However, it is important to recognize that this knowledge and understanding evolved over the past two to three decades. Early forays into HF therapy targeted the hemodynamic derangement characteristically seen in severe HF patients [11]. Numerous observational studies were performed evaluating the acute hemodynamic effects of diuretics, hydralazine, nitrates, and other vasodilators. The era of randomized clinical trials in HF was ushered in with the publication of VHEFT-1 in 1986 [12], demonstrating a benefit of combination therapy with isosorbide dinitrate and hydralazine on outcomes in chronic HF. Subsequent clinical trials transitioned our focus from vasodilation to neurohormonal blockade and in particular to agents that inhibited the RAAS. Simultaneously, cautious case series and then larger trials demonstrated the profound benefits of beta-blockers on both mortality and meaningful salutary effects on ventricular remodeling [13–18].
The focus of newer HF trials remained primarily on RAAS antagonists [19–21] until further blockade of the RAAS proved less fruitful and, in certain cases, harmful [22]. Subgroup analyses from Val-HEFT and VALIANT showed increased adverse events in patients taking a combination of ACE inhibitor, angiotensin receptor blocker (ARB), and beta-blocker therapy [21, 23]. Moreover, trials of endothelin antagonists, cytokine antagonists, recombinant natriuretic peptide, centrally acting sympatholytics, and direct renin inhibitors on a background of ACE inhibition and beta blockade were also shown to have either neutral or harmful effects of treatment (Fig. 1.2) [22].
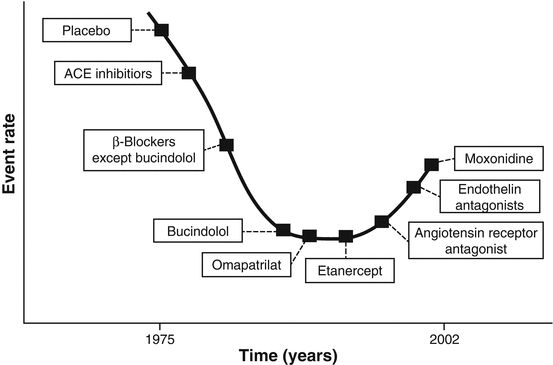
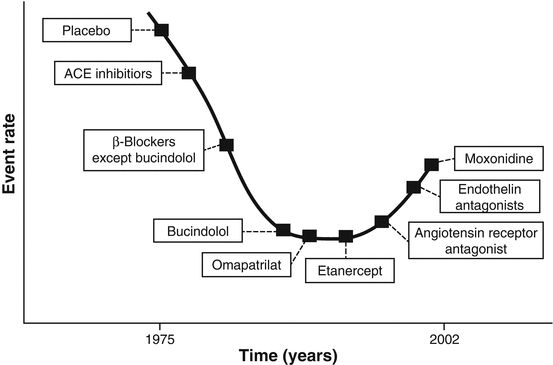
Fig. 1.2
Saturation of benefits with incremental neurohormonal blockade in chronic heart failure (Modified from: Mehra et al. [ 22])
After a decade of mostly negative trials, the PARADIGM-HF trial interrupted this trend in 2014. LCZ696, a novel combination compound of valsartan and sacubitril, a neprilysin inhibitor, led to substantial and significant reductions in mortality and multiple metrics of morbidity [24]. Publication of the PARADIGM-HF underscored the investigative shift away from pure RAAS inhibition and toward novel pathways, new drug delivery methodologies, or a focus on treating comorbidities that negatively affect HF progression. In particular, there has been great interest in drugs that influence cardiomyocyte energetics and/or metabolism and interventions such as gene therapy, stem cell therapy, noncoding RNAs, and ventricular assist devices as a potential route to recovery [25–27].
However, novel therapies are costly and sustain a long delay from conception to Phase III trials to regulatory approval. As an example, the initial studies evaluating neprilysin and RAAS blockage date back to the early 1990s, with publication of the first Phase III trial showing efficacy occurring in 2014 [24, 28]. Accordingly, there exists an increasing motivation to repurpose previously approved therapies for newer indications. Repurposing allows for faster drug delivery to the market and lower costs. In support of this concept, the American National Institutes of Health created an initiative to strengthen the partnership between academic institutions and industry which has already resulted in more than 50 intellectually protected but previously abandoned products becoming available for research [29].
Interestingly, HF as a field has a history of repurposing. In 2005, the AHEFT study reexamined the effect of the combination of hydralazine and isosorbide dinitrate in African-Americans already taking background ACE inhibitor and beta-blocker therapy [30]. AHEFT showed a substantial and sustained benefit on mortality in this subpopulation beyond that is seen in the original VHEFT trials. The drug combination was approved by the FDA in a new formulation in 2005 [31]. In 2007, Costanzo et al. published the UNLOAD study showing that ultrafiltration, shown to have less neurohormonal activation and more total body salt removal than diuretics alone, led to increased weight loss and decreased hospitalization rates as compared to standard therapy [32]. This study ushered in a series of trials examining the outcomes of ultrafiltration in HF patients without renal failure – a repurpose of the technology developed for dialysis. Indeed, ultrafiltration can be thought of as repurposing one of medicine’s oldest treatments: bloodletting or venesection. Further repurposing efforts have likewise focused on expanding application of previously approved therapies for severe HF, such as mineralocorticoid antagonists and cardiac resynchronization therapy, to those patients with milder HF symptoms [33–36].
The HF community naturally asked the next question: have we done enough to repurpose previously discovered treatments targeting the ANS? Initial enthusiasm for clonidine, a presynaptic alpha2 agonist that results in central inhibition of the SNS, waned with the publication of the MOXCON study showing harmful effects of its cousin, moxonidine, in HF patients [37, 38]. Animal studies of clenbuterol, a combined beta1 antagonist and beta2 agonist with anabolic characteristics, have been shown to exhibit beneficial effects on cardiac and myocyte remodeling as well as myocyte function [39, 40]. This preceded attempts to repurpose this drug from its initial indication for asthma toward a therapy for HF. In human HF, clenbuterol has largely been investigated in conjunction with ventricular assist devices for myocardial recovery, with promising preliminary results [40, 41]. However, trials have also shown detrimental effects on endurance and exercise duration in HF patients and use of this drug remains limited in HF [40, 42].
Subsequently, focus has shifted to non-pharmacologic, device-based strategies directed at the ANS. To date, investigation of device therapies in HF has targeted four ANS sites: (1) carotid baroreceptor stimulation, (2) vagal nerve stimulation, (3) spinal cord stimulation, and (4) renal sympathetic denervation [37, 43].
Baroreceptor activation therapy (BAT) involves the stimulation of one or both carotid sinuses resulting in inhibition of the sympathetic nervous system, predominantly studied in patients with resistant hypertension. In animal models of HF, BAT has effected improvements in LVEF, LV remodeling, and survival [37, 44]. Human studies are largely in their infancy with pilot data showing improvements in 6 min walk distance and reductions in sympathetic nervous system activation and NT proBNP levels [37]. These findings were recently confirmed in a multicenter, multinational randomized controlled trial [45]. Further trials of BAT in systolic and diastolic HF are planned; development and study of minimally invasive endovascular implantation techniques are scheduled [46].
Like BAT, vagal nerve stimulation (VNS) is performed via surgical implantation and has been used for epilepsy and depression treatment for decades [43]. More recently, this method has been repurposed for HF with animal studies showing improvement in LVEF, hemodynamics, arrhythmias, and survival [37, 43, 46]. Initial studies in human HF have shown improvements in walk distance and quality of life metrics with conflicting results in regard to cardiac remodeling [37, 47]. These promising results have led to the initiation of a large randomized clinical trial of VNS in HF patients [43]. In addition, minimally invasive VNS has shown potential in preclinical and pilot studies in both cardiac and noncardiac conditions [48, 49].
As with vagal nerve stimulation and BAT, spinal cord stimulation is surgically implanted and has been used for peripheral vascular disease, angina, and chronic pain [37]. Animal HF models have shown improved hemodynamics with decreased afterload, lower blood pressure, as well as improvement in LVEF and reduction in arrhythmias and levels of natriuretic peptides [37]. Small pilot studies in HF have suggested improved quality of life, symptoms, peak oxygen consumption, and conflicting results on LV remodeling [43, 50, 51].
The last of the four current treatments is renal sympathetic denervation (RSD), currently the only one addressed by minimally invasive, nonsurgical methods. As with BAT, RSD has been predominantly studied in resistant hypertension. The enthusiasm generated from the immense success of initial RSD trials (SIMPLICTY-1, SIMPLICITY-2) has been greatly curbed with the publication in 2014 of the negative results of the first blinded randomized control trial of RSD, SIMPLICTY-3 [52]. In HF, initial pilot and small randomized trial data have shown procedural safety as well as improvement in symptoms, LVEF, and natriuretic peptide levels with a trend toward a beneficial effect on HF hospitalizations [46]. Both animal and human data exist showing improved natriuresis, hemodynamics, and left ventricular functioning, diastolic dysfunction, as well as reduction in left ventricular hypertrophy, some of which appear to be independent of blood pressure effects [46, 53]. In addition, preliminary data also suggest potential positive effects of RSD on insulin resistance, glucose metabolism, arrhythmias, and obstructive sleep apnea, thereby targeting some of the comorbidities and sequelae of HF [53].
Preclinical and clinical data strongly support a definite pathophysiologic mechanism to validate the benefit of ANS modulation; preliminary data is intriguing. As newer technologies evolve with transition away from surgical implantation to more minimally invasive techniques, the improved safety profile could further tip the scales toward therapeutic benefit. As Dr. Braunwald fittingly quoted Winston Churchill in his call to arms in our war against heart failure, “Now, this is not the end. It is not even the beginning of the end. But it is, perhaps, the end of the beginning” [25]. Certainly, the utility of the current repurposed approach to the neural pathways is an exciting topic for this publication.
References
1.
3.
Braunwald E, Chidsey CA. The adrenergic nervous system in the control of the normal and failing heart. Proc R Soc Med. 1965;58:1063–6.PubMedPubMedCentral
4.
5.
Cohn JN, Levine TB, Olivari MT, et al. Plasma norepinephrine as a guide to prognosis in patients with chronic congestive heart failure. N Engl J Med. 1984;311:819–23.PubMedCrossRef
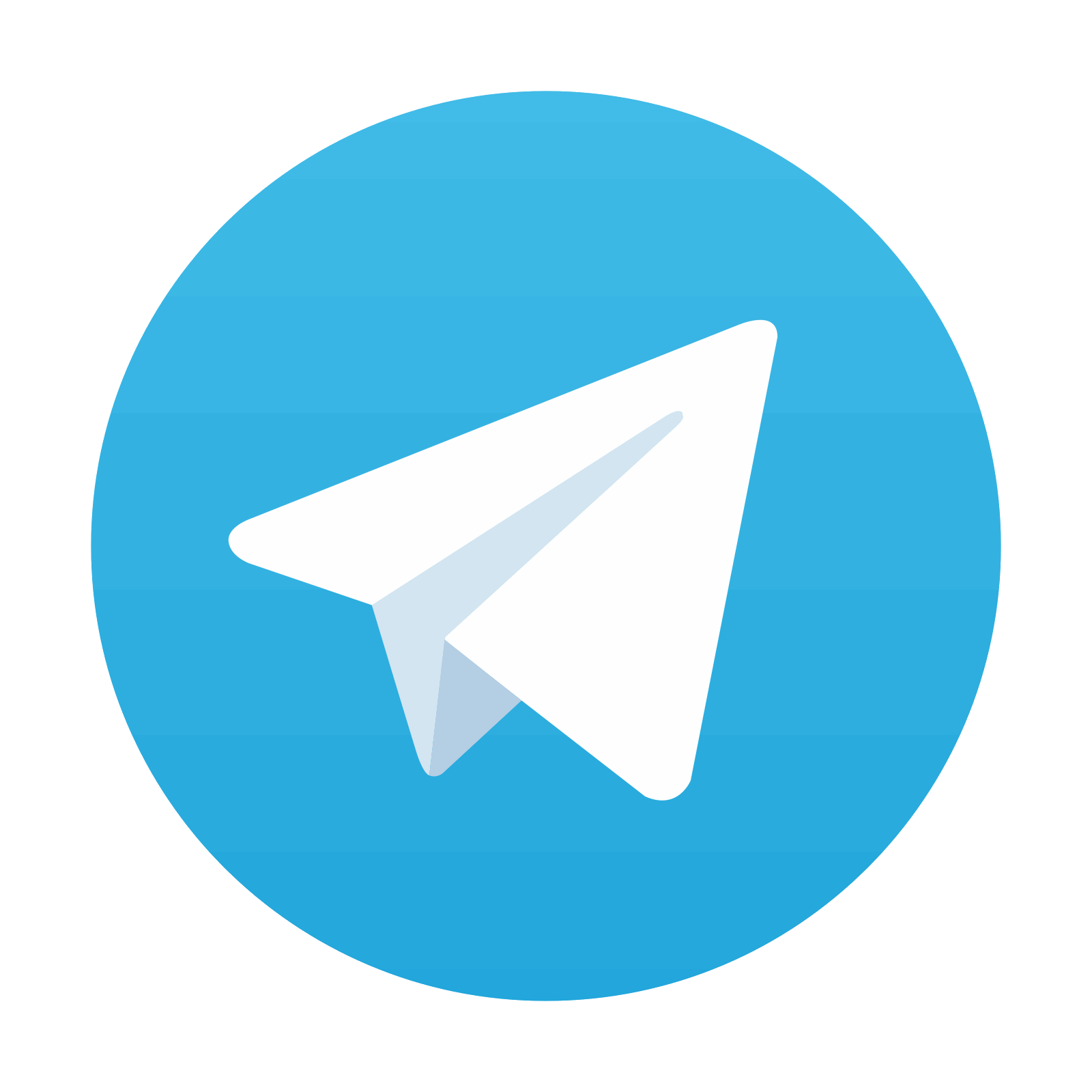
Stay updated, free articles. Join our Telegram channel

Full access? Get Clinical Tree
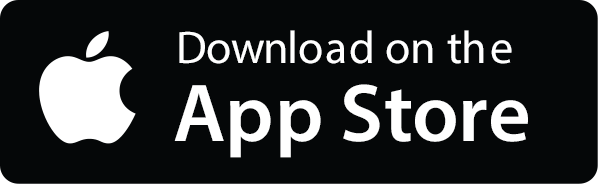
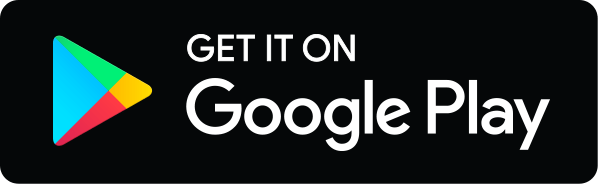