The coronary vasculature may be artificially divided into 3 compartments: the epicardial vessels, the coronary microvasculature, and the venous system1 (Figure 11-1).
The epicardial coronary arteries (diameter >500 μm) are predominantly capacitance vessels and, in the normal state, offer little resistance to blood flow. The main epicardial coronary arteries are comprised of the right coronary artery (RCA), the circumflex artery, and the left anterior descending (LAD) artery. The RCA arises from the right aortic sinus, descending in the right atrioventricular groove, giving off a marginal branch. The RCA passes to the inferior surface of the heart and continues in the posterior interventricular groove as the posterior descending artery (PDA). The RCA may give off a further branch supplying the lateral left ventricular wall—the posterior left ventricular branch. The left coronary artery arises from the left aortic sinus as the left main stem, which divides into the circumflex artery and the LAD artery. The circumflex artery runs laterally in the left atrioventricular groove and gives off obtuse marginal braches. The LAD runs in the anterior interventricular groove to the apex of the heart. The LAD gives off septal perforators and diagonal branches.2 In approximately 70% of people, the RCA continues as the PDA (right-dominant circulation); in 10%, the circumflex artery forms the PDA (left-dominant circulation); and in 20%, the PDA is formed by an anastomosis of the circumflex artery and RCA (co-dominant circulation) (Figure 11-2).
Figure 11-2
Ex vivo arteriogram of a normal human heart demonstrating the normal coronary epicardial anatomy: RCA (red contour), LAD (green contour), and circumflex artery (blue contour). The extensive anastomotic communications of the coronary microvasculature are well visualized but are not demonstrated on standard invasive coronary angiography. (Used with permission from Fulton WF. Immersion Radiography of Injected Specimens. The British Journal of Radiology. 1963;36:685-688.)

Epicardial coronary blood flow occurs predominantly in diastole, due to the effect of systolic myocardial compression on the coronary microvasculature. The subendocardium is most susceptible to ischemia (in comparison to the subepicardial myocardium) due to the greater effect of left ventricular systolic compression and higher resting oxygen consumption. Myocardial oxygen extraction at rest is high (60%-80%); therefore, increased oxygen consumption during exercise is predominantly met by increases in myocardial blood flow rather than increased oxygen extraction.3 The coronary microvasculature is the predominant site of autoregulation of myocardial blood flow and resistance to coronary blood flow. Pre-arterioles (diameter 100-500 μm) are comprised of proximal and distal vessels, which are most responsive to changes in flow and pressure, respectively. Pre-arterioles regulate perfusion pressure into the subtended arteriolar compartment. Arterioles (diameter <100 μm) match myocardial blood supply and demand and are the predominant site of metabolic regulation of myocardial blood flow.3-5 In the healthy state, myocardial blood flow may be increased 4- to 5-fold.
Coronary artery disease (CAD) is a significant cause of morbidity and mortality, with an annual incidence of approximately 1% worldwide.6 Modifiable risk factors (eg, hypertension, dyslipidemia, tobacco smoking, sedentary lifestyle, type 2 diabetes mellitus) and nonmodifiable risk factors (eg, increasing age, male sex, family history of premature CAD) are well recognized, and primary and secondary prevention strategies are well established.6
Invasive coronary angiography is the reference standard test for the diagnosis of obstructive epicardial coronary atherosclerotic plaque in patients presenting with angina. However, up to 40% of patients presenting with angina have no evidence of obstructive epicardial disease on invasive coronary angiography.7 Anatomic and functional abnormalities in both the epicardial and microvascular coronary compartments may limit myocardial blood flow and result in angina. Therefore, there is increasing utilization of invasive diagnostic tests to assess coronary vascular function at the time of diagnostic coronary angiography. The coronary pressure wire and the intracoronary Doppler flow wire are currently the most established methods. In addition to functional assessment, adjunctive intracoronary imaging is also increasingly used to guide percutaneous coronary intervention (PCI), providing information on lesion and coronary stent characteristics. Intravascular ultrasound (IVUS) and optical coherence tomography (OCT) are the most commonly used intracoronary imaging tools. These functional and anatomic diagnostic tests for CAD will be considered in clinical context.
Invasive coronary angiography involves cannulation of an artery to gain access to the aortic root. This is predominantly performed via a radial artery and less commonly via a femoral artery, as radial access is associated with fewer periprocedural complications.8 Coronary guide catheters (typically 5-6 Fr) are manipulated to the aortic root, and the left and right coronary ostia are cannulated in turn. Iodinated contrast media is injected, and orthogonal x-ray projections are acquired to anatomically assess for epicardial CAD.
A 64-year-old man presented with an inferior ST-segment elevation myocardial infarction (Figure 11-3). Invasive coronary angiography demonstrated an occluded distal RCA with evidence of extensive thrombus (Figure 11-3A). The culprit stenosis has irregular edges, and there appears to be ulceration at the distal edge. Balloon angioplasty failed to achieve reperfusion, and therefore, aspiration thrombectomy was performed, resulting in reperfusion of the distal vessel (Figure 11-3B) and collection of a massive coronary thrombus (Figure 11-3D). Figure 11-3C demonstrates the appearance of the RCA after drug-eluting stent implantation.
Figure 11-3
(A) Occluded right coronary artery. Two coronary guidewires are in situ. The culprit lesion (yellow arrow) and distal thrombus (red arrows) are indicated. (B) Appearance following aspiration thrombectomy. The distal vessel is now visualized. (C) Appearance following coronary stent implantation. The stented segment is marked (red contour). (D) The aspirated coronary thrombus next to a coronary torque device (black arrow) is shown for scale. The white platelet-rich component (red arrow) and red erythrocyte-rich component (blue arrow) of the thrombus are marked.

A patient presented with stable angina after having undergone previous coronary artery bypass grafting (Figure 11-4). Invasive coronary angiography demonstrated a significant stenosis in the saphenous vein graft to the right PDA. The patient’s native RCA was occluded with the stump of the native vessel being visualized.
Figure 11-4
(A) Invasive coronary angiogram of the saphenous vein graft (red contour) to right posterior descending artery (yellow contour). There is a stenosis in the mid portion of the saphenous vein graft (blue arrow) as well as a stenosis before the insertion point of the graft (yellow arrow) onto the native vessel. (B) Native right coronary artery stump (yellow arrow), conus branch (red contour), and sternal wire (red arrow).

Revascularization for stable CAD is indicated to improve anginal symptoms.6 The “ischemia hypothesis” supposes that PCI may improve outcomes in patients with stable CAD and a moderate to large ischemic burden,9 and this hypothesis will be addressed by ongoing clinical studies.10
The role of PCI in the treatment of patients presenting with ST-segment elevation myocardial infarction is well established, with a target of <90 minutes from first medical contact to reperfusion.11
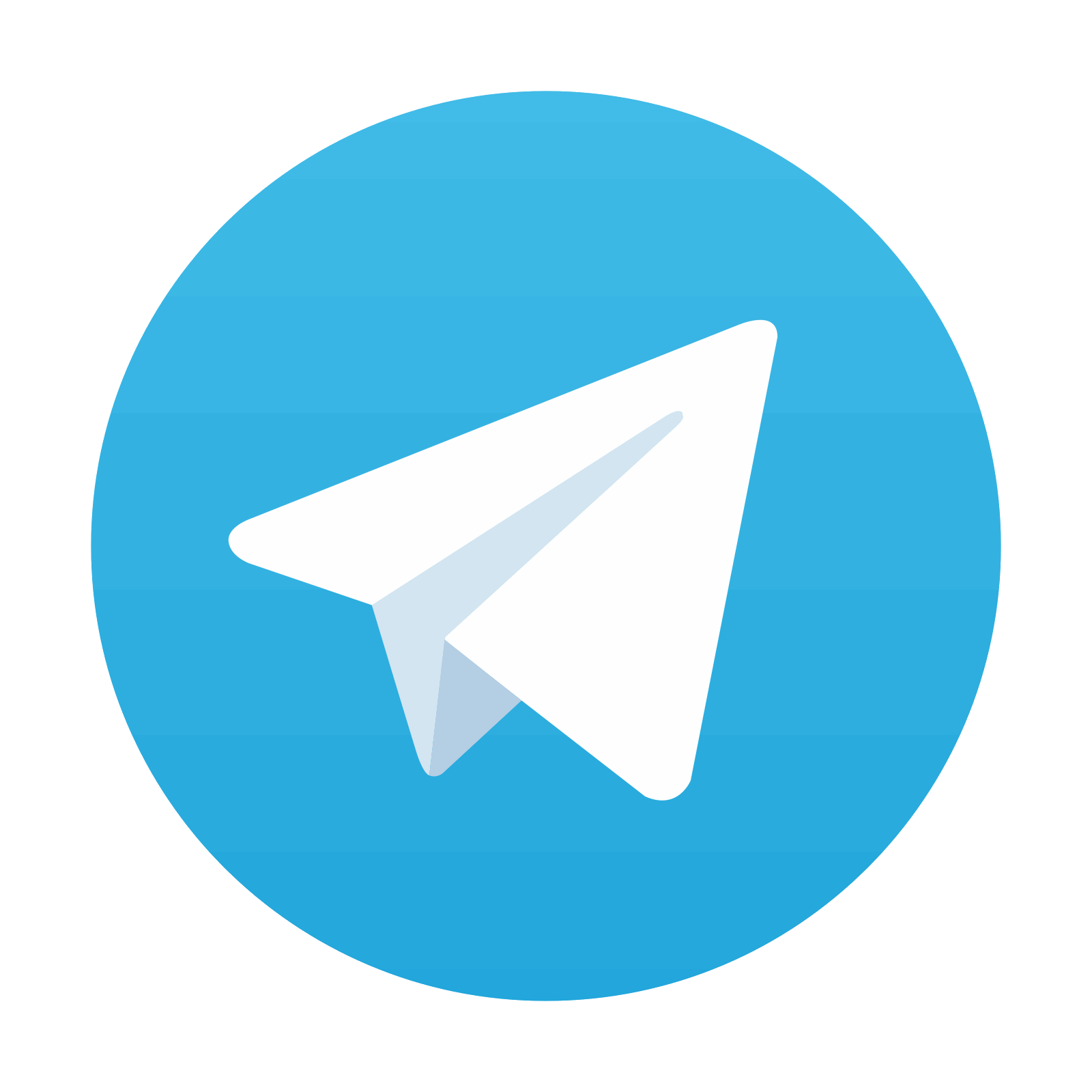
Stay updated, free articles. Join our Telegram channel

Full access? Get Clinical Tree
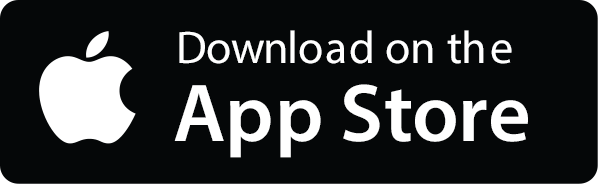
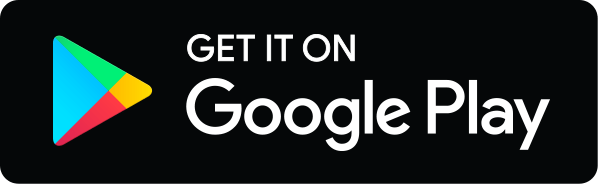