (Philips Medical Systems, Best, The Netherlands) or a Vivid E9 with a V4 transducer (GE Medical Systems, Milwaukee, WI). Starting from the position of a standard five-chamber view, the probe was angulated upward and toward the left until inflow, outflow, and apex of the right ventricle became visible. This view resembles a short-axis view to a certain extent but also includes the entire apical portion of the right ventricle. RT3DE volumes were acquired from an apical view with the RV apex next to the transducer and also depicting the complete inflow and outflow portions. On the basis of this 2D planning view ( Figure 1 ), one full-volume data set was acquired including four 90° to 110° subvolumes under a single end-expiratory breath-hold with stable transducer position. Temporal resolution was 20 to 30 volumes/sec, depending on sector size.
Evaluation of RT3DE Data
Each RT3DE volume was transferred to the Ventripoint Medical System (VMS) version 1.2.6804.1278 (Ventripoint Diagnostics, Seattle, WA). The participant’s weight and height were entered. After selecting representative 2D echocardiographic imaging planes from single RT3DE data sets, end-diastolic and end-systolic frames were marked. Subsequently, anatomic landmarks such as the RV endocardium, the interventricular septum, the apex, and the pulmonic and tricuspid annulus were marked with points on the end-diastolic and end-systolic frames of each view ( Figure 2 A). Endocardial fiducial points were placed on the inner endocardial border (i.e., on the base of the RV trabeculations. At least 11 points were required for creating a 3D model. This model could be overlaid with the corresponding 2D scan image used to pick a single point of interest ( Figure 3 ). To refine the result, points could be removed, added, or repositioned on the corresponding 2D plane. The resultant 3D model was then used to calculate end-diastolic volume (EDV) and end-systolic volume (ESV) as well as stroke volume (SV) and EF.
The RV models were created with data from the knowledge database in VMS, which were retrieved through a secure Internet connection. The database contains a library of templates for hearts with normal as well as abnormal shapes and sizes characteristic for the various heart diseases of interest (including TOF, systemic right ventricle, and pulmonary hypertension). Database entries are based on piecewise smooth-subdivision surface reconstruction models designed to offer a faithful representation of the 3D shape as well as an accurate volume representation. This method has already been evaluated. A regional statistical process selects the most fitting heart shape for the corresponding set of fiducial points.
CMR Imaging
Participants were placed on the examination table in the supine position. Vector electrocardiographic leads and an abdominal belt for the detection of respiratory motion were attached. Volumetric measurements were performed with a clinical 3.0 T whole-body magnetic resonance imaging system (Achieva 3.0 T TX; Philips Medical Systems) equipped with parallel radiofrequency signal transmission technology for enhancing image uniformity (maximum gradient performance, 80 mT/m; slew rate, 200 T/m/sec). A 32-element phased-array receive-only surface coil was used for signal detection. For assessment of LV and RV volumes, a stack of 15 to 21 short-axis slices was acquired by applying a segmented multislice, multiphase vector electrocardiographically triggered steady-state free precession gradient-echo sequence (repetition time, 2.7 ms; echo time, 1.35 ms; excitation angle, 40°; slice thickness, 5–6 mm; no slice gap). The matrix size was 160 × 240, and the field of view was 384 mm. This provided an in-plane resolution of 1.6 × 1.6 mm and 25 cardiac phases under short end-expiratory breath-holding periods of <12 sec in duration.
Evaluation of CMR Data by Conventional Software
Quantification of the volumetric CMR data was performed offline on a workstation using the threshold-based HDZ MR-Tools software package (labeled “CMR MOD ”; HDZ, Bad Oeynhausen, Germany). A condensed description of the software package is found elsewhere. RV volumes were calculated by summing the products of all cavity (slice) areas and the respective slice thickness.
Evaluation of CMR Data by VMS Software
After importing the CMR stacks into the system, the range of slices needed for RV volumetry was selected. In analogy to the echocardiographic tool, end-diastolic and end-systolic frames and landmarks were marked as described above ( Figure 2 B). Three-dimensional reconstruction was accomplished by the same method as for the RT3DE approach.
Statistical Analysis
RT3DE and CMR data were each analyzed by a different investigator. For interobserver variability, another investigator was introduced who had undergone intensive interoperator training in the use of the VMS software. Intra- and interobserver variability had been previously thoroughly assessed for the CMR MOD software. Blinded intra- and interobserver comparisons for the KBR software were performed with data from 20 individuals selected to cover all four KBR tools (five individuals for each tool). The two readings required for intraobserver variability testing were obtained ≥14 days apart. Descriptive statistics were derived from all relevant continuous variables. Correlation analysis was completed by linear regression analysis and Pearson correlation using SPSS version 21 (SPSS, Inc, Chicago, IL). Bland-Altman analysis was performed to quantify the agreement between the different evaluation algorithms as well as to assess intra- and interobserver variability. The latter were also expressed by intraclass correlation coefficients (ICCs).
Methods
We prospectively enrolled 40 patients with different cardiac defects and RV pathologies. All patients had been referred to our CMR department for diagnostic clinical examination. The majority of investigations were performed in awake and cooperative individuals. In seven younger children unable to hold their breath, CMR and RT3DE imaging were carried out one after the other under anesthesia. Echocardiography was performed in the same laboratory immediately after the CMR examination. To account for RV shapes varying with different diseases, the KBR software provides different references for RV reconstruction in specific malformations. Twenty patients were suitable for the reference “postoperative TOF,” and five patients with dilated right ventricles due to left-to-right shunts were investigated with the tool “pulmonary hypertension” as recommended by the manufacturer. Ten patients had pathologic LV-to-RV interactions due to LV disease, resulting in abnormal LV shapes. This group included patients with cardiomyopathies as well as those with aortic valve disease and impaired LV function. In these patients, we used the “right ventricle” tool for normal right ventricles. Five patients who had undergone Mustard procedures were assessed with the “d-TGA – atrial switch” reference. Additionally, a group of 20 healthy children and young adults was recruited. Their ventricles were assessed with the tool “right ventricle” for right ventricles with normal shape. In addition to a good ultrasound window, we used a modified RV apical view for RT3DE imaging. Mandatory for the inclusion of these healthy individuals were normal results on standard echocardiography as well as sinus rhythm. The study was approved by the local institutional ethics review committee (local registration no. 20/2011) and conforms to the principles of the Declaration of Helsinki and to German law. Informed written consent was obtained from all participants and/or their legal guardians before participation.
RT3DE Imaging
A single sonographer with 7 years of experience conducted all RT3DE studies with an iE33 system with an X5-1 transducer (Philips Medical Systems, Best, The Netherlands) or a Vivid E9 with a V4 transducer (GE Medical Systems, Milwaukee, WI). Starting from the position of a standard five-chamber view, the probe was angulated upward and toward the left until inflow, outflow, and apex of the right ventricle became visible. This view resembles a short-axis view to a certain extent but also includes the entire apical portion of the right ventricle. RT3DE volumes were acquired from an apical view with the RV apex next to the transducer and also depicting the complete inflow and outflow portions. On the basis of this 2D planning view ( Figure 1 ), one full-volume data set was acquired including four 90° to 110° subvolumes under a single end-expiratory breath-hold with stable transducer position. Temporal resolution was 20 to 30 volumes/sec, depending on sector size.
Evaluation of RT3DE Data
Each RT3DE volume was transferred to the Ventripoint Medical System (VMS) version 1.2.6804.1278 (Ventripoint Diagnostics, Seattle, WA). The participant’s weight and height were entered. After selecting representative 2D echocardiographic imaging planes from single RT3DE data sets, end-diastolic and end-systolic frames were marked. Subsequently, anatomic landmarks such as the RV endocardium, the interventricular septum, the apex, and the pulmonic and tricuspid annulus were marked with points on the end-diastolic and end-systolic frames of each view ( Figure 2 A). Endocardial fiducial points were placed on the inner endocardial border (i.e., on the base of the RV trabeculations. At least 11 points were required for creating a 3D model. This model could be overlaid with the corresponding 2D scan image used to pick a single point of interest ( Figure 3 ). To refine the result, points could be removed, added, or repositioned on the corresponding 2D plane. The resultant 3D model was then used to calculate end-diastolic volume (EDV) and end-systolic volume (ESV) as well as stroke volume (SV) and EF.
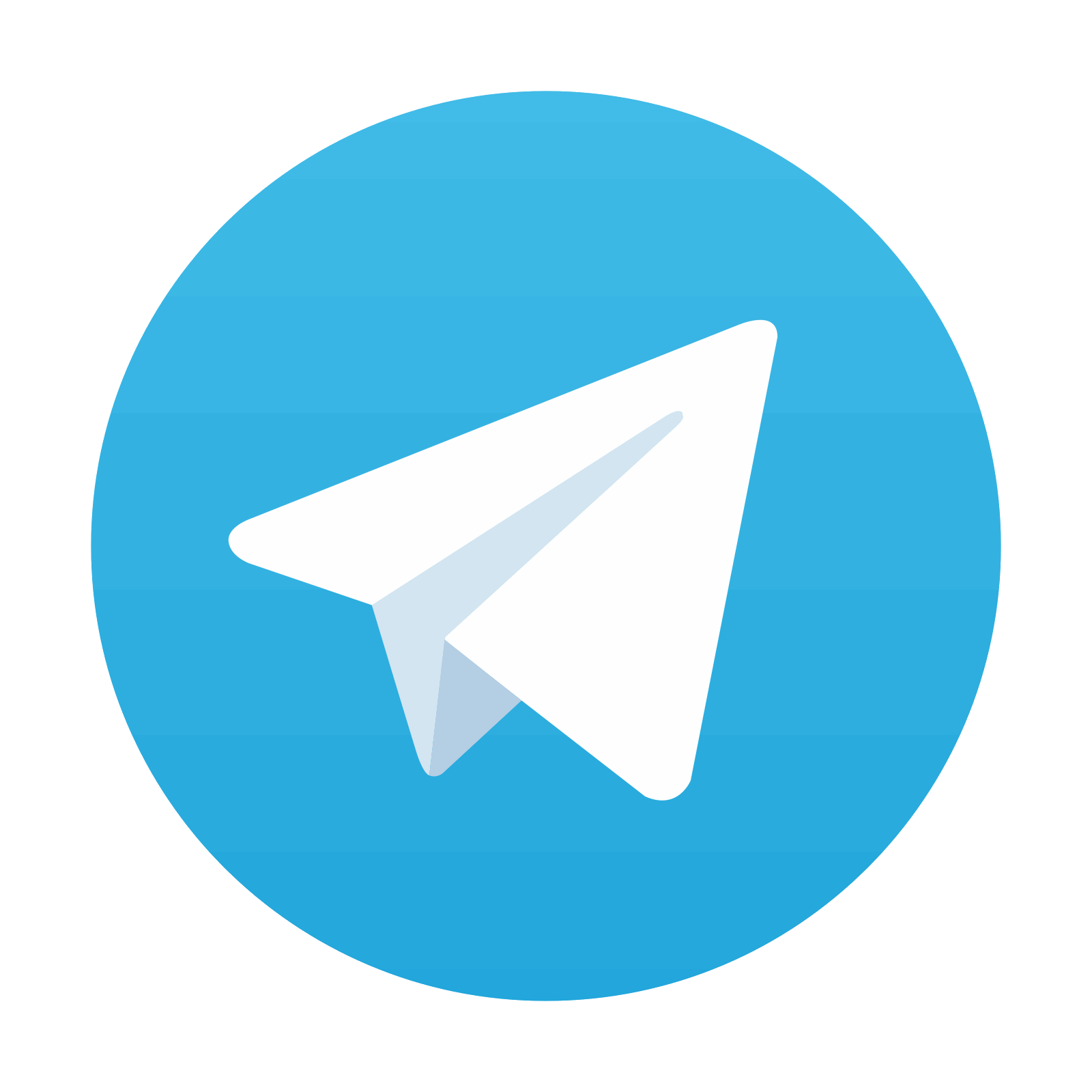
Stay updated, free articles. Join our Telegram channel

Full access? Get Clinical Tree
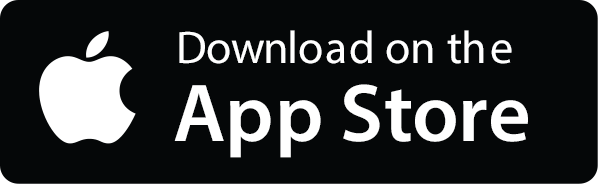
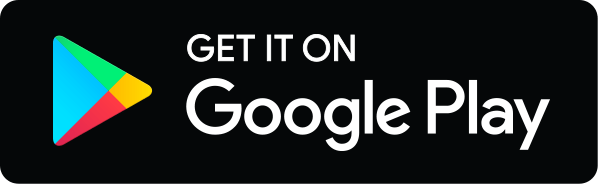
