Fig. 1.1
Dr. Domingo Liotta (left) and his TAH (right)
My own involvement with MCS systems dates from 1965, when I was a medical student at Baylor. All medical students were required to have a research project, and as a result I worked under Liotta’s direction on the TAH. Although the Baylor TAH worked well for short periods in calves, none of the early experiments resulted in 24-h survival. As a result, DeBakey decided that the TAH was unready for clinical use. The clinical efforts of the program shifted to the partial artificial heart or left ventricular assist device (LVAD). In 1966, DeBakey performed the first successful clinical implantation of an LVAD. The patient, a 37-year-old woman, was supported by the device for 10 days. During this period, she recovered sufficiently that the pump could be removed. By the late 1960s, DeBakey had used the LVAD in several patients, two of whom survived [8]. I was on his service at the time, and the experience further enhanced my dedication to this endeavor.
On December 9, 1967, the first human heart transplant was performed on December 9 by Christiaan Barnard, in Cape Town, South Africa. The initial results were so promising that the procedure was soon adopted by many other surgeons throughout the world. Application of this therapy was severely limited by the scarcity of donor hearts. At Baylor, Dr. Liotta envisioned that the TAH could serve as a short-term “bridge” until a donor heart was available to heart transplantation. At that time, Denton Cooley was also on the staff at Baylor. As a renowned cardiovascular pioneer, Cooley was the world’s most experienced heart surgeon. He had founded the Texas Heart Institute (THI), of which he was surgeon-in-chief. In May 1968, Cooley performed the first successful heart transplant in the USA [9]. Within a few months, he had gained the world’s most extensive experience with this procedure. In late 1968, Liotta approached Cooley and asked if he would consider using a TAH as a bridge to transplantation. Cooley agreed to this plan but insisted that the device be used only in an emergency and after the completion of more animal experiments. Before long, Dr. Liotta had a calf that survived for 44 h while being totally supported by the TAH.
In April 1969, Cooley implanted the artificial heart in Haskell Karp, a 47-year-old man who could not have survived without the device [10]. After the implant, the patient was alive, awake, and in stable condition. The TAH kept him alive for 64 h, until a transplant could be performed. Unfortunately, immunosuppression was poorly understood at that time. Mr. Karp began receiving immune suppressants when the TAH was implanted. By the time of the transplant, his white blood count had fallen to below 2000 cells mc/L. He died of overwhelming sepsis 32 h after the transplant. Nevertheless, this first clinical TAH experience proved that a patient could be supported by a mechanical heart.
Despite its promise, the early era of heart transplantation soon ended in disappointment. By the early 1970s, most of the recipients had died of infection or organ rejection. For this reason, cardiac transplantation was almost universally abandoned for at least a decade. The US government redirected its research funds toward the development of an implantable LVAD, not as a bridge to transplantation but as a permanently implantable device. By that time, I was involved in heart surgery and MCS research at THI. After transplantation was renewed in the early 1980s, the pulsatile HeartMate implantable pneumatic LVAD was developed in our laboratory. In 1994, it became the first implantable pump to be approved for clinical use by the US Food and Drug Administration (FDA). The HeartMate vented electric (VE) LVAD (◘ Fig. 1.2) was implanted in 1991, and this untethered device was the first device that allowed patients to leave the hospital (◘ Fig. 1.3). As of early 2012, more than 10,000 HeartMate pumps had been implanted worldwide. However, they were too large to fit in smaller patients, including many women and children. Perhaps even more important, the durability of these pumps was limited.



Fig. 1.2
The HeartMate I LVAD

Fig. 1.3
First patient to have the HeartMate XVE implanted and the first patient to be discharged with an LVAD (Image reproduced with permission from Frazier [21]. Figure 1. Copyright Elsevier)
While the human body is at rest, a healthy heart beats 70 times a minute. This is equivalent to 4200 beats an hour and more than 100,000 beats a day. Therefore, the ideal circulatory support pump must be durable enough to beat more than 36 million times a year. Because the initial LVADs could function reliably for only about 2 years, long-term LVAD support would have necessitated frequent pump replacement. As a result, these devices were impractical as destination therapy and were practical only for bridging to transplantation. Therefore, the number of patients who could benefit from LVADs long term was limited to the number of available donor hearts – about 2400 in the USA and 3500 worldwide each year. Thus, LVAD technology would have no epidemiologic effect on the approximately 100,000 patients in the USA alone who faced premature death without a replacement heart. This caused Norman Shumway, a heart transplant pioneer at Stanford University, to comment during a nationally televised debate with Dr. Jarvik that bridging to transplantation would have no statistical benefit; it would simply result in a nightmare – further prolonging the waiting list for heart transplants.
Contemporaneous with what I referred to as “Shumway’s nightmare,” I began to further explore the use of implantable continuous-flow (CF) blood pumps, which would function without creating a pulse. When the idea of using implantable, long-term, nonpulsatile pumps was first proposed, it incurred severe criticism from the medical community. The concept gave rise to a debate about whether or not a pulse is necessary. However, the heart is the only organ that actually needs a pulse, which is required not for systole but for the diastolic blood flow that provides an energy source. If the heart fails, however, this becomes a moot point. The heart could potentially be unloaded by a CF pump, which would perhaps restore the normal Starling response. Heart surgeons of that era knew that patients who could not be weaned from CPB but who had a heartbeat could usually be maintained by a CPB flow of about 2 L/min; however, their heart could not compensate if they were separated from this support. As a preliminary experiment, in 1984, I had implanted a small CF pump supplied by Bio-Medicus, Inc. (currently Medtronic, Minneapolis, Minnesota, USA), in two pigs. In each case, the heart was fibrillated, but an adequate cardiac output was maintained by the CF pump in the left ventricle. I believed that such devices would offer much longer durability (potentially up to 20 years) than the flexing membrane in pulsatile pumps. In addition, because CF pumps were much smaller, they would fit a wider range of patient body sizes.
From the early 1980s, I was a clinical advocate of implantable long-term CF pumps. For this reason, at a 1986 conference I was approached independently by two medical engineers, Robert Jarvik and Richard Wampler, who asked me to test their respective designs for implantable CF blood pumps. At first, I was skeptical of these devices, but I thought that we should follow Claude Bernard’s advice to proceed with the experiment and let nature provide the answer [11].
The first of these cf-LVADs to be implanted clinically was the Hemopump (◘ Fig. 1.4), designed by Wampler. It was about the size of the eraser on a number 2 lead pencil and spun at up to 25,000 rpm. I was initially concerned that it might act like a kitchen blender and destroy the blood cells, but our laboratory showed that the device was safe in short-term experiments in calves. In 1988, we successfully implanted it in a 55-year-old man with heart failure (◘ Fig. 1.5), thereby rescuing him from certain death [12, 13]. This pump may be likened to the Wright brothers’ flying machine, which also was initially regarded as impractical; the Hemopump got pulseless blood flow technology “off the ground” as a feasible approach to temporary circulatory support. Later, the Hemopump was implanted numerous times, both in the USA and abroad, but insufficient funding was available to make the device comply with FDA requirements. Fortunately, in 1994, I was at a congress in Germany, where I encountered an old friend, Dr. Helmut Reul, of Aachen. He was a pioneering biomedical engineer who had trained in Houston. I encouraged him to look at the technology represented by the Hemopump, as I felt that it was an important step forward. Dr. Reul soon began developing this technology in Germany. His work resulted in the device currently known as the Impella LVAD for temporary support, which is currently approved for clinical use in both Europe and the USA.



Fig. 1.4
The Hemopump

Fig. 1.5
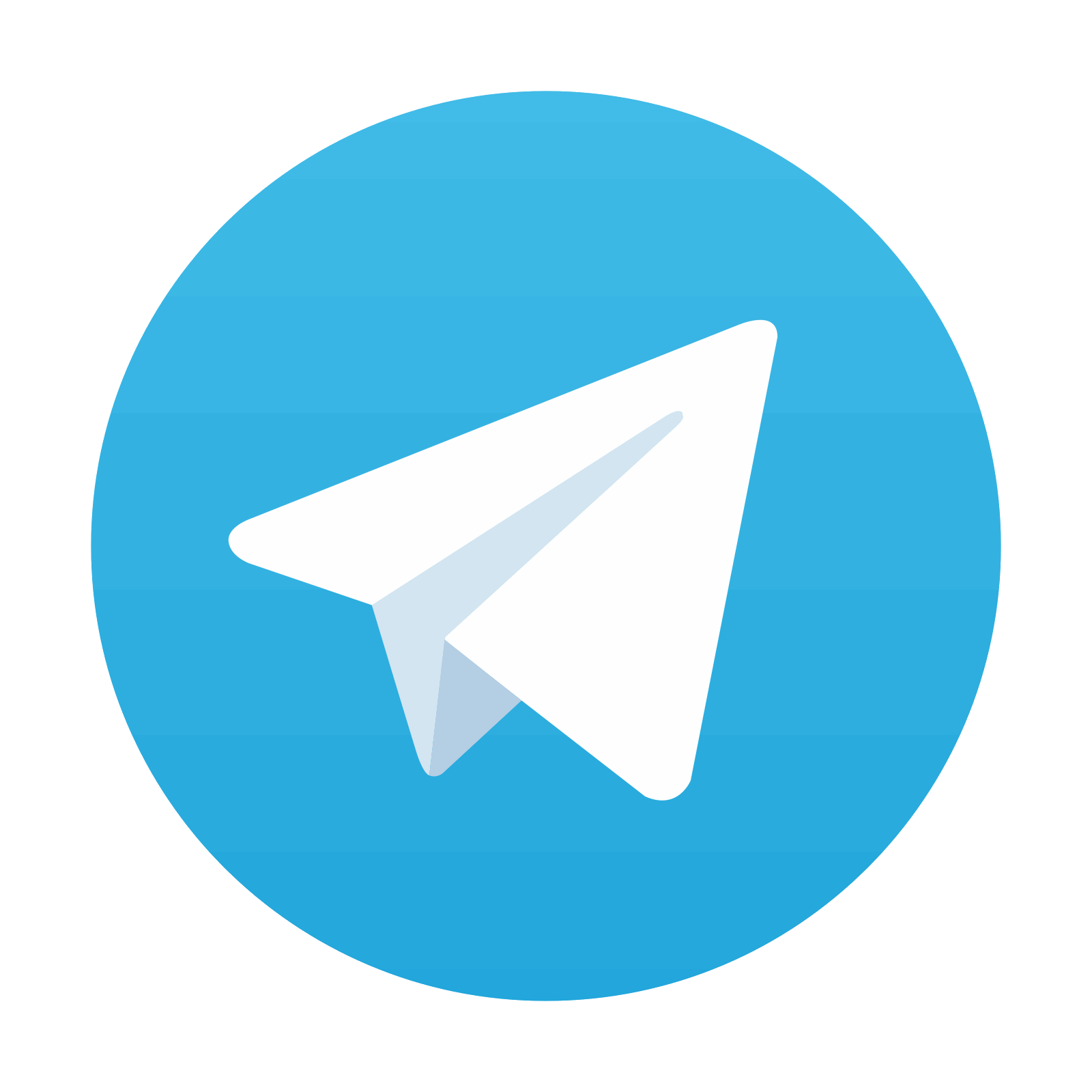
Dr. Richard Wampler (left), Dr. O. H. Frazier (right), and the first patient to receive a Hemopump (center) (Image reproduced with permission from Frazier [21]. Figure 2. Copyright Elsevier)
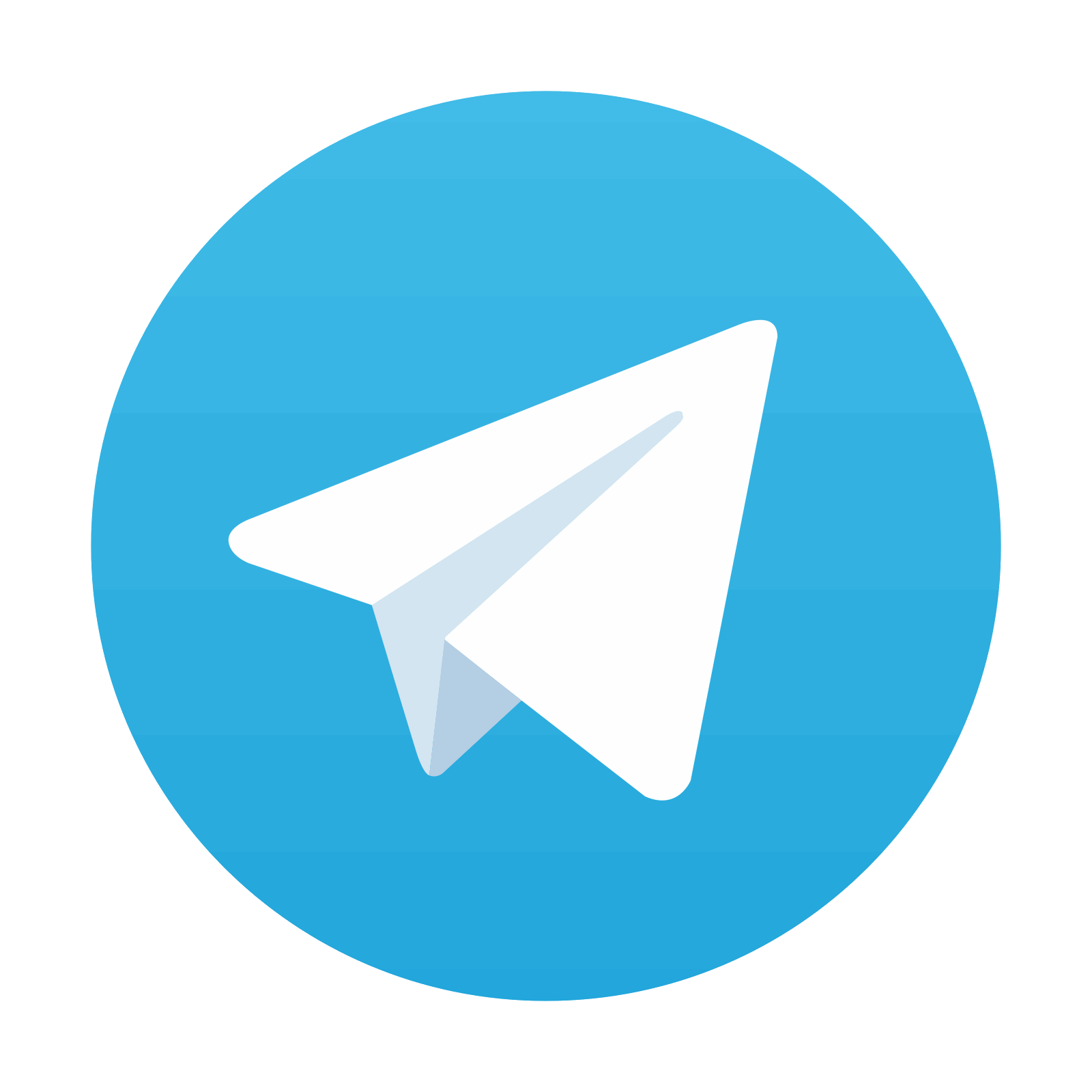
Stay updated, free articles. Join our Telegram channel

Full access? Get Clinical Tree
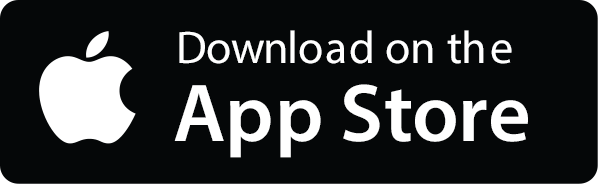
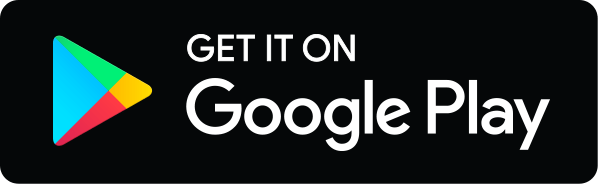
