, Rohit Arora3, 4, Nicholas L. DePace5 and Aaron I. Vinik6
(1)
Autonomic Laboratory Department of Cardiology, Drexel University College of Medicine, Philadelphia, PA, USA
(2)
ANSAR Medical Technologies, Inc., Philadelphia, PA, USA
(3)
Department of Medicine, Captain James A. Lovell Federal Health Care Center, North Chicago, IL, USA
(4)
Department of Cardiology, The Chicago Medical School, North Chicago, IL, USA
(5)
Department of Cardiology, Hahnemann Hospital Drexel University College of Medicine, Philadelphia, PA, USA
(6)
Department of Medicine, Eastern Virginia Medical School Strelitz Diabetes Research Center, Norfolk, VA, USA
Introduction
The human body is a remarkable complexity of systems, whose functions are fully integrated to support life as we know it. Together, these systems enable the body to adapt to, and function normally in, a vast diversity of environments and over an extensive range of dysfunctional circumstances. One of the main systems responsible for this adaptability and capability is the autonomic nervous system (ANS) and the interplay between its two branches: the parasympathetic and sympathetic nervous systems (PSNS and SNS, respectively) [1–4].
Living things, including people, go to a considerable amount of trouble to stay alive. Some of the work and effort involved in staying alive is expended at the level of consciousness, but fortunately, much of it is carried out automatically under the direction of a great communication system of the body – the Autonomic Nervous System. Jay Tupperman, MD, Metabolic Physiology, 1968
The ANS is a regulatory branch of the nervous system. Portions of the ANS exist in the central as well as the peripheral nervous systems. Functions include regulation of blood pressure (BP), heart rate (HR), airway size and airflow to the lungs, digestive tract functions, sweat production, bladder control, sexual function, nervous system function (including depression and anxiety), gland and hormone function, and pupil size. The ANS acts through a balance of its own two components, the PSNS and SNS (P and S). Autonomic dysfunction results from an imbalance between the P and S components and may affect one or more systems of the body. Autonomic disorders may be congenital or acquired, and primary or secondary. If the P and S become unbalanced, a person may experience a variety of symptoms that are sometimes vague and, under therapy, often changes the organ system affected.
The P and S, like the rest of the nervous system, are plastic. They “remember” situations and “learn” about (adapt to) new situations. Injury or a disease causes an adaptation to a dysfunctional state. Therapy, treatment, or lifestyle modifications may be able to retrain the P and S to yet another new state either to support a cure, to minimize morbidity or mortality, or to minimize dosing in maintenance therapy. Therapy typically affects (directly or indirectly) the P or the S. Measurements of P and S responses to disease, and then to therapy, enables documentation of patient responses to both and titration of therapy specifically for the individual patient. Independent, simultaneous measures of P and S function offer quantitative documentation of clinical trending not available with current noninvasive measures of autonomic function (i.e., HR variability or beat-to-beat BP). This measurement is P&S monitoring.
The P and S branches work together to control and coordinate virtually all cells and systems within the body. The primary function of the two is to find a means of maintaining homeostasis and normal function, even in the face of disease and end-organ dysfunction [5]. This great compromise is achieved heartbeat by heartbeat and breath by breath. Therefore, measuring P and S activity provides additional information documenting an individual’s underlying physiology, enabling greater insight into disease processes, disorders, and patient responses to genetic predispositions, therapy, and lifestyle [6].
Old School
Most remember from medical school a few quaint facts about the ANS: that the SNS is considered the “fight or flight” system and the PSNS is considered the “rest and digest” system. The SNS is the adrenergic system communicating with end-organ tissue via noradrenaline or norepinephrine as its primary neurotransmitter. The PSNS is the cholinergic system, communicating with end-organ tissue via acetylcholine as its primary neurotransmitter. That in general, the SNS speeds up metabolism and the PSNS slows it down. And, of course, “point and shoot.”
Anatomy
It is true, in general, that the SNS is the adrenergic system. The exceptions to this are the sympathetic innervation of the eccrine sweat glands and some blood vessels which remain cholinergic. Below the level of the head, the SNS ganglia are relatively distant from the end-organs. Its synapses are on end-organs at the level of the sympathetic chain ganglia from which these nerves arise. The preganglionic sympathetic fibers project from the spine, between the thoracic and lumbar segments, and communicate with the postganglionic nerves with acetylcholine. Sympathetic nerves are efferent nerves, directly innervating all organs but the upper and lower gastrointestinal (GI) tract (see Table 1.1). The SNS is the reactionary nervous system responding based on metabolic thresholds set by the PSNS (Fig. 1.1).

Table 1.1
Normal or expected and abnormal results of P and S activation
Organ system, organ or tissue | Sympathetic activation | Associated SNS disorders | Parasympathetic activation | Associated PSNS disorders |
---|---|---|---|---|
Brain | Arousal | Hyperactivity | Induce sleep | Depression |
Hypothalamus | – | – | Activate | Growth and development |
Eyes | Pupil dilation | – | Pupil constriction | – |
Lachrymal | – | – | Tearing | – |
Salivary glands | – | – | Salivation | Sjögren’s disease |
Thyroid | Increase | Hyperactivity | Decrease | Hypoactivity |
Heart – inotropy | Increase | High BP | Decrease | Low BP |
Chronotropy | Increase | Tachycardia | Decrease | Bradycardia |
Lungs – bronchi | Increase = constriction | Increase = asthma, COPD | – | – |
Decrease = dilation | – | |||
Ventilation | – | – | Increase = slow breathing | Increase = hypoxia |
Decrease = fast breathing | Decrease = short of breath | |||
Upper GI | – | – | Increase = more motility | Increase = GERD (overactivity) |
Decrease = less Motility | Decrease = GERD (underactivity), gastroparesis | |||
Lower GI | – | Irritable bowel syndromea | Increase = more motility | Increase = diarrhea |
Decrease = less motility | Decrease = constipation | |||
Pancreas (islets of Langerhans) | Increase insulin | Shock or | Decrease | Diabetes |
Coma | Insulin | Mellitus | ||
Liver | Release glucose and nutrients | Hyperactivity | Store glucose and nutrients | Hypoactivity |
Splanchnic system | – | – | Increase = open | Hypovolemia |
Decrease = close | – | |||
Adrenal glands | Increase = release catecholamines | Increase = hyperactivity | – | – |
Decrease = store catecholamines | Decrease = hypoactivity | |||
Kidneys | Decrease volume | Dehydration | Increase volume | Hyperhydration |
Angiotensin–renin | Increase = more volume, thirsty | Increase = hypertension | – | – |
Decrease = less volume | Decrease = hypotension | |||
Bladder | Increase sphincter tone | Persistent full feeling | Decrease sphincter tone – voiding | Frequent urination |
Sex function – female | Orgasm | – | Vaginal lubrication | – |
Estrogen | Increase | ? | Decrease | Perimenopause |
Sex function – male | Ejaculation | Premature ejaculation, impotence | Erection | No ejaculation |
Testosterone | Increase | Rage | Decrease | Impotence |
Peripheral vasculature | Increase = vasodilation | Increase = hypertension | – | – |
Decrease = vasoconstriction | Decrease = hypotension | – | – | |
Sweat glands | Increase = sweating, reduce core temperature | Increase = hyperhidrosis | – | – |
Decrease = conserve core temperature | Decrease = anhidrosis | – | – |

Fig. 1.1
A diagrammatic representation of the autonomic nervous system (ANS) and its two branches, the parasympathetic and sympathetic nervous systems. Note that the ANS controls all other organs and precedes those organs. Since symptoms do not occur until end-organ dysfunction, autonomic dysfunction is asymptomatic
PSNS synapses tend to be near or in the organ of innervation. The thoracic and upper abdominal organs are innervated by parasympathetic nerves that form the vagus nerve (cranial nerve X) and arise from the upper medulla in the brainstem: nucleus tractus solitarius (afferent), dorsal nucleus of the vagus nerve (efferent), and nucleus ambiguous (efferent). The dorsal nucleus and nucleus ambiguous project efferent signals and the tractus receives afferent signals. PSNS nerves directly innervate all organs but blood vessels, bronchi, the adrenals, and sweat glands. The lower abdominal organs receive parasympathetic projections from sacral ganglia via sacral nerves 2 and 3.
In the head, cervical ganglia project the SNS into the brain, facial blood vessels, and pupils. The PSNS projections are carried in cranial nerves III, VII, and IX, innervating the pupils (CN III); lachrymal glands (CN VII), mucous glands of the nose and palate (CN VII), and submandibular gland (CN VIII); and the parotid glands (CN IX). Within the brain, the limbic system (cingulate gyrus), the amygdala, and basal ganglia communicate with the upper medullary parasympathetic nuclei, integrating the central nervous system cholinergic pathways. These pathways also affect the central serotonergic system. The reticular activating system (RAS), basal ganglia, and frontal cortex communicate, via the spinal cord, with the SNS integrating the catecholamine systems, including the serotonin, epinephrine, and norepinephrine pathways. The feedback center between the SNS and PSNS is the upper medullary brainstem.
Within the SNS, outside the brain, there are three main divisions: the alpha-adrenergic (or α-adrenergic), the beta-adrenergic (or β-adrenergic), and the dopaminergic systems. Of the α-adrenergics, there are three subdivisions, α1, α2, and α3, primarily innervating the vasculature. Of the β-adrenergics, there are four subdivisions, β1, β2, β3, and β4. The most well-known β-adrenergic systems are the β1- and β2-adrenergic receptors. The β1-receptors are primarily located on the heart. The β2-receptors are primarily located in the bronchi and secondarily located in the heart. The dopamine receptors are G protein-coupled receptors: D1, D2, and D3. The SNS regulates circulatory baroreceptor reflex (BRR) though sensors in the aorta, carotids, and renal arteries. The BRR in turn regulates peripheral vascular resistance and inotropic cardiac function (including strength of contraction) and thereby regulates BP. The SNS input via the atrioventricular node regulates cardiac chronotropy and thereby regulates HR. The SNS is involved with the arousal systems of the body and regulates bronchial diameter (but not ventilation) and sweating.
The angiotensin–renin system that controls blood volume is considered a system within the SNS, and blockade of the angiotensin–renin system causes dramatic reductions in the sympathetic measure (LFa [7]). It assists in the regulation of BP by regulating blood volume (see flow diagram, Fig. 1.2). As a result, angiotensin–renin agents (e.g., ACE inhibitors, ARBs, and renin blockers) all effect a reduction in systemic, sympathetic activity.


Fig. 1.2
The angiotensin–renin system control of BP regulation by regulating blood volume
Within the PSNS, outside the brain, there are also two major divisions: the vagal and the sacral. The principal neurotransmitter, acetylcholine, interacts with G protein-coupled muscarinic acetylcholine receptors M1–M5 and nicotinic ligand-gated ion channels (termed neuronal type I–III and muscle type IV).
Through the vagus nerve, the PSNS regulates HR. PSNS influence on BP is indirect through its feedback relationship with the SNS. The PSNS regulates ventilation by controlling diaphragm and intercostal muscle activity. The PSNS influences bronchial diameter through its feedback relationship with the SNS. Stretch receptors within the lungs send ventilatory information via the PSNS afferent neurons in the vagus nerve, which feeds back onto the sinoatrial node of the heart. In this way the PSNS assists in regulating HR. This regulation manifests as respiratory sinus arrhythmia (RSA). This is known as the cardiovagal effect. This, in healthier and younger individuals, is responsible for the increase in instantaneous HR (IHR) with inhalation and decrease in IHR with exhalation. In this way, RSA manifests autonomic function, and respiratory activity may be independently analyzed to isolate, and independently and simultaneously measure, parasympathetic activity as it influences cardiac function. This has been shown to represent PSNS function throughout the body [7]. The PSNS indirectly affects BP through the SNS. The PSNS mediates rest by slowing functions. Parasympathetic excess in the brain leads to depression and depression-related syndromes. The PSNS uniquely regulates the GI tract and the associated splanchnic systems.
Presynaptic myelinated axons are projected through cranial nerves or spinal nerves to synapses located in ganglia from which postsynaptic unmyelinated fibers are derived, which target the innervated organ. It is also important to realize that at first glance these systems appear to have opposing effects (see above). Simultaneous stimulation of both results can be synergistic. For example, stimulation of PSNS with SNS results in a greater increase in cardiac output. Sympathetic stimulation increases the ejection fraction and parasympathetic stimulation slows the heart, allowing increased efficiency of cardiac filling. As a result, baroreceptors perceive stretch in the aorta with increasing BP. They, in turn, send signals through the sensory component of the vagus nerve, terminating in the nucleus of the tractus solitarius in the medulla. From here, presynaptic sympathetic fibers project to the ventrolateral nucleus of the medulla and parasympathetic presynaptic neurons to nucleus ambiguous in the medulla. Coordinated firing of both arms is necessary to respond to changes in BP, and if damage occurs, this coordination is reset to accommodate new levels, which might be too high or too low for a particular individual. Thus, for any given situation, one needs to consider the system in its entirety and that damage to one or other component might not have as devastating an effect as damage to the whole system.
Biochemistry
The ANS communicates through two main neurotransmitters: acetylcholine and norepinephrine (noradrenaline for the British among you). Acetylcholine is the preganglionic neurotransmitter for both divisions of the ANS, stimulating the postganglionic P and S fibers in the autonomic ganglia. Acetylcholine is also the postganglionic neurotransmitter of parasympathetic neurons. The chemical structure and the process that produces acetylcholine are depicted in Fig. 1.3. Nerves that release acetylcholine are said to be cholinergic. At the effector organs, sympathetic ganglionic neurons release norepinephrine to act on adrenergic receptors, with the exception of the sweat glands and the adrenal medulla. The chemical structure and the process that produces norepinephrine are depicted in Fig. 1.4. Nerves that release norepinephrine (noradrenaline) are said to be adrenergic.



Fig. 1.3
The chemical structure and the process that produces, acetylcholine. Nerves that release acetylcholine are said to be cholinergic. Parasympathetic nerves are typically cholinergic

Fig. 1.4
The chemical structure and the process that produces norepinephrine (noradrenaline for the British among you). Nerves that release norepinephrine are said to be adrenergic or catecholaminergic. Sympathetic nerves are typically adrenergic
Parasympathetic postganglionic neurons release acetylcholine as the primary neurotransmitter to stimulate muscarinic receptors on target tissue (e.g., cardiac or smooth muscle or glands). At the adrenal cortex, there are no postsynaptic neurons. Instead, the presynaptic neurons release acetylcholine to act on nicotinic receptors which stimulate the adrenal medulla to release adrenaline (epinephrine) into the bloodstream. Adrenaline acts on adrenoceptors throughout the body, producing a widespread increase in sympathetic activity.
Most postganglionic sympathetic neurons release norepinephrine. The effects of norepinephrine on effector cells are mediated by two types of sympathetic (adrenergic) receptors: alpha- and beta-adrenergic receptors. Alpha-adrenergic receptors are distributed throughout the vasculature smooth muscle, stimulating vasoconstriction. Beta-adrenergic receptors are primarily distributed throughout the thorax. Beta-1 adrenergic receptors are primarily located on the heart, stimulating increased chronotropic or inotropic responses. Beta-2 adrenergic receptors are primarily located in the bronchi, stimulating bronchodilation. Norepinephrine stimulates alpha-receptors to dilate the pupil and beta-receptors to relax the bladder and the uterus and to stimulate the breakdown of glycogen to glucose in fat and liver cells.
Parasympathetic activity stimulates splanchnic activity and serotonin production, which aids in the rest and digest process. The chemical structure and the process that produces serotonin are depicted in Fig. 1.5. Sympathetic activity stimulates the renin–angiotensin–aldosterone system to aid in mediating BP through blood volume control in the stress response. The systemic, including sympathetic, interaction with the renin–angiotensin–aldosterone system is depicted in Fig. 1.6. Included in the catecholaminergic pathway (Fig. 1.4) is the dopamine. Deficiencies in the dopamine system are the primary indication in basal ganglia movement disorders such as Parkinson’s disease. Parkinson’s disease is known to involve the SNS, including the eventual denervation of the sympathetic input to the heart [8].



Fig. 1.5
The chemical structure and the process that produces serotonin. Parasympathetic activity stimulates splanchnic activity and serotonin production, which aids in the rest and digest process

Fig. 1.6
The systemic, including sympathetic, interaction with the renin–angiotensin–aldosterone system. Through central feedback centers, sympathetic activity works with the renin–angiotensin–aldosterone system to aid in mediating BP through blood volume control in the stress response. Blue and red dashed arrows indicate stimulatory or inhibitory signals, which is also indicated by the ±, respectively. In the tubule and collecting duct graphics, the gray dashed arrows indicate passive transport processes. The active transport processes are indicated by solid gray arrows. The other solid arrows either indicate a secretion from an organ (blue, with a starting spot) or a reaction (black). These two processes can be stimulated or inhibited by other factors (Reproduced from http://commons.wikimedia.org/wiki/File:Renin-angiotensin-aldosterone_system.png)
While acetylcholine and norepinephrine (noradrenaline) are the primary neurotransmitters of the ANS, other neurotransmitters, including the precursors of acetylcholine and norepinephrine, affect the P and S branches, respectively. These include dopamine, purines, pyrimidines, nitric oxide, glutamine, GABA, aldosterone, arginine vasopressin, and more. The specific activities of these neurotransmitters and neuromodulators may be implicated in specific diseases or disorders and offer more information and options for therapy. We refer you to the many scholarly texts for more information on these other neurotransmitters, e.g., [1, 6, 8, 9].
Physiology
Both ANS branches mediate quality of life functions such as eating and sleeping well, orthostasis, proper bladder and bowel function, temperature control, and proper sex function (“point and shoot”). Historically, autonomic study focused on systemic functions (e.g., HR, BP, sleep, thermoregulation, and sex function), but specific autonomic involvement was not considered, largely due to a lack of data resulting from a lack of easy assessment. Typical disorders classically attributed to autonomic dysfunction are dizziness, lightheadedness, vertigo, and hyperhidrosis [1, 3–5].
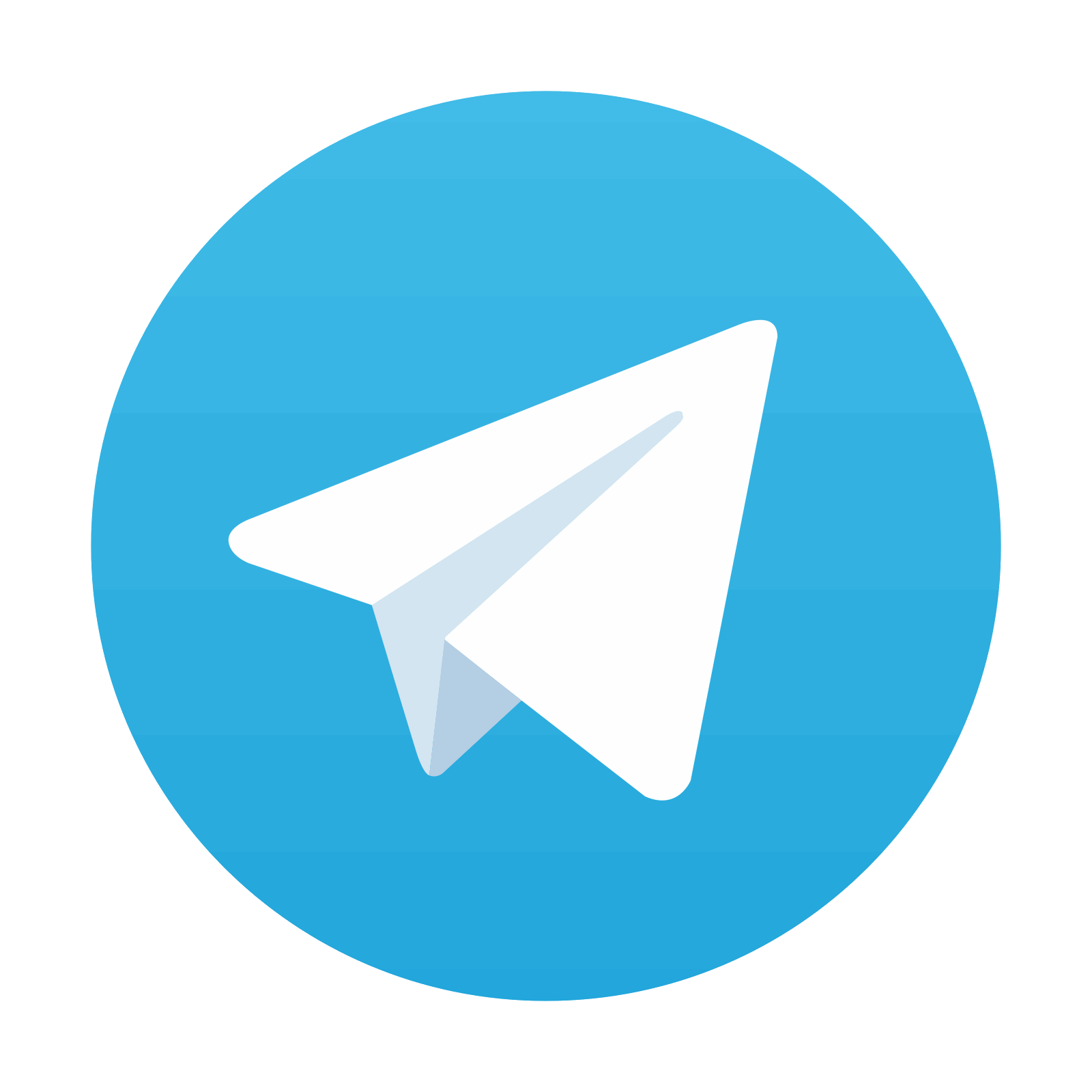
Stay updated, free articles. Join our Telegram channel

Full access? Get Clinical Tree
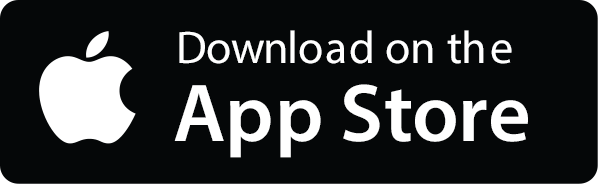
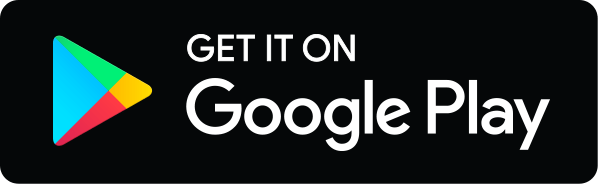