30 Intraoperative Neuromonitoring During Thoracic Spine and Spinal Cord Surgery Abstract Somatosensory evoked potentials (SSEPs) and motor evoked potentials (MEPs) are employed for spinal cord monitoring during thoracic spine and spinal surgery. SSEPs directly monitor dorsal column function and MEPs directly monitor pyramidal tract function. As spinal cord injury commonly affects both systems, concurrent monitoring of both modalities affords important redundancy while enabling detection of injury insolated to either system. SSEPs may be performed continually throughout surgery, but since each SSEP consists of the average of the brain’s response to several hundred stimuli acquired over several minutes, detection of injury by SSEPs is necessarily delayed. MEPs recorded from muscle require no averaging and results are instantaneous. However, since they are performed intermittently, the frequency and timing of MEP testing must be sufficient to detect injury in a timely manner. Anesthetic management is critical to SSEPs and MEP monitoring. The cortical component of SSEPs is suppressed by most common anesthetic agents. Small changes in anesthetic dose can result in large changes in SSEPs, so it is desirable that stable anesthesia be maintained, and necessary that changes be communicated. The same considerations apply to MEPs recorded from muscle. MEPs are often even more sensitive to anesthetic agents; total intravenous anesthetic is commonly recommended, although balanced anesthesia, including a low dose of volatile inhalational agent, has been reported to be similarly effective in most patients. Traditionally, monitoring has focused on “critical periods” during surgery, for example, following correction of a spinal deformity. It is now recognized that best practice often entails monitoring throughout the entire procedure. Keywords: intraoperative neuromonitoring, somatosensory evoked potentials, motor evoked potentials, M-waves, D-waves, anesthetic fade Clinical Pearls • Intraoperative neurophysiological monitoring using both motor evoked potentials (MEPs) and somatosensory evoked potentials (SSEPs) enables detection of injury restricted to either motor or sensory pathways. Since spinal cord injury during thoracic spine surgery commonly affects both systems, monitoring both provided added safety through redundancy. • SSEP monitoring should include both cortical and brainstem signals. Cortical SSEPs are the easier to record and less susceptible to interference, but are often affected by anesthetic agents. Brainstem SSEPs, although more difficult to record, are unaffected by anesthetics. • MEPs are safe. Tongue bites and patient movement are the primary risks. • MEPs should be recorded with sufficient frequency both to detect injury in a timely manner and also to allow for reassessment of the “baseline” in light of increasing suppression by anesthetics over time, even at constant doses. Intraoperative neurophysiological monitoring (IONM) serves to detect and to reduce the risk of neurological injury during spine surgery. Somatosensory evoked potential (SSEP) monitoring of spinal cord function was first introduced in the late 1970s.1 Its effectiveness was subsequently demonstrated in a study of 1,168 scoliosis surgeries at the Royal Orthopedic Hospital, where it was reported to have zero false negatives and a technical failure rate of only 2.2%. The authors suggested that SSEP monitoring was superior to, and should probably replace, the Stagnara wake-up test.2,3 The utility of SSEP monitoring was firmly established by the mid-1990s, when a large multicenter study revealed a nearly threefold reduction in incidence of severe postoperative neurological deficits following scoliosis surgery when IONM was employed.4 Although uncommon, false negatives did occur. This is not surprising, as SSEPs directly monitor the function of the dorsal column sensory pathways of the spinal cord, and serve only as surrogates for the integrity of other pathways. SSEPs are, in fact, generally effective surrogates for “global” cord function because injury due to blunt trauma, compression and ischemia, is usually widespread, affecting multiple pathways.5,6,7,8 Nonetheless, injury sparing the dorsal columns will go undetected by SSEP monitoring.9 SSEP monitoring can also fail for technical reasons related, for example, to interference from electrical noise and muscle activity, or to the effects of anesthetic agents; in some cases, baseline pathology may render SSEP monitoring impossible. Motor evoked potentials (MEPs) elicited by transcranial electrical simulation, initially described in 1980,10 are directly dependent on corticospinal tract integrity and are more direct monitors of motor function. MEP monitoring is widely employed, along with SSEP monitoring, during surgery that places the spinal cord at risk. The concurrent use of MEP and SSEP monitoring not only permits detection of injury restricted to either motor or somatosensory systems, but also provides an important level of added safety through redundancy. If, for example, MEPs are transiently abolished by a bolus of an anesthetic agent, or SSEPs become unmonitorable due to electrical noise or muscle artifact, monitoring can continue using the other, uncompromised, modality. Additionally, MEP and SSEP monitoring can be complementary, responding with differing sensitivities and speeds to changes in anesthetics and blood pressure, and to spinal cord injury. SSEPs are recorded at the scalp following peripheral nerve stimulation—usually the median or ulnar nerves in the upper extremity and tibial or common peroneal nerves in the lower extremity. Subdermal needle electrodes are commonly used for both stimulation and for recording. Subdermal corkscrew type electrodes or surface electroencephalography (EEG) electrodes, secured with collodion, may also be used for recording. Stick-on surface electrodes may be used for stimulation, but are, in general, less reliable. Median SSEPs are usually larger signals, and therefore are easier to record, than ulnar SSEPs, but ulnar SSEPs can provide information about injury below C6 that would not be detected by median SSEPs. They can also be more sensitive to brachial plexus stretch injuries related to patient positioning. Tibial nerve SSEPs are generally higher amplitude and more robust than peroneal SSEPs, and produce much less patient movement. Nonetheless, common peroneal SSEPs are useful in patients in whom tibal SSEPs are not monitorable, for example, due to peripheral neuropathy or ankle edema. Recording electrodes are placed at specific scalp locations, which correspond to the topography of the electrical fields generated by the somatosensory cortex. In addition to cortically generated signals, scalp electrodes also detect signals that are generated in the brainstem and conducted to the scalp through the cranial contents. SSEPs are recorded using differential amplifiers that amplify the voltage difference between two inputs. This allows for selective recording of tiny neurophysiological signals in the presence of electrical noise, such as power main frequency noise radiated by OR equipment (e.g., blood warmers, electrically powered tables and operating microscopes), by eliminating the noise signals seen equally by both amplifier inputs. Differential amplifiers also allows for selective recording of focally recorded, cortically generated, SSEPs by electronically subtracting the widely distributed brainstem signals that are equally detected in both input electrodes. SSEPs are tiny signals, 1 to 2 orders of magnitude smaller than the EEG; typically, responses to several hundred stimuli must be averaged over several minutes to record an SSEP waveform. When the SSEP signal is particularly small, or the electrical environment is noisy, more time is required. Both cortical and brainstem signals are used for SSEP monitoring. Both provide equivalent information regarding spinal cord integrity, but are affected differently by noise and anesthetic agents. Brainstem SSEP signals are resistant to anesthetic effects, but they are more easily contaminated by electrical noise and interference from muscle activity. They may also be difficult to record in patients with preexisting myelopathies. Cortical SSEPs are less vulnerable to noise contamination but they are more susceptible to attenuation by anesthetic drugs. Brainstem SSEPs can be recorded from any scalp electrode, using a neck electrode as an “inactive” reference. A common misconception is that the neck electrode detects cervical evoked potential signals; indeed, SSEPs recorded using a cervical reference are commonly, but incorrectly, termed “cervical” SSEPs. (A small signal is, in fact, generated by the gray matter of the cervical enlargement following upper extremity stimulation, but its contribution to SSEP monitoring is negligible.) Upper and lower extremity brainstem SSEPs appear quite similar, each consisting of a prominent positivity, reflecting activation of the caudal medial lemniscus, followed by a longer duration negative signal generated in the rostral brainstem ( Upper extremity cortical SSEPs ( Fig. 30.1 Brainstem SSEPs recorded following ulnar (upper trace) and tibial (lower trace) nerve stimulation. The positive (downward going) peaks denoted by arrows correspond to signals generated in the caudal portion of the medial lemniscus. These are generally the most reliable brainstem SSEP signals for monitoring. Cerv corresponds to a cervical reference electrode. Fig. 30.2 Cortical SSEP to left ulnar nerve stimulation (top trace) is recorded using a “scalp-to-scalp” bipolar derivation CP4 to CP3, to effectively electronically subtract the brainstem potential (CP3-Cerv) from the composite cortical plus brainstem potential (CP4-Cerv). Fig. 30.3 The scalp location of the cortical SSEP to tibial nerve stimulation varies considerably among normal individuals. At one extreme, shown in variant A, a prominent positive signal (arrow) is recorded at the vertex, with very little lateral spread. At the other extreme, shown in variant B, a prominent positivity (arrow) is recorded over the scalp ipsilateral to the stimulated limb, and very little is recorded at the vertex. Lower extremity cortical SSEPs are somewhat more complicated because their scalp topography differs considerably among normal individual. In some patients, lower extremity cortical SSEPs appear as a positivity at the scalp vertex, while in others they consist of a centropatietal positivity ipsilateral to the stimulated leg, accompanied by a contralateral negativity, and very little or nothing recorded from the vertex ( In addition, when possible, it is helpful to record from electrodes over Erb’s point and in the popliteal fossa to detect the SSEP signals distal to the region at risk of surgically related injury. Erb’s point and popliteal fossa recordings provide important controls, and help to distinguish loss of SSEPs due to a surgically related cause from loss due to failure of stimulation. The halogenated inhalational agents (e.g., isoflurane, sevoflurane, and desflurane) and nitrous oxide attenuate cortical SSEPs.12,13 Their combined effects are synergistic, that is, SSEP attenuation produced by nitrous oxide along with a halogenated agent is greater than the sum of their effects when used separately.13,14 Young children and infants can be especially sensitive.15 Importantly, not only is the degree of SSEP depression dose dependent, but the effect can be nonlinear, so that a small increase in dose can produce a large drop in cortical SSEP amplitude.16 Moreover, remarkable interpatient variability is encountered ( Brainstem SSEPs are essentially unaffected by clinically relevant concentrations of anesthetic agents. Neuromuscular blocking (NMB) agents do not affect the amplitudes or latencies of SSEPs, but they can substantially facilitate SSEP recording by eliminating contamination from electrical interference generated by muscle activity. Fig. 30.4 (a,b) SSEPs monitored in 2 patients receiving identical anesthesia (0.5% isoflurane, 50% nitrous oxide, propofol 35 ug/kg/min). Cortical SSEPs (CPz-Fpz and CPz-CP4 channels) are markedly attenuated in patient A, but are well preserved in patient B. Brainstem SSEPs are (arrows in Fpz-Cerv channel) are unaffected in both patients. In patient A, brainstem SSEPs are suitable for monitoring; in patient B, brainstem SSEPs are obscured by muscle artifact after 13:15. The standard “alarm criteria” for SSEP change is a 50% drop in amplitude or a 10% increase in latency compared to baseline.20 Spinal cord injury most commonly results in loss of SSEP amplitude and deterioration of SSEP waveform morphology, resulting respectively from conduction block and desynchronization of conduction in dorsal column axons. Latency changes are generally less prominent. Since cortical SSEPs are greatly influenced by anesthetic drugs, it is important that baselines be set after stable maintenance anesthesia is achieved, and readjusted following any changes. Further, over hours, cortical SSEPs often gradually increase in latency and decrease in amplitude.21 This effect, known as “anesthetic fade,” must be distinguished from SSEP changes due to clinically significant events. The standard “50/10” warning criteria are empirically based, and best serve as guides, rather than strict thresholds for detection of injury. Further, in practice, trial-to-trial variability and unavoidable contamination by noise and artifact can make 40 and 60% amplitude changes difficult to distinguish meaningfully. A better approach is for the surgeon to be notified when a new, sudden drop in amplitude or deterioration of wave-shape occurs that exceeds that expected based on prior signal variability observed during the case, even if the amplitude change appears less than 50%. This approach can permit more timely alerts and more accurate identification of the cause of SSEP changes.22 MEPs are monitored by stimulating the motor cortex and recording from either the spinal cord or from muscle. A single, brief, transcranial electrical stimulus produces a volley of pyramidal tract activity—a prominent D-wave resulting from direct activation of pyramidal cell axons followed by a series of smaller I-waves produced indirectly through trans-synaptic activation of pyramidal cells by cortical interneurons.23 A single stimulus is usually insufficient to elicit a response from muscle (M-wave) under general anesthesia. Rather, M-waves are generated using trains of several stimuli, one to several milliseconds apart. Each stimulus in the train produces a D-wave and perhaps several I-waves. Together, they summate temporally at alpha motor neurons in the lumbar and cervical enlargements of the spinal cord, causing the motor neurons to fire and generate an M-wave ( Commonly monitored arm and leg muscles contain several hundred overlapping regions, each 5 to 10 mm in diameter, innervated by a single spinal cord alpha motor neuron. An alpha motor neuron along with the family of muscle fiber it innervates is known as a motor unit. The M-wave is a composite of action potentials generated by activated motor units with muscle fibers close to recording electrodes. An M-wave thus reflects contributions from only a small percentage of the total number of motor units in a given muscle.26
30.1 Introduction
30.2 Somatosensory Evoked Potentials
30.2.1 Brainstem SSEPs
Fig. 30.1). They differ principally in latency following stimulation, reflecting the greater length of sensory pathways from the leg.
30.2.2 Cortical SSEPs
Fig. 30.2) appear as a negative signal detected by an electrode over the contralateral centroparietal scalp, at CP3 or CP4 scalp locations11 referred to an electrode elsewhere on the scalp to remove noise and subcortically generated SSEP signals. (Throughout this chapter, CP3 and CP4 refer to left and right centroparietal locations, C3 and C4 are slightly anterior to CP3 and CP4, and C1 and C2 are mesial to C3 and C4. CPz is a midline position just posterior to the scalp vertex. Fpz a frontopolar scalp location. These positions are defined precisely in the American Clinical Neurophysiology Society electrode nomenclature guideline.11) The scalp region where the cortical SSEP is largest can be small, and careful electrode placement is necessary. Several channels, using different electrode pairs, may be used and the channel producing the best recording selected for monitoring.
Fig. 30.3). In most patients, lower extremity cortical SSEP scalp topography lies somewhere between these two extremes. Accordingly, it is essential that at least two different channels be devoted to detecting lower extremity cortical SSEPs; the channel proving the most robust recording is selected for monitoring.
30.2.3 Anesthetic Effects
Fig. 30.4). Although most commonly used intravenous agents (i.e., propofol, benzodiazepines, opioids) also have some depressant effects on cortical SSEPs, these are relatively minor.12,17,18 Dexmedetomidine does not appear to depress cortical SSEPs.19
30.2.4 Interpretation
30.3 Motor Evoked Potentials
Fig. 30.5).24 M-wave recording can be further enhanced by delivery of two trains, separated by typically 10 or 20 ms. The first train likely acts through segmental and suprasegmental polysynaptic facilitatory mechanisms to reduce post-synaptic membrane polarization, preparing spinal cord alpha motor neurons to more readily reach threshold and fire in response to the second train (
Fig. 30.6).25
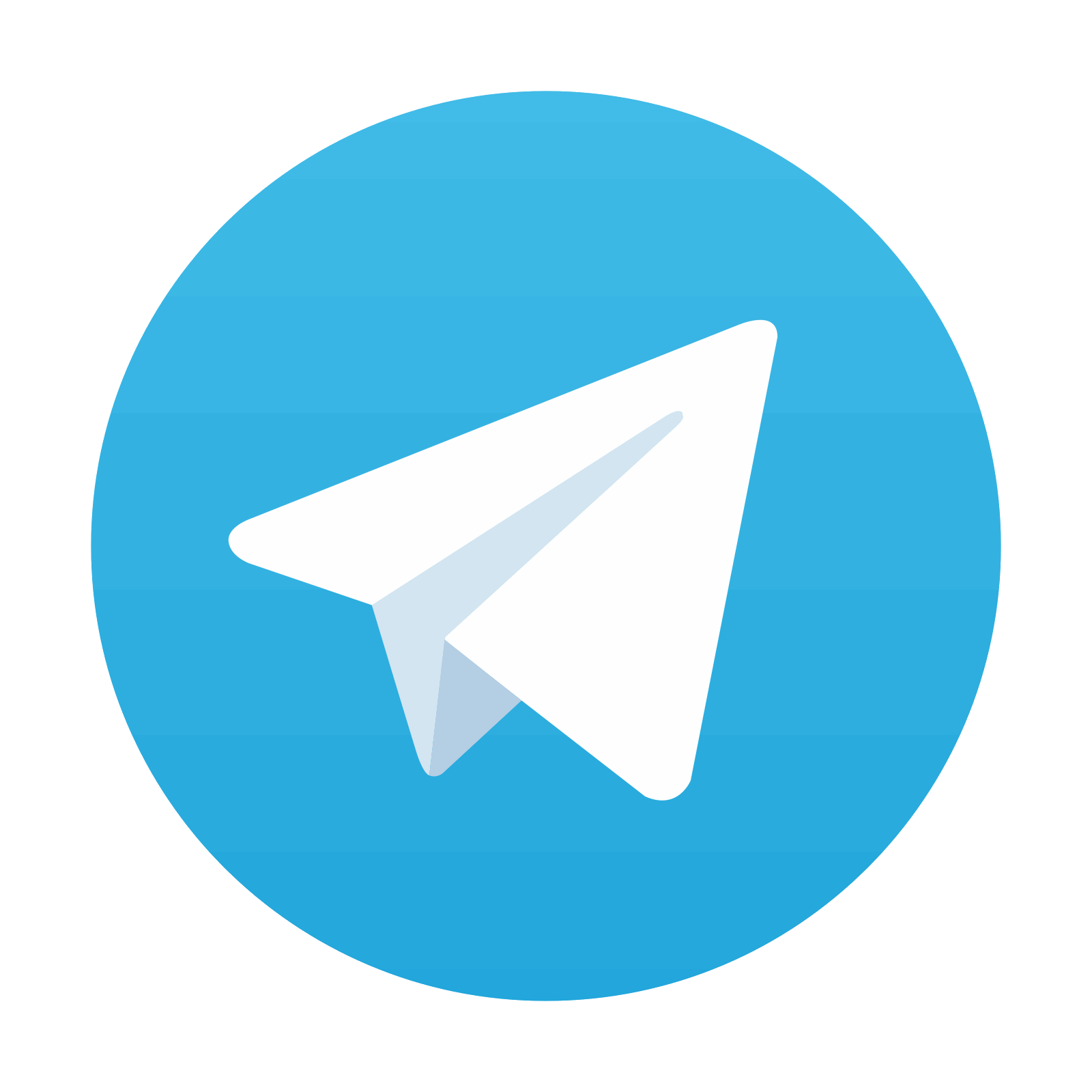
Stay updated, free articles. Join our Telegram channel

Full access? Get Clinical Tree
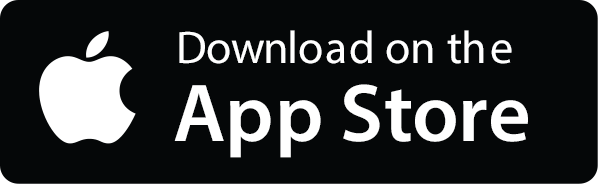
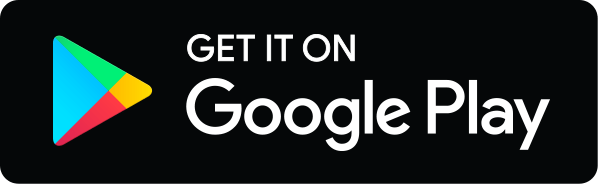