Minutes
Energy failure
Excitotoxicity
Increase in intracellular Ca2+, Na+, Cl−
Peri-infarct depolarization
Diffusion of glutamate and K+ in the extracellular space
Cell swelling
Hours
Generation of reactive oxygen species
Oxidative stress
Increased inducible nitric oxide synthase (iNOS) activity
Nitrosative stress
Free radicals damage membranes, mitochondria, and DNA
Early postischemic inflammation
Free radicals activate caspases and inflammatory mediators
Early blood-brain barrier dysfunction
Upregulation of adhesion molecules promotes leukocyte infiltration
Microvascular injury
Microglial activation
Cytokine receptor activation
Upregulation of matrix proteases
Days
Matrix protease activation
Late blood-brain barrier dysfunction
Proapoptotic signaling and cytotoxic proteins
Late postischemic inflammation
Apoptosis
Remodeling
IL-1β and TNF-α are the cytokines that mediate the initial inflammatory response [2]. IL-1β and TNF-α subsequently induce a secondary inflammatory response, more lasting, mediated by IL-6 and IL-8. These cytokines play an important role in the development of acute-phase reactants, such as C-reactive protein (CRP) and fibrinogen, and in the release of cell adhesion molecules, which contribute to leukocyte aggregation and later adhesion to vascular wall [3]. The overexpression of IL-6 by microglia and astrocytes may have a dual role in acute ischemia, with potential neurotoxic and neuroprotective actions [4]. CRP and pentraxin 3 (PTX3) are members of the pentraxin family, which plays a major role in the human innate immune response. PTX3 activates the complement pathway and enhances macrophage and dendritic cell activity. Microglia is also activated after cerebral ischemia. Activated microglia secretes cytokines such as TNF-α and develops phagocytic and major histocompatibility complex (MCH) class II-restricted antigen presenting features. Microglia can also produce growth factors like brain-derived neurotrophic factor (BDNF) [5]. The inflammatory mediators that are produced during the innate immune response induce the expression of cellular adhesion molecules (CAMs) that mediate leukocyte recruitment, transendothelial migration, and adhesion to the vascular endothelium. Leukocytes present in the ischemic area produce more inflammatory mediators, which activate adaptive immune response [6].
All these inflammatory responses are an important determinant of the clinical outcome, lesion size, edema, and hemorrhagic transformation. The breakdown of the blood-brain barrier (BBB) allows the passage of some of these substances into the peripheral circulation and cerebrospinal fluid, where they can be measured. S100B is assumed to be a marker of generalized BBB dysfunction. S100B belongs to a multigene family of calcium-binding proteins. Peripherally found biomarkers related to inflammation may therefore be clinically useful in ischemic stroke.
Biomarkers in the Clinical Setting of Ischemic Stroke
Diagnosis of Acute Ischemic Stroke
About a third of patients who present with stroke-like symptoms have a stroke mimic. Although brain magnetic resonance imaging (MRI) with diffusion sequence may be very useful for stroke diagnosis, its sensitivity for ischemic stroke has been reported to be of 92 % with a specificity of 75 %. DWI-negative MRI was particularly associated with less severe stroke (NIHSS 4 vs. 7), posterior circulation location, higher prevalence of small vessel disease, and longer time from symptom onset to imaging (120 min vs. 109 min) [7]. Hence, biomarkers capable of identifying patients with ischemic stroke might be clinically useful.
One of the most promising biomarkers for acute ischemic stroke is S100B. S100B is a calcium-binding protein synthesized by astrocytes and expressed primarily by these cells in the central nervous system (CNS) and to a lesser extent by Schwann cells. In a normal status, the concentration of S100B is 40-fold higher in CSF than in serum. Serum S100B levels have been shown to be significantly increased following ischemic stroke from 10 h to 2–3 days after stroke onset [8]. Serum levels of S100B were pointed to be useful for distinguishing between posterior circulation strokes and nonvascular vertigo. Two observational prospective studies found statistically significant higher levels of S100B in patients with posterior circulation stroke than in patients with noncentral causes of vertigo [9, 10]. Receiver-operating characteristic analysis revealed a sensitivity of 94.4 % and a specificity of 31.8 % for detecting stroke in patients presenting with vertigo for S100B [9].
Higher plasma levels of TNF-α, IL-6, and IL-1β in acute ischemic stroke have been found mainly in cardioembolic strokes [11].
Cell adhesion molecules (CAMs) released into the bloodstream may also be useful for the diagnosis of ischemic stroke. Previous studies showed higher serum levels of soluble intercellular adhesion molecule-1 (sICAM-1) and soluble vascular cell adhesion molecule-1 (sVCAM-1) in stroke patients on admission, compared to healthy controls [12].
The diagnostic accuracy of a biomarker panel to diagnose acute stroke including MMP-9 and S100B was evaluated in a prospective multicenter trial – the Biomarker Rapid Assessment in Ischemic Injury (BRAIN) study [13]. Within a 3-year period, 1,146 patients presenting with symptoms suspicious for stroke were enrolled. The multivariate model was capable of only moderately differentiating between stroke patients and mimics. Setting a threshold to the 25th percentile, it was possible to show a sensitivity of 86 % and a specificity of 37 % for discriminating stroke patients from mimics [13].
Stroke Severity
The need for biomarkers (a “brain troponin”) that can reflect the severity of real-time brain injury has increased with the development of acute stroke therapies. Such biomarker can speed up decisions regarding the appropriateness of chemical or mechanical revascularization.
Several biomarkers showed an association with stroke severity as indicated by a more severe clinical picture and a larger infarct volume. S100B has a positive correlation with total infarct volume [14] and admission National Institute of Health Stroke Scale (NIHSS) scores [15]. Increased S100B in blood is not specific for cerebral infarction, as increases occur in other neurologic diseases, like traumatic brain injury. Higher levels of glial fibrillary acidic protein (GFAP) [16] and IL-6 [17] are also associated with larger infarct volumes.
MMPs are implicated in numerous pathogenic mechanisms in acute ischemic stroke, including BBB disruption. MMP-9 serum level was significantly increased after stroke onset, correlating with infarct volume and stroke severity [18]. In acute ischemic stroke higher baseline blood MMP-9 levels are associated with thrombolysis failure [19].
In a recent study, adiponectin values at day 0 were positively associated with neurological severity as evaluated by the NIHSS at admission [20]. Neuron-specific enolase (NSE) was also significantly correlated with the degree of neurological deficit (NIHSS on admission) [21]. The expression of vascular endothelial growth factor (VEGF) was correlated with infarct volume and clinical disability (Scandinavian Stroke Scale) [22]. d-dimer levels at admission were also associated with total infarct volume in patients with acute ischemic stroke and atrial fibrillation [23]. The excitatory neurotransmitter glutamate was associated with infarct growth [24]. Elevated neuropeptide proenkephalin A (PENK-A) concentrations were also associated with the severity of ischemic stroke, assessed by the NIHSS and modified Rankin Scale [25].
Hemorrhagic Transformation
Hemorrhagic transformation (HT) is more likely to occur with increasing infarct size, early recanalization, cardioembolic etiology, and use of recombinant tissue plasminogen activator (rtPA). Early HT (less than 24 h after stroke onset) has been associated to MMP-9 and MMP-2 activation. MMP-9 is a proteolytic enzyme involved in tissue remodeling [26]. These metalloproteinases damage the neurovascular unit and lead to BBB disruption. Delayed HT (more than 24 h after stroke onset) has been related to the activation of MMP-2, MMP-3, MMP-9, and endogenous tissue plasminogen activator, neuroinflammation, and factors that promote vascular remodeling [26].
Increased MMP-9 levels measured in plasma samples of patients before rtPA administration (>140 ng/ml) were associated with HT [27]. The sensitivity, specificity, and positive and negative predictive values for HT by MMP-9 levels > 140 ng/mL were 92 %, 74 %, 26 %, and 99 %, respectively. When plasma levels of cellular fibronectin (c-Fn) ≥ 3.6 μg/mL were used in combination with MMP-9 activity, the specificity increased to 87 % and the positive predictive value increased to 41 % [28]. c-Fn is a component of the basal lamina that is synthesized and secreted by endothelial cells. When the basal lamina is disrupted, c-Fn is released into the plasma, leading to the recruitment of leukocytes.
Vascular adhesion protein-1 (VAP-1), a cell surface and circulating enzyme involved in the recruitment of lymphocytes and neutrophils through its semicarbazide-sensitive amine oxidase (SSAO) activity, has also been shown to predict HT. In an observational, prospective study of 141 ischemic stroke patients, significantly higher levels of plasma VAP-1/SSAO activity were present in patients who subsequently experienced HT [29].
Malignant Middle Cerebral Artery Infarction
Space-occupying brain edema is a life-threatening complication in patients with large hemispheric stroke. In 80 % of patients it leads to death, and patients who survive have severe neurological deficits.
In a study of 51 patients admitted within 6 h after stroke symptom onset caused by proximal middle cerebral artery (MCA) occlusion, 16 developed a malignant MCA infarct. In these patients, a 12-h S100B value > 0.35 μg/L predicted malignant infarction with a sensitivity of 75 % and a specificity of 80 %. A 24-h value > 1.03 μg/L provided a sensitivity of 94 % and a specificity of 83 % [30].
In an observational, prospective study of 40 consecutive patients with malignant MCA infarctions and 35 controls with massive MCA infarctions, less than 70 years of age and matched by stroke severity on admission, plasma c-Fn levels greater than 16.6 μg/mL were associated with the development of malignant infarctions with a sensitivity of 90 %, specificity of 100 %, and negative and positive predictive values of 89 and 100 %, respectively [31].
There are discrepant results regarding the role of MMP-13 as an independent predictor of infarct growth 24 h after stroke onset. Although an association was initially shown, subsequent studies could not detect significant differences in MMP-13 blood levels after stroke compared with controls [32, 33].
Ischemic Stroke Recurrence
Elevated blood CRP levels are a predictor of the risk of cardiovascular events, as they are known to be associated to endothelial cell dysfunction and decreased production of nitric oxide [34]. In a nested case-control study derived from the Perindopril Protection Against Recurrent Stroke Study (PROGRESS), inflammatory markers associated with the acute-phase response (IL-6, TNF-α, CRP, and fibrinogen) were associated with risk of recurrent stroke (>3 years of outcome). However, these markers were dependent on each other in multivariate models, and once all were included, only TNF-α retained a borderline association [35]. In a study that included transient ischemic attack and minor stroke patients, CRP was not associated with recurrent vascular events [36]. Also, in the MITICO study, a multicentered prospective observational study designed to assess the prognostic value of markers of inflammation in relation to the risk of recurrence of ischemic stroke, CRP was not independently associated with recurrent ischemic stroke within the first year after the index event. Only baseline levels of IL-6 > 5 pg/mL and VCAM-1 > 1,350 ng/mL were associated with vascular disease recurrence risk (OR: 28.7; 95 % CI: 14.2–58.0 vs. OR: 4.1; 95 % CI: 2.4–7.1, respectively) following adjustment for confounding variables [37]. This independent association of IL-6 or VCAM-1 with stroke recurrence was not reproduced in further studies [38, 39].
The majority of studies that analyzed stroke recurrence combined different stroke etiologies. However, biomarker profiles regarding stroke recurrence may differ between lacunar stroke and other ischemic stroke subtypes. In the study Levels of Inflammatory Markers in the Treatment of Stroke (LIMITS), an international, multicenter, prospective biomarker study nested within Secondary Prevention of Small Subcortical Strokes (SPS3), a phase III trial in patients with recent lacunar stroke, among recent lacunar stroke patients, CRP levels predicted the risk of recurrent strokes. In patients with an initial lacunar stroke compared with the bottom quartile, those in the top quartile (CRP > 4.86 mg/L) were at increased risk of recurrent ischemic stroke even after adjusting for demographics and risk factors (adjusted HR, 2.32; 95 % CI, 1.15–4.68) [40].
Lipoprotein-associated phospholipase A2 (Lp-PLA2) is an inflammatory biomarker that has been described as an independent risk marker for recurrent events in ischemic stroke [41]. Lp-PLA2 is an enzyme that hydrolyzes oxidized phospholipids, releasing lysophosphatidylcholine, that has pro-inflammatory properties. It is considered to be involved in the development of atherosclerosis and plaque rupture [41]. In 2005, the Lp-PLA2 blood test was approved by the US Food and Drug Administration (FDA) for assessing the risk of ischemic stroke [41].
The Northern Manhattan Stroke Study analyzed the predictive role of Lp-PLA2 drawn at the time of initial stroke in predicting the risk of recurrent stroke. In this population-based study of 467 patients with a first ischemic stroke, Lp-PLA2 conferred a 2.1-fold increase in recurrent stroke risk (HR, 2.08; 95 % CI, 1.04–4.18) [42].
Patients with elevated erythrocyte sedimentation rate (ESR) have been shown to have a greater risk of recurrence within 2 weeks of stroke onset [43].
Prognosis
Several studies have demonstrated an association between blood biomarkers of several pathophysiological processes and outcome after stroke. In most cases, however, it was not demonstrated if measurement of such biomarkers had an increment on prognostic accuracy over existing clinical and imaging predictors. This fact strongly limits their clinical significance [17].
Cardiac Biomarkers
A number of studies consistently found an increased mortality in stroke patients with increased cardiac troponin I or T [44–48], including the recent large biomarker substudy of the RE-LY trial (Randomized Evaluation of Long-Term Anticoagulation Therapy) [49]. Although some negative results were published [17], it was demonstrated in a retrospective study that adding troponin levels to a multivariate clinical model predicting long-term mortality increased the discriminative power of the model [50]. This effect persisted independently of traditional major coronary risk factors [51]. Some authors consider that troponin levels may reflect neurogenic cardiac damage, which is more frequent in severe strokes and is by itself a cause of acute stroke mortality. An ongoing prospective study in a large cohort of stroke patients is aiming to determine the causes of troponin elevation using coronary angiography [52, 53].
In a recent study with more than 400 patients, brain natriuretic peptide (BNP) was an independent prognostic marker for overall mortality in patients with ischemic stroke or TIA [54].
Inflammatory Biomarkers
In a recent meta-analysis evaluating the prognostic value of blood IL-6 in the prediction of functional outcome after stroke, standardized IL-6 levels in the 4th quartile were independently associated with a poor outcome (OR = 2.346). However, the additional predictive value of IL-6 was only moderate [55]. Higher levels of plasma IL-6 were also associated with death within the first 2 years after a stroke [56] and increased risk of future infection [56]. Higher MMP-9 levels were also correlated with functional outcomes [18].
CRP is an acute-phase-response protein, which may itself have pro-inflammatory effects and increase secondary brain injury. There is a significant association between elevated baseline high-sensitivity CRP levels and unfavorable long-term functional outcomes [57]. This association is present in a dose-related manner [58]. Elevated serum CRP levels in the acute and subacute phases (0–15 days) independently predicted mortality in several large cohort studies [58, 59], including in patients submitted to intravenous thrombolysis, regardless of vessel recanalization. However, measurement imprecision and confounding by infection and nonspecific inflammation are important limitations in considering CRP as a biomarker in acute stroke. The levels of fibrinogen that is also an acute-phase protein similarly are a predictor of poor functional outcome of stroke [60].
Elevated neuropeptide proenkephalin A (PENK-A) concentrations may have prognostic value for fatal and nonfatal events [25]. In one study, chemokines CCL17 and CCL22 showed a very faint power to discriminate patients who improve within the first 24–48 h after stroke from the remaining. Indeed, none of these chemokines seems to be a reliable prognostic biomarker in the hyperacute phase of stroke [61].
Other Biomarkers
Copeptin is a hypothalamic hormone derived from the precursor of vasopressin. Copeptin levels were shown to independently predict outcome and mortality in acute stroke. Also, in a multivariate model, copeptin improved significantly prediction when added to the NIHSS score and age [62]. The excitatory neurotransmitter glutamate is associated with poor outcome [24] and glial fibrillary acidic protein (GFAP) was positively correlated with the outcomes [16]. S100B blood levels at 48–96 h after stroke onset correlate positively with functional outcome in patients with nonlacunar MCA infarctions [63]. High plasma S100A12 levels on admission are associated with a poor outcome [64]. In a recent study, adiponectin values at day 0 were positively associated with poor outcome (modified Rankin Scale ≥ 3 on day 90) [20].
Trials of Immune Treatment in Acute Stroke
A few randomized controlled trials (RCT) have tested the hypothesis that intervening in the immune response within the ischemic cascade could improve stroke outcome. Unfortunately, despite all the encouraging results in basic science and animal models, results were deceiving as all these trials were negative. Enlimomab, a murine intercellular adhesion molecule 1 (ICAM-1) antibody, was tested against placebo in an RCT that included 625 patients treated within 6 h after stroke onset. Enlimomab was not effective and increased significantly the risk of infections and fever [65]. An adaptive dose-response trial of a neutrophil inhibitory factor succeeded to enroll 966 acute stroke patients, but was early terminated for futility [66]. An interleukin receptor antagonist was safe and well tolerated in acute stroke patients [67], but it was not tested in a phase III study. A trial of interferon beta completed enrollment, but results were not published [68, 69]. Arundic acid (ONO-2506), a novel modulator of astrocyte activation, was tested in a dose-escalating, randomized, double-blind phase I trial in 92 patients with acute ischemic stroke, within 24 h of stroke onset [70]. The study drug was infused for 1 h daily over 7 days. No dose-related pattern of serious adverse events was detected. In an exploratory efficacy analysis, a dose of 8 mg/kg/h produced a favorable trend in reduction of NIHSS score, needing confirmation in a future clinical trial, whose results were not published [71]. Minocycline is an antibiotic that acts via several pathways, including inhibition of microglial activation and reduction of T-cell migration. Minocycline was evaluated in a randomized controlled pilot trail including 95 patients treated within 24 h from stroke onset. The drug was safe, but not efficacious. A meta-analysis including this study and two other trials suggested that minocycline may increase the odds of survival free of handicap, but there was substantial heterogeneity between trials [72].
< div class='tao-gold-member'>
Only gold members can continue reading. Log In or Register a > to continue
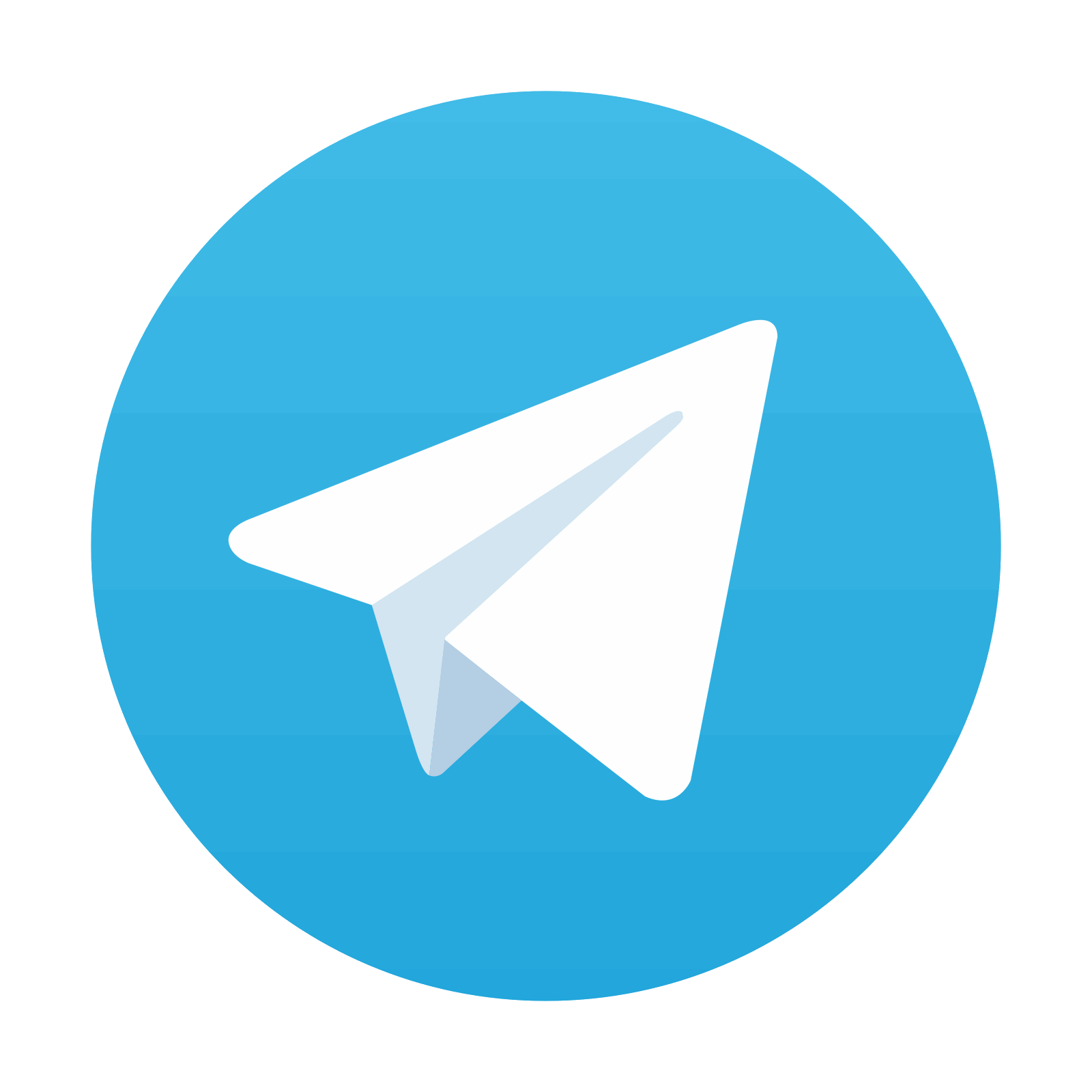
Stay updated, free articles. Join our Telegram channel

Full access? Get Clinical Tree
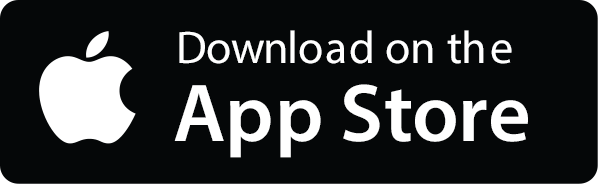
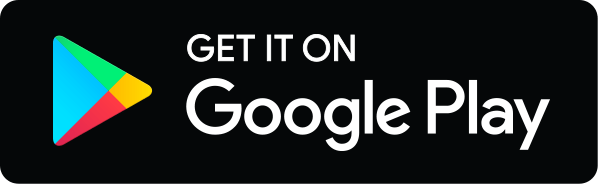