A 75-year-old woman presents with effort intolerance, progressive dyspnea on exertion to minimal activity, and progressive feet, ankle, and lower leg swelling. She has a 3-year history of idiopathic nonischemic cardiomyopathy with a left ventricular ejection fraction (LVEF) of 35% measured within the last year. She also has a history of obesity, type 2 diabetes mellitus for 15 years with diabetic nephropathy, and hypertension (HTN) for 25 years. The medication profile includes: furosemide 40 mg orally twice a day, carvedilol 25 mg orally twice a day, enalapril 10 mg orally twice a day, and glargine insulin 60 units subcutaneously every night at bedtime. Vital signs were blood pressure of 105/70 mm Hg, heart rate 95 beats per minute, respiration rate 20 breaths per minute, weight 82 kg, and body mass index 33 kg/m2, temperature 98.4°F. Her physical examination reveals jugular venous distention, rales one-half of the way up posteriorly, cardiac enlargement, an S3, 2/6 murmur consistent with mitral regurgitation, hepatomegaly with hepatojugular reflux, and 4+ pitting edema to the midtibia bilaterally, and no visible rashes. The chest x-ray demonstrated cardiomegaly and pulmonary edema and the electrocardiogram revealed normal sinus rhythm, left axis deviation, left bundle branch block, and QRS duration of 120 ms. Laboratory testing was notable for: Na = 133 mEq/L, K = 5.0 mEq/L, creatinine 1.7 mg/dL (estimated glomerular filtration rate [eGFR] = 29 mL/min/1.73 m2), BUN = 48 mg/dL, glucose = 235 mg/dL, glycohemoglobin = 7.2% and B-type natriuretic peptide = 2765 pg/mL. The urine albumin to creatinine ratio was 315 mg/g. The patient initially received furosemide 80 mg intravenously twice a day with a good diuresis, but on the third hospital day the creatinine rose to 2.9 mg/dL and the urine output dropped to 4 mL/kg for 12 hours. The patient’s pulmonary examination improved somewhat; however, the S3 and peripheral edema were essentially unchanged. The managing team was confronted with a series of questions regarding the differential diagnosis, prognosis, and next management steps.
The differential diagnosis includes type 1 cardiorenal syndrome, interstitial nephritis, subclinical sepsis, and prerenal azotemia. Because the patient has considerable evidence of volume expansion, it is unlikely that there has been enough volume loss to have caused prerenal azotemia. The presence of jugular venous distention suggests she has elevated central venous pressure, which is the strongest hemodynamic determinant of type 1 cardiorenal syndrome. While the kidneys may not be receiving enough forward output and there may transiently be a slowed plasma refill from the extravascular space, the patient is very unlikely to be truly volume depleted. There was no evidence of fever or focal infection; hence the overlap between infection and heart failure (HF), while common, is probably not the issue in this patient. Interstitial nephritis generally presents with rash, fever, and urinary eosinophils, all of which are absent in this patient. Additionally, she has not taken any medications that commonly induce this syndrome. We are left with a working diagnosis of type 1 cardiorenal syndrome in which the onset of acute heart failure (AHF) has led to an attempt at diuresis and then a marked reduction in glomerular filtration and urine output.1
We are in the midst of an HF chronic disease pandemic with the aging of populations in the Western world.2 Survivorship in the settings of long-standing hypertension, myocardial infarction, valvular disease, and myocardial disease has led to an increased prevalence pool of patients with established HF. Approximately half of patients with HF have reduced LVEF or HFrEF and the other half have HF with preserved LVEF, or HFpEF. HFrEF can be attributed to myocardial ischemia or prior infarction in two-thirds of cases, while approximately one-half of those with HFpEF have a significant contribution to their illness due to ischemia as a result of coronary artery disease (CAD).3 Because of the considerable overlap between HTN, diabetes, and other risk factors for CAD and myocardial disease with chronic kidney disease (CKD), it is a common occurrence to find HF patients (either HFrEF or HFpEF) who also have evidence of CKD, such as reduced estimated glomerular filtration rate (eGFR <60 mL/min/1.72 m2), albuminuria (≥30 mg/g albumin to creatinine ratio in spot urine), or structural abnormalities in the kidneys or urological tract (eg, polycystic kidney disease, unilateral kidney).4 Additionally, it is well known that in the setting of AHF decompensation, that acute kidney injury (AKI) occurs in approximately 25% of hospitalized patients (type 1 cardiorenal syndrome).5 Finally, evidence suggests that HF itself can set up renal pathophysiology in a way to manifest reduced eGFR or albuminuria. This scenario has been termed type 2 cardiorenal syndrome.6 Thus there is a considerable interface between cardiac and renal function in both health and disease. This chapter provides a framework to understand the kidney in HF from a graphical and pictorial perspective.
A normal human body has approximately 5 liters of blood volume and a cardiac output of 3 to 5 liters per minute at rest. Cardiac output can increase to ∼35 liters per minute with aerobic exercise such as running. There is an important Frank-Starling relationship between end-diastolic volume and forward stroke work, which is analogous to the volume per contraction that would partially perfuse the kidneys as shown in Figure 7-1. At rest the parasympathetic system via acetylcholine release predominates over the sinoatrial node and maintains the heart rate in the 50 to 100 beats per minute range. In athletes, parasympathetic tone can be more pronounced and result in even lower sinus rates. With exercise, the sympathetic nervous system via the release of norepinephrine predominates and the sinus node rate increases. Additionally, contractility of the myocardium becomes more forceful, resulting in greater ventricular systolic pressures. The sympathetic nervous system achieves increased cardiac output by increasing heart rate, end-diastolic volume, and stroke volume (Figure 7-2). In order for these responses to occur there must be increased venous return to the heart.
At rest approximately 60% of blood volume is in the venous system at any given time (Figure 7-3). The veins have much thinner walls than the arteries and thus have a much greater capability to dilate creating potential for pooling of blood volume. The tunica media in veins is innervated by the sympathetic nervous system and stains intensely for norepinephrine from sympathetic neuromuscular terminals. This innervation works to control venous tone, and thus provides minute-to-minute regulation of venous return to the heart, which has an important pressure relationship to the overall cardiovascular system as shown in Figure 7-4. As shown in Figure 7-4, the hemodynamic performance of the right-sided chambers, pulmonary circulation, and left-sided systemic chambers are all highly and dynamically dependent on venous return.
The kidneys receive arterial blood flow through the renal arteries, which arise from the abdominal aorta just inferior to the superior mesenteric artery. Each kidney receives approximately 600 mL/min of flow and together renal blood flow (RBF) represents ∼20% of cardiac output. The kidneys receive a disproportionate share of cardiac output positioned distally in the aorta because the renal circulation is a very low resistance circuit. This phenomenon has been termed a “vascular waterfall” and the kidneys allow blood to move in response to a ~100 fall in pressure, which is roughly double that of other organ systems including the heart. The renal artery (Figure 7-5) subdivides into segmental branches, then arcuate branches, and ultimately into afferent arterioles that deliver blood to the glomerular tuft and reconstitute as efferent arterioles that go on to form the peritubular network, vasa recta, and then subsegmental and segmental renal veins, which converge on the main renal veins back to the inferior vena cava. This valveless system carries a large blood volume back to the heart and thus is vulnerable to changes in forward perfusion pressure, back pressure, or changes in organ fluid content. Because the kidneys are in the retroperitoneal space unlike other viscera, there is lesser tolerance for organ expansion in the setting of organ edema. Multiple studies have shown that measures of central venous pressure, inferred renal venous pressure, and reduced outflow are strong determinants of type 1 cardiorenal syndrome in patients with AHF.7
Intrarenal autoregulatory mechanisms maintain RBF andglomerular filtration rate (GFR) independent of renal perfusion pressure (RPP) over a broad range of systemic arterial pressure (80-180 mm Hg).8 Such autoregulation is mediated largely by the myogenic response, in which smooth muscle cells of the afferent arteriole sense changes in arteriolar pressure and adjust their tone accordingly to avoid fluctuations in blood flow to the glomerular tuft (Figure 7-6). Additional autoregulation is achieved by the tubuloglomerular feedback mechanism, in which afferent arteriole tone is adjusted in response to changes in NaCl content of tubular fluid. The components of this mechanism will be discussed shortly. The glomerulus is a unique vascular structure with multiple layers that constitute the filtration barrier between plasma and urine, including: (1) glycocalyx, (2) fenestrated endothelium, (3) glomerular basement membrane, formed by fusion of podocyte and endothelial basement membranes, and (4) foot processes of podocytes. The glomerulus also houses the mesangium, juxtaglomerular apparatus, and macula densa cells, which serve a variety of regulatory processes. The mesangial cells have cytosolic contractile proteins that enable them to change shape and regulate blood flow into the glomerulus via the afferent arteriole. Angiotensin II is an important regulator of this function. The juxtaglomerular apparatus refers to the close arrangement of the distal tubule, extraglomerular mesangium, and the afferent arteriole containing specialized juxtaglomerular cells. The juxtaglomerular portion of the distal tubule contains darkly staining, prominently nucleated macula densa cells, which are both the sensing and signaling component of the previously mentioned tubuloglomerular feedback mechanism. In addition to regulating arteriolar tone, the macula densa cells respond to changes in distal tubular NaCl by regulating renin release from the nearby juxtaglomerular cells.9 Thus the anatomy and normal physiology of renal perfusion and glomerular filtration is particularly responsive to changes in both forward flow and venous return. The sodium-glucose transporter-2 channels are located in the proximal tubule and are a major target for a new class of antidiabetic agents, SGLT-2 inhibitors which have been associated with reductions in heart failure hospitalization and cardiovascular death. Additionally, the peritubular network (Figure 7-7) is the site where renal tubules in close proximity to both the tubular lumen and the blood-capillary interface regulate sodium, chloride, ammonium, and bicarbonate in the urine. Each distal convoluted tubule is drained into a collecting duct, and thus, each collecting duct services approximately 4 to 8 nephrons (Figure 7-8). The overall pathway of blood flow through the kidney is depicted in Figure 7-9. The principal cell in the collecting duct has 2 major functions that are relevant in HF: (1) effector response to intranuclear signaling from aldosterone, prompting the cell to reclaim sodium and dump potassium, contingent on distal delivery of sodium, and (2) effector response to cell surface activation of vasopressin 2 receptors to arginine vasopressin, which prompts aquaporin channels to incorporate into the lumen membrane, allowing for reclamation of water.10 Because of the close proximity of the collecting ducts to the vasa recta, both of these systems work to deliver large amounts of sodium and water to the bloodstream when these hormonal systems are activated.
In summary, the kidneys are positioned to be the most hemodynamically and neurohormonally responsive to changes in blood volume, flow, perfusion, back pressure, sodium, and water in the setting of HF. As a result, both chronic and acute renal filtration function are 2 of the most important parameters in the prognosis and management of patients with all forms of HF.
It is beyond the scope of this chapter to discuss in detail the role of each neurohormone in detail that is participating in normal physiology, as well as the pathophysiology of HF. In brief, there are multiple important regulatory systems that are activated in HF that work toward preserving perfusion to the brain at the expense of the kidneys, and direct the maximal amount of sodium and water reclamation possible despite the adverse consequences of responses. The sympathetic nervous system releases norepinephrine via peripheral synapses at the neuromuscular junctions within afferent and efferent renal arterioles as well as mesangial cells. This results in stimulation of both alpha- and beta-adrenergic receptors, which in turn decrease RBF, increase intraglomerular pressure, and increase sodium retention. These effects result in a decrease in renal blood flow, an increase in intraglomerular pressure, and increased sodium retention. Norepinephrine is a stimulus for juxtaglomerular cells to release renin, which is the starting point for the renin angiotensin system. In addition to norepinephrine, both epinephrine and dopamine as precursor molecules have effects on the kidneys primarily in the proximal and distal tubule with varying effects depending on the family of receptors. In general, both epinephrine and norepinephrine stimulate the reabsorption of salt and water. Dopamine, however, acting on a different family of receptors, can stimulate natriuresis and diuresis. In general, the epinephrine and norepinephrine effects are more powerful and the net effect of sympathetic stimulation to the kidneys is release of renin, reduction in RBF, increased salt and water retention, and a slightly reduced GFR.
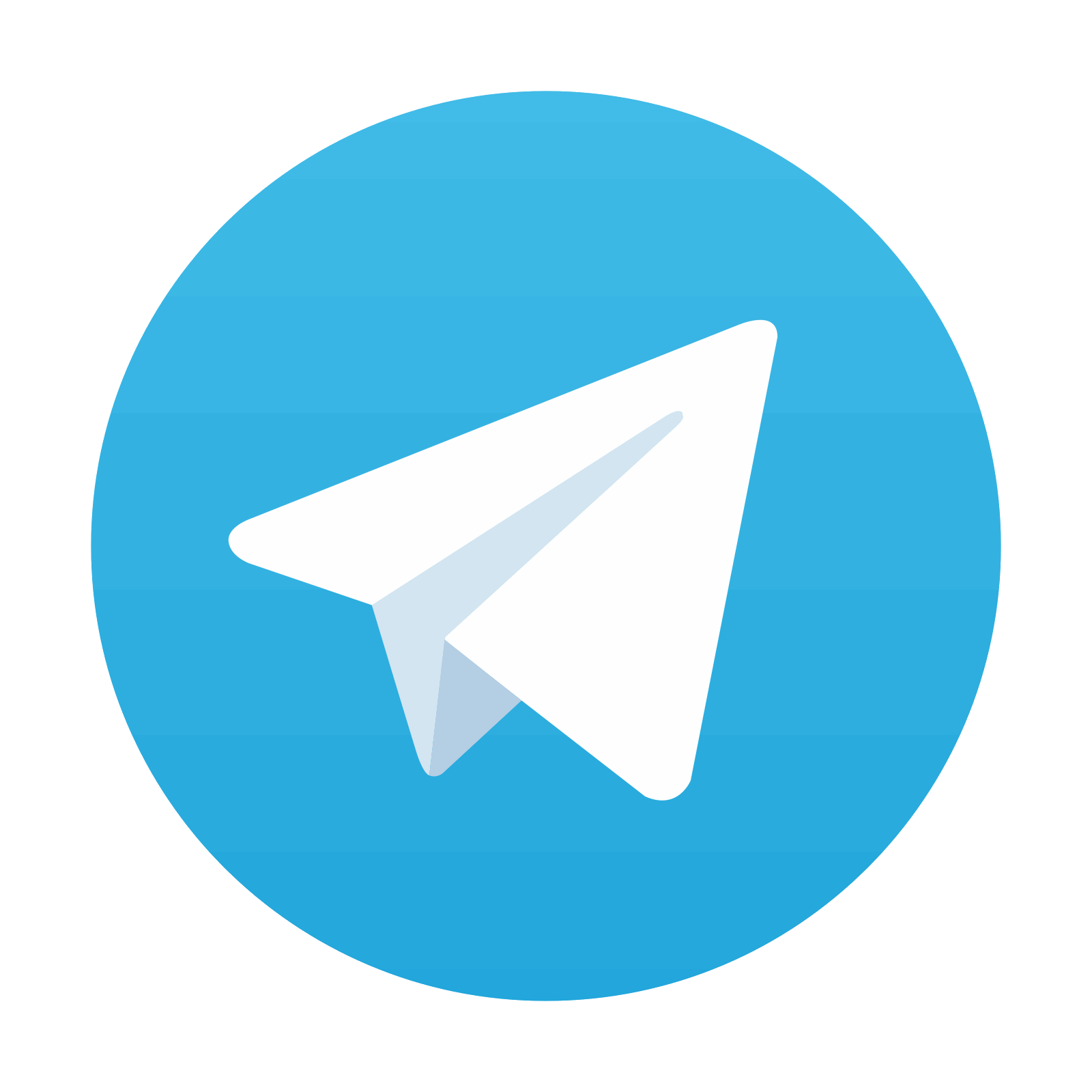
Stay updated, free articles. Join our Telegram channel

Full access? Get Clinical Tree
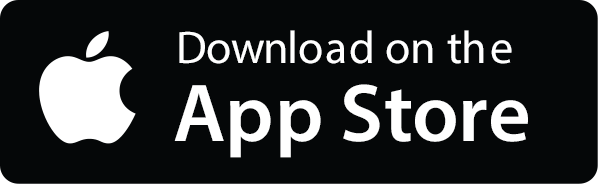
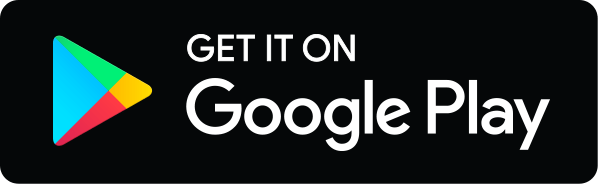