
Introduction
The management of cerebrovascular disease has often lagged behind the advances made in the management of cardiovascular disease. However those same technological advances in cardiovascular devices have facilitated the development of dedicated neurovascular devices, which have resulted in a narrowing of the treatment gap. Still there remain major differences primarily due to anatomical and physiological differences between the coronary and cerebral vasculatures.
Cerebrovascular Disease
Epidemiology
There are approximately 800,000 strokes each year in the United States. Of these, more than 83% to 85% are ischemic strokes; the remaining 15% to 17% of strokes are hemorrhagic. Of the former, 70% are large cerebral vessel occlusions and the remainder are so-called small vessel strokes (i.e., lacunar strokes). Spontaneous intracerebral hemorrhage (ICH), otherwise known as parenchymal hemorrhage, accounts for roughly two thirds of hemorrhagic strokes while spontaneous (nontraumatic) subarachnoid hemorrhage (SAH) accounts for the remainder and is caused by ruptured cerebral aneurysms. Stroke mortality has been declining due to advances in medical treatment and prevention and stroke currently ranks as the fourth leading cause of death in the United States, although it remains as the second leading cause worldwide. However, stroke remains the leading cause of adult disability in the United States.
What makes stroke unique, as compared with coronary artery disease (CAD) for example, is that it can be caused by a multitude of different pathological processes. Unlike acute myocardial infarction, which is overwhelmingly caused by ruptured coronary artery atherosclerotic plaques, stroke can be ischemic or hemorrhagic. Ischemic stroke can be caused by cardiac embolism (20%), cervical-cranial large vessel atherosclerosis (20%), lipohyalinosis-associated lacunar stroke (25%-30%), unknown causes (25%-30%), or a variety of rare causes (5%) (e.g., arterial dissection, vasculitis, migraine, hypercoagulable states, mitochondrial encephalopathies, etc.). Coronary disease and stroke share similar risk factors but hypertension stands out as the major risk factor. Hypertension is the major cause of parenchymal (nonaneurysmal) ICH as well as lacunar strokes. Populations at increased risk for ischemic stroke include African-Americans, patients with diabetes mellitus, men, and the elderly.
Clinical Manifestations
The clinical manifestations of stroke are highly varied and can range from asymptomatic to catastrophic. The exact manifestation is dependent on the location of the stroke, the volume of the brain affected, the rapidity of insult onset, the underlying health of the brain, the adequacy of brain collaterals in the case of ischemic injury, patient age, as well as a multitude of systemic, serological, and genetic factors. Generally speaking, stroke consists of the painless loss of neurological function except in the case of aneurysmal SAH, which is classically associated with an instantaneous and severe headache. Parenchymal ICH is associated with headache in about 40% to 50% of patients and the headache tends to be progressive in onset. Both ICH and SAH can be associated with nausea and vomiting along with focal neurological dysfunction. The typical clinical manifestations of ischemic stroke of the anterior circulation (i.e., the carotid artery territory) include unilateral motor and sensory dysfunction and cognitive dysfunction, with or without visual loss. The cognitive dysfunction can consist of confusion but more commonly consists of aphasia (a disturbance of language) if the dominant (usually left) hemisphere is affected. If the nondominant (usually right) hemisphere is affected cognitive dysfunction may manifest with visual-spatial deficits and hemi-neglect. Alteration of consciousness is atypical in anterior circulation strokes unless the stroke is massive and if found early on suggests that an ICH is the cause rather than ischemia. Posterior circulation (i.e., vertebrobasilar territory) strokes manifest with crossed sensory-motor deficits typically associated with diplopia, severe dysarthria, gait imbalance, ataxia, and vertigo. Profound and deep alteration of consciousness (i.e., stupor or coma) is much more likely than in anterior circulation strokes.
The majority of large ischemic strokes affect the middle cerebral artery (MCA) territory for several reasons. The bilateral MCAs supply the majority of the cerebral hemispheres and are effectively the terminations of the ICAs, which carry 80% of cerebral blood flow. Additionally, the cervical ICAs are the most common site of extracranial cerebral atherosclerosis.
Transient neurological deficits may also occur and can herald a permanent event. These transient ischemic attacks (TIAs) are clinically defined by complete resolution of the neurological deficits within 24 hours; longer events are classified as strokes. Modern imaging (i.e., magnetic resonance diffusion weighted imaging), however, has shown that events lasting hours are very often associated with permanent injury. Most true TIAs will last 5 minutes to a couple of hours. These events are most often associated with large vessel processes such as left atrial thrombi in atrial fibrillation, cervical internal carotid artery stenosis, or intracranial stenosis. The other etiologies described above may all manifest with TIA, including ICH (rarely). Therefore a TIA is a true medical emergency and requires the same thorough evaluation that a patient presenting with stroke would receive.
Diagnostic Evaluation
The diagnostic evaluation of the patient presenting with cerebrovascular disease is aimed at limiting the potential for permanent neurological injury. As can be surmised from the above discussion of the heterogeneous pathogenic mechanisms and varied clinical manifestations of stroke, a simple cookbook or algorithmic approach to all stroke patients is not feasible. However, there are many published guidelines that can facilitate the process. Generally speaking, the first step, after the basic ABC’s of resuscitation and neurological examination, is to differentiate an ischemic event from a hemorrhagic event. This is essential because one may mimic the other and the major and most dreaded side effect of all therapy for ischemia is ICH and vice versa. To accomplish this, a noncontrast computerized tomography (CT) scan of the brain should be performed to evaluate the brain parenchyma and its surroundings. Magnetic resonance imaging (MRI) has much greater sensitivity and specificity than CT but is time consuming and, except in some research institutions, is inadequate in the ultra-early phases of ICH. An evaluation of the cerebral vasculature should also be performed evaluating the entirety of the cerebral vascular tree, from aortic arch to the intracranial vessels. Both CT- and MRI-based noninvasive angiographic techniques (i.e., CTA and MRA) are available and have their benefits and drawbacks. Catheter cerebral angiography with digital subtraction (DSA) remains the gold standard for evaluating the vasculature. A cardiac evaluation (electrocardiography, echocardiography) along with a variety of laboratory studies may also be warranted.
The Cerebral Vasculature
The brain and cerebral circulation have several unique characteristics and are histologically different from other vessels in the body. Shortly after penetrating the skull base (~1 cm) the cerebral arteries lose the external elastic lamina and the tunica muscularis and adventitia thin considerably. As a consequence they become significantly more fragile and prone to injury during interventional procedures. Furthermore, after penetrating the dura matter the arteries enter the subarachnoid space that overlies the surface of the brain. Any injury that results in vessel rupture or perforation (e.g., dissection) can lead to catastrophy because the resultant SAH and/or ICH can result in a rapid and marked elevation of intracranial pressure (ICP) leading to either herniation or reduction/cessation of cerebral blood flow. The elevated ICP and its consequences are difficult to manage pharmacologically and sometimes require emergent neurosurgical decompression. Another important consideration is that embolization or occlusion of distal branches or perforators, even if nearly microscopic, can result in major disability. Finally, the cerebral vessels are also extremely tortuous and prone to vasospasm. For example, the internal carotid arteries (ICAs) have 180-degree turns in their cavernous segments. The proximal segments of the intracranial ICA run through the densest bone in the human body, the petrous bone, and are subsequently anchored within the cavernous sinus and the layers of the leathery dura matter. These factors, combined with their fragility, make the navigation of endovascular devices intracranially particularly difficult and potentially hazardous.
The brain is supplied blood by the paired ICA and vertebral arteries (VAs). The ICAs are branches of the common carotid artery and have no extracranial branches. Their first important intracranial branch is the ophthalmic artery. Shortly thereafter they (variable) give off the posterior communicating artery (PCom), which is the major anterior-posterior circulation collateral. They then give off the very important anterior choroidal artery before dividing into the MCA and anterior cerebral arteries (ACAs) ( Figure 25-1 ). The VAs are the first branches of the subclavian arteries and have many muscular branches in the neck as well as collaterals to the spinal cord. They enter the foramen magnum and join together to form the single basilar artery (BA) after giving off the (usually) large posterior inferior cerebellar artery. The BA has numerous perforators and branches supplying the pons, midbrain, and remainder of the cerebellum ( Figure 25-1 ). The BA then divides into the bilateral posterior cerebral arteries (PCAs), which supply the occipital lobes. The PCom joins the ipsilateral PCA and in combination with the bilateral ICA, bilateral ACA, and single anterior communicating artery (ACom) complete the Circle of Willis. The Circle is an inherent source of collaterals and (when present) can completely supply CBF to the territory of an occluded ICA or BA. Unfortunately, a complete Circle exists in only 25% to 40% of humans.

There are essential perforating arteries that emanate from the MCA, BA, and PCA trunks ( Figure 25-1 ). These vessels supply critical structures and, although small (50-200 µm), their occlusion can cause major and disabling neurological deficits. These vessels are at risk of occlusion during intracranial interventions, especially if they were the etiology of the presenting symptoms. Furthermore, they are particularly vulnerable to wire perforation. They arise from the dorsal (superiorly in anterior-posterior view) aspect of the MCA and (posteriorly in anterior-posterior view) BA.
Acute Ischemic Stroke Treatment
Current acute stroke therapies include intravenous (IV) tissue plasminogen activator (tPA), which is effective in both small and large vessel strokes and endovascular therapy (EVT), which is used for large vessel strokes. The former was approved in 1996 by the Food and Drug Administration (FDA) based mainly upon the results of the National Institute of Neurologic Disorders and Stroke (NINDS) study. More than a decade later, on average, only 5% to 10% of patients with ischemic stroke receive IV-tPA treatment. The major limiting factors are the strict criteria for the administration of IV-tPA, in particular the narrow time window for administration of only 3 hours from clear symptom onset. Furthermore, IV-tPA is poorly effective at recanalizing larger vessels. The middle cerebral artery (MCA) recanalization rate is approximately 30% while the larger ICA is successfully recanalized in <10% of cases. Consequently, EVT has been studied as an adjunctive/alternative treatment for large vessel acute ischemic stroke. Endovascular therapy (AKA Intraarterial thrombolysis [IAT]) is not an FDA-approved treatment. The only FDA-approved treatment for acute ischemic stroke (IS) remains IV-tPA.
The indications for EVT are evolving and since there is no approved EVT for stroke, there is a great deal of variability between practitioners in the field with regards to the indications. In general, patients with acute IS presenting less than 4.5 hours meeting criteria should be offered IV-tPA, and EVT should be offered to all others, and to those who refuse IV-tPA. It is important to note that treatment with IV-tPA does not reduce stroke mortality and patients with large vessel occlusions, large thrombus burden (i.e., thrombi longer than 8 mm), and those with more severe strokes respond less well to IV thrombolysis. For these patients EVT may be considered as an (unproven) alternative to IV thrombolysis. In one observational study of 112 patients with hyperdense middle cerebral artery (MCA) sign, half of whom received IV-tPA and the other EVT, favorable outcome was doubled and risk of death reduced by two thirds in patients treated with EVT.
The traditional time window for EVT is up to 6 hours for thrombolysis and 8 hours for mechanical embolectomy. The duration of ischemia is a leading predictor of neurological outcome, but with modern penumbral imaging selection of patients for EVT may be time-independent (see further on).
Patients must also have a significant clinical deficit that warrants intervention since mild deficits (i.e., National Institutes of Health Stroke Score [NIHSS] <4) are less likely to be associated with a visible proximal large arterial occlusion; such milder strokes have an excellent prognosis on average even without treatment. On the other end of the spectrum patients with severe strokes (NIHSS >20) generally do not benefit as much from treatment. Multimodal imaging techniques such as perfusion imaging may help select patients with major deficits who have small infarct cores and large penumbral zones who may still benefit from treatment. A recent prospective cohort study (Diffusion and Perfusion Imaging Evaluation for Understanding Stroke Evolution Study 2 [DEFUSE 2]) tested whether an MRI perfusion-based definition of target mismatch (TMM) could appropriately detect who would benefit from EVT within 12 hours of stroke onset. Of the 99 patients with perfusion imaging, 78 had a TMM, and 42 of whom were treated beyond the 6-hour time window. With recanalization and a TMM there was an odds ratio (OR) of 8.5 for good neurological outcomes in patients treated >6 hours along with a 2.9 OR for those treated <6 hours, compared with those without a TMM. Importantly, no reperfusion led to infarct growth and patients without a TMM did not benefit from recanalization (OR 0.2; p = 0.004). Unfortunately there is a paucity of level 1 evidence on the use of and parameters for defining penumbra with these techniques. An alternative is to select patients using the Alberta Stroke Program Early CT score (ASPECTS): a 10-point scale with 10 representing a normal CT and 0 a complete infarct of the entire MCA territory. A baseline ASPECTS score of ≥8 is an excellent predictor of clinical response to treatment.
The contraindications for IAT are generally any that would increase the risk of ICH. A history of spontaneous ICH or an untreated ruptured aneurysm or arteriovenous malformation are contraindications to thrombolysis although mechanical embolectomy may be performed in selected patients. A history of dementia, unless mild, should be a contraindication as those patients have a low probability of recovering. Relative contraindications are active anticoagulation with any class of anticoagulant, including antiplatelet agents; one small study has shown that mechanical embolectomy may be safe.
Techniques
Intraarterial Thrombolysis
A coordinated team approach is essential to the efficient, rapid, and safe implementation of endovascular therapy. Multiple concurrent events must be coordinated and everyone on the team should know in advance what their duties are. For example, while one is prepping and draping, someone else is readying the thrombolytic agents, while another is manging the medical needs of the patient. A cart containing all of the stroke specific devices should be brought into the suite if necessary to avoid delays searching for devices. Although practices vary, an 8 Fr sheath should be inserted into the femoral artery (a radial approach is feasible but has its own challenges, especially if a balloon occlusion guide catheter is to be used). Alternatively a 6 Fr sheath can be inserted. Ideally a 6 Fr guide catheter should then be quickly tracked into the vessel of interest. Although arch variants (e.g., common origin of the innominate and left common carotid artery or a bovine arch) are not uncommon (25%-30% of patient) and may make selective cannulation difficult, taking time to perform an arch angiogram can waste precious minutes. In practice most patients will have a CTA performed during the initial assessment and all such scans should always include the aortic arch and great vessels that can be of great help in planning for unusual anatomy or ostructions.
For anterior circulation strokes a common carotid artery (CCA) injection should be performed, and then if safe to do so a selective internal carotid artery (ICA) injection should be performed. For posterior circulation (i.e., vertebrobasilar [VB]) strokes, the dominant VA (generally the left, which is easier to cannulate) should be gently injected. All angiography should be performed in standard biplanar views (AP and lateral) using digital subtraction angiography (DSA). It is important to use a large field of view to visualize the entire inner table of the skull. Angiography should include the entirety of the arterial, capillary, and venous phases. Besides the identification of the occlusion and contraindications to thrombolysis (e.g., arteriovenous malformation) an assessment of collateral blood flow is essential since the presence of robust collaterals is a marker for clinical benefit and their absence is a harbinger of poor outcomes and probable futility. Full angiography of the other cerebral vessels is greatly discouraged especially if non-invasive imaging (i.e., CTA or MRA) is available and can be performed after recanalization is achieved, if necessary.
Any unnecessary delay in initiation of therapy should be avoided but if there are no collaterals seen on the ipsilateral injection it is reasonable to quickly inject the other vessels because a complete absence of collaterals is a grave prognostic sign and recanalization therapy should be reconsidered.
The periprocedural use of antithrombotics is variable and only one study (PROACT I) has studied this aspect, although it was in the setting of intra-arterial thrombolysis with pro-urokinase. In that trial, a high-dose heparin regimen was associated with excessive risk of ICH and the low-dose regimen (2000 U heparin bolus followed by 500 U/hr up to 4 hours) was found to be safer and has effectively been adopted by many in the field.
Stable access to the symptomatic vessel was one of the most important factors that determined procedural success but advancements in catheter design and manufacturing have made this less of an issue. Still sheath and guide catheter choice remains important. In most patients with straight cervical vessels a a short sheath and a 6 Fr neuroguide catheter (e.g., Envoy XB, Cordis Inc., Miami, Florida) advanced into the distal cervical ICA or V2 segment of the VA will provide adequate support for most therapeutic devices. In cases with severely tortuous great vessels or steeply angulated aortic arches, a modified approach utilizing a long 6-8 Fr sheath with the tip in the distal common carotid or subclavian arteries may be necessary for stable access. The newer generation of highly deliverable guide catheters such as the Neuron Max (Penumbra Inc., Alameda, California) provide stable access while being highly deliverable even intracranially.
The next step is to advance a microcatheter to the site of occlusion. This is most commonly performed over a soft-tipped hydrophillic 0.014-inch wire. Wire advancement and placement is one of the riskier aspects of neuro-interventions due to the aforementioned fragility of the cerebral vessels. Great care and advanced knowledge of the anatomy and variations are neceesary. The wire tip must not cannulate the small perforators arising from the superior MCA or posterior BA walls to avoid perforation or occlusion. The occlusion should be crossed with the wire with careful attention to the course of the tip while avoiding any tip deflection. The catheter can then be advanced into or past the thrombus. In most cases mechanical embolectomy will be performed, therefore the catheter needs to be advanced at least 2-3 cm distal to the distal most aspect of the thrombus. This can be gleaned from careful angiography noting the retrograde pial collateral flow, which will often reach the distal aspect of the occlusion or ideally from the preprocedure CTA. Some operators like to perform microcatheter angiography to document the exact location of the occlusion. This has been associated with an increased risk of ICH and may waste precious time that could be better used to achieve recanalization.
Some operators will then infuse thrombolytic agents distal to the clot. This has fallen out of the favor in the modern era of mechanical embolectomy but is an option in selected individuals with distal occlusions in which mechanical approaches may not be feasible. There is a great variability in the thrombolytic agents used for IAT. The agents that have been used include t-PA, streptokinase, retiplase, urokinase, as well as recombinant pro-urokinase (rpro-UK). Streptokinase is no longer used due to an excessive risk of hemorrhage. The PROACT II trial studied a fixed dose infusion of rpro-UK over 2 hours directly into the MCA; unfortunately while the trial was positive the clinical benefit in PROACT II was not profound. Even though the initiation of recanalization therapy in PROACT II was approximately within 5.3 hours from stroke onset, recanalization occurred well past the 6 hour window in many patients due to the mandate for a 2-hour infusion. Most operators, including the author, advocate a more rapid infusion of thrombolytics to be given in aliquots every 5-10 minutes. The author also advocates for an adjustment of the dose of thrombolytic so as to use the lowest effective dose to decrease the risk of ICH which has been associated with thromboytic dosage.
The following factors are associated with a higher risk of ICH therefore warranting lower doses of thrombolytics: older age especially >80 years old, presenting blood pressure >185/110, hyperglycemia, long duration of ischemia >4-6 hours, the presence of early infarct signs on pretreatment CT or MRI, and the presence of any other confounders such as the infusion of GPIIb/IIIa inhibitors. The lowest possible dose of all antithrombotic and fibribolytic agents should be used to the decrease the risk of ICH. Generally thrombolytics are reserved for those with distal thrombi not amenable to mechanical embolectomy devices or those who fail such interventions. Maneuvers such as wire manipulation of the thrombus have been described but are mostly ineffective and there are no prospective data supporting their use as they were prohibited in PROACT II.
Combination treatment with thrombolytics and GPIIb/IIIa antagonists has some potential advantages and has been described in small series. Anecdotally the combination may be most effective in cases of atherosclerotic occlusions, thrombosis complicating endovascular procedures or cases of artery to artery embolism due to endothelial injury (e.g., dissection, ruptured plaque, etc.). GPIIb/IIIa antagonists may have a role if emergency stent implantation is necessary. The use of these agents is very poorly studied and has been associated with ICH; furthermore there is no indication for continuous infusion as is the practice in acute myocardial infarction due to a very high rate of intracranial hemorrhage.
Combined IV/IA Thrombolysis
Intravenous tPA can be rapidly initiated without the need to wait for the EVT team or vascular lab to be available. Therefore an approach of IV thrombolysis followed by IAT has been described and studied in the IMS (Interventional Management of Stroke) trials. IMS I (a phase I feasibility and safety trial) combined IV and IA tPA and the follow-up IMS II added the MicroLys US infusion catheter (EKOS Inc., Bothell, Washington) to the combined IV/IA approach. In the IMS II treatment arm the ORs of attaining an mRS ≤2 were 1.74 and 2.82 compared with tPA and placebo-treated subjects, respectively.
The IMS III trial was a randomized controlled trial that enrolled 656 patients (out of a planned 900) treated within 3 hours of stroke onset with NIHSS ≥10 who were randomized to receive IV-tPA alone versus IV-tPA followed by EVT. The latter consisted largely of IA-tPA (80%) followed in order of frequency by the Merci Retriever (Stryker Inc., Kalamazoo, Michigan) (29%), and Penumbra (Penumbra Inc., Alameda, California) (16%), EKOS (6.6%), and Solitaire (Covidien/EV3 Inc.) (1.5%). The study was stopped prematurely for reasons of futility without any safety concerns. Analysis of enrolled patients did not show a significant difference in good outcomes between the IV only versus IV/EVT group, although trends in favor of the combined approach were noted in patients presenting with NIHSS >20. Symptomatic ICH rates and mortality did not differ between groups, indicating that the combined approach is as safe as IV-tPA alone. There was a strong correlation between recanalization success and outcomes. The limitations of IA-tPA and first-generation embolectomy devices were highlighted by the fact that in the endovascular therapy arm there was only a 44% thrombolysis in cerebral infarction (TICI) 2b/3 rate. Furthermore, initiation of EVT was generally very slow with a mean IV-tPA to groin puncture time of 82 min. These findings underscore the critical importance of complete and rapid recanalization. IMS III did not require proof of vascular occlusion at enrollment but in a prespecified subgroup analysis of patients with baseline CTA confirming a large vessel occlusion, there was a 6% higher rate of good outcomes in favor of the combined treatment, particularly in patients with ICA occlusion (23% in the IV/IA group vs. 5% in the IV-only group; p = 0.14).
At this time therefore there are no data to support a combined IV/IA strategy in IV-tPA eligible patients; however, in IMS III, patients were randomized to EVT before completion of the IV infusion so those results may not be strictly applicable to failed IV-tPA patients. To complicate matters there is no consensus on what constitutes IV-tPA “failure.” There are some data that recanalization, if it occurs, will occur within 1 hour of tPA infusion, therefore it is reasonable to consider EVT if patients do not recanalize or improve clinically within 1 hour of receiving IV-tPA.
Mechanical Embolectomy
Despite the fact that thrombolysis, both IV and IA, has been proven effective, it has significant limitations. The speed of recanalization and recanalization efficacy are the primary limitations. Pharmacological thrombolysis is based on the assumption that all thrombi/emboli are similar and are equally amenable to thrombolysis. In reality thrombi/emboli may be composed of different components (platelets, fibrin, cholesterol debris, etc.) and are of widely varied sizes and volumes. Furthermore thrombolysis may be contraindicated under certain circumstances due to an increased risk of systemic bleeding (e.g., gastrointestinal)) or intracerebral hemorrhage (e.g., head trauma). Mechanical embolectomy removes many of these limitations due to the lack of a systemic and persistent pharmacological effect. Anecdotal reports using a variety of devices and snares designed for foreign body removal were first reported in the late 1990s. Since that time dedicated devices have been developed and tested. The major advantage of this approach is the potential to achieve recanalization in a few minutes rather than 1 to 2 hours or longer. There are some theoretical disadvantages primarily an increased risk of vascular injury and dissection.
The technique of mechanical embolectomy varies depending on the device being used. Currently, the stent-trievers are the dominant devices and their use is markedly simpler than the older (Merci Retriever and Penumbra) devices. The approach is exactly like the IAT approach with two major differences. The first is that mechanical embolectomy is most effective with proximal flow arrest and aspiration. Therefore once the site of occlusion has been confirmed angiographically the 5 Fr diagnostic catheter or 6 Fr guide catheter are exchanged for an 8 Fr or 9 Fr balloon occlusion guide catheter (e.g., Merci). The lesion is crossed with the microcatheter and microwire but device-specific microcatheters must be used. The wire is removed and then the embolectomy device is delivered to the site of occlusion with about half of the device deployed distal to the thrombus and the remainder within the thrombus ( Figure 25-2 ). Angiographic confirmation of appropriate device deployment is then performed, which should show restoration of antegrade flow through the occluded segment as the tines of the stent push the thrombus aside. The device is left deployed for 3 to 10 minutes. Then with the balloon on the guide catheter inflated to occlude antegrade flow, the device is slowly withdrawn (while still deployed) while aspiration on the central lumen of the balloon guide catheter is performed. This creates retrograde flow in the parent vessel and facilitates thrombus extraction. The device can be reprepped and utilized for more passes as needed.

Balloon Angioplasty and Stenting
In several large Japanese series angioplasty has been reported to be very effective with recanalization rates near 90%. Those series have also reported lower rates of ICH compared with IAT (3% vs. >10%). These results may not be generalizable since intracranial atherosclerosis is the leading cause of ischemic stroke in Japanese and Asian populations and angioplasty may be particularly effective, as it is in the coronary arteries, under such circumstances. Still angioplasty has sometimes been effective even with non-atherosclerotic occlusions and is generally performed with gentle balloon inflation using an undersized coronary balloon. The author recommends angioplasty in cases of likely thrombotic occlusion (e.g., the presence of calcification at the site of occlusion, especially in patients of African-American or Asian descent without atrial fibrillation); this approach is anecdotal and it needs to be validated prospectively.
Stenting has also been reported to be highly effective particularly in cases of cervical internal carotid artery atherosclerotic occlusion. There has only been one prospective study of stenting in AIS in which 20 patients were treated with a self-expanding stent. That study demonstrated a TIMI 2/3 recanalization rate of 100% with a 5% sICH rate. Sixty percent of the patients had a good neurological outcome. As with angioplasty, stenting may be especially effective for cervical ICA atherosclerotic occlusions; the major drawback is the increased risk of ICH due to the need for dual antiplatelet therapy. The benefits of successful stenting include the possibility of improving flow rapidly even if the angiographic result is less than optimal. Another potential benefit is the definitive treatment of the underlying causative lesion and prevention of acute or subacute stroke recurrence. The drawbacks of emergent intracranial stenting include vessel injury, especially if the stent is oversized. Since most AIS patients will not be taking dual antiplatelet agents if stents are implanted GPIIb/IIIa receptor antagonists may be necessary but there are only anecdotal reports of their use in this setting.
It is worth re-emphasizing that stroke is heterogeneous and it is the author’s belief that treatment should be tailored to the needs of every individual, for example, intracranial stenting in an elderly patient with tortuous and heavily calcified vessels may be associated with a high likelihood of complications and primary angioplasty or thrombolysis may be safer approaches. The goal of rapid recanalization must not come at cost of ICH. The technique of intracranial stenting is discussed in the section below on Intracranial Angioplasty and Stenting.
Clinical Outcomes
Although there have been numerous series of IAT reported they shared in common only two qualities, they were a series of patients who had ischemic stroke and who were treated with intra-arterially delivered thrombolytics. They differed greatly in patient selection, duration of ischemia, occlusion location, stroke etiology, the endovascular approaches, and the pharmacological agents used. Individually most of these series suggested that IAT is effective at recanalization and that patients derived clinical benefit. Unfortunately a meta-analysis suggested there was no net benefit with pharmacological IAT.
Only one randomized trial of IAT has been completed and published, the Prolyse in Acute Cerebral Thromboembolism (PROACT) II trial. PROACT II evaluated the recanalization and clinical efficacy of rpro-UK in acute ischemic stroke. In that trial patients who had MCA occlusion of <6 hours duration, without CT evidence of infarct greater than one third of the MCA territory, were randomized to an infusion directly into the MCA of a fixed dose (9 mg) of rpro-UK over 2 hours or placebo. Mechanical clot disruption was not permitted. All patients received 2000 units of unfractionated heparin followed by 500 U/hr for a total of 4 hours. Treatment was associated with a 15% absolute benefit (58% relative benefit) in the 90 day modified Rankin Scale (p = 0.04). In the treatment group the TIMI 2 or 3 recanalization rate was 66% at 2 hours (vs. 18% for the placebo group); unfortunately the TIMI 3 rate was only 19% in the treatment arm.
In PROACT II the treatment group symptomatic ICH risk was 10% but only 2% in the control patients. This symptomatic ICH rate compared favorably with the rates in the major IV-tPA trials (6% in the NINDS trial, 9% in ECASS II, and 7% in ATLANTIS). Despite the positive results of the PROACT II trial the FDA did not grant approval, so IA thrombolysis remains an investigational treatment. Intra-arterial thrombolysis had become the standard of care at many academic medical centers until the advent of the newer mechanical embolectomy devices.
A second randomized trial of IAT was the Japanese Middle Cerebral Artery Embolism Local Fibrinolytic Intervention Trial (MELT) of intra-arterial urokinase for <6 hour MCA occlusion. It was stopped after enrolling 114 patients because of IV-tPA approval in Japan. The primary endpoint of mRS ≤2 was not significantly different and the rate of sICH was 9%. A preplanned secondary analysis showed that recovery to normal or near normal (mRS ≤1) was significantly higher in the treatment group (42.1% vs. 22.8%; p = 0.045).
The accepted time window for the initiation of IAT has been 6 hours from stroke onset in the anterior (ICA and MCA) circulation. Anecdotal reports have suggested that the time window in the VB circulation may be longer. Some investigators have treated patients including those with anterior circulation strokes well beyond the traditional time windows, suggesting that time is not the absolute determinant of EVT success. In one series of 55 consecutive EVT patients selected by perfusion imaging with a mean NIHSS of 19.7 ± 5.7, 21 were treated on average within 18.6 ± 16.0 hours from stroke onset while the other 34 patients were treated on average 3.4 ± 1.6 hours from stroke onset. Recanalization rates (82.8% vs. 85.7%; p = NS) and the rates of good neurological outcome (41.2% vs. 42.9%; p = NS) were similar. What was most encouraging was that the risk of symptomatic ICH was also comparable between the early and late treatment groups (8.8% vs. 9.5%; p = NS). The duration of ischemia was not a predictor of poor outcome or death. A larger multicenter, retrospective study confirmed these results in 237 anterior circulation stroke patients treated with IAT on average 15 ± 11.2 hours after stroke onset. In that study patients were also selected based on either CT- or MRI-based perfusion imaging. Ninety-day good clinical outcomes and mortality were 45% and 21.5%, respectively. Remarkably sICH occurred in 8.9%. Although both of these series were retrospective and need to be confirmed with prospective studies, these data suggest that patients with salvageable brain tissue and severe clinical deficits should be considered for EVT regardless of stroke duration.
Endovascular therapy has several potential advantages as compared to IV thrombolysis; chief among which are the longer time window and increased recanalization efficacy. Additionally EVT may be safer in situations where there is an increased risk of hemorrhage such as recent (<2 weeks) thoracic or abdominal surgery, extra-cerebral hemorrhage, arterial puncture in a noncompressible site, as well as in patients being treated with systemic anticoagulation ( Table 25-1 ). Mechanical embolectomy is the safest approach in such patients but even IAT may be safer due to the fact that lower doses of local thrombolytics may be given.
|
* Unruptured, incidental, nonthrombosed aneurysms are not a contraindication.
‡ Contraindications for thrombolysis between 3 hours to 4.5 hours from stroke onset.
Mechanical embolectomy with the MERCI clot retriever received FDA approval 10 years ago on the basis of the single arm MERCI study. That trial of 151 patients included patients treated within 3-8 hours of stroke onset and only evaluated the safety and feasibility of mechanical embolectomy. The clot burden in the MERCI study was on average greater than that in the PROACT II and MELT trials since all comers, including ICA occlusion, were enrolled; and although the IV tPA trials did not assess for arterial occlusion the mean NIHSS in MERCI was far greater consistent with more large vessel occlusions. Device-only recanalization (TIMI 2-3) was achieved in 46% but with adjunctive thrombolysis it increased to 60.3%. A good outcome (mRS ≤2) was achieved in 27.7% of patients and 43.5% were deceased by 90 days. Most informative was that the symptomatic ICH was only 7.8%, which was only slightly greater than the 6% seen with IV tPA. Therefore since the MERCI trial proved the safety of the device the FDA granted approval for “clot removal” not for stroke treatment.
The follow-up Multi MERCI trial was another multicenter single-arm trial of the first generation MERCI retrievers and the second-generation retriever with the added goal of exploring the technical efficacy and safety of embolectomy in patients with “failed” IV thrombolysis. Twenty-nine percent of patients had “failed” IV-tPA and 34.8% received intraprocedural IA thrombolytics. Device-only recanalization was noted in 55% but was 68% with adjunctive thrombolysis. The clinical results were similar to MERCI with a good outcome in 36% and 34% mortality. The rate of sICH was 9.8%. Much has been made of the relatively poor clinical outcomes in these trials compared with the outcomes in the randomized IV-tPA trials. Those are inappropriate comparisons, however, since the MERCI trials had patients with more severe strokes, larger thrombus burdens, and longer durations of ischemia. These trials were not designed or powered to show clinical efficacy; rather they were designed to show effectiveness in clot removal. Since they have been proven effective at clot removal and recanalization, which is the most effective treatment for stroke it has been assumed that they are effective at stroke treatment.
The MERCI Registry, the largest prospective registry of mechanical embolectomy, included 1000 “real-world” patients. It had no predefined exclusion criteria (Jovin T, Oral Presentation, ISC, February 2011, Los Angeles, California). The Registry patients were treated later than in the earlier studies, approximately 17% were treated beyond 8 hours from stroke onset. Recanalization was achieved in 80.1% of patients but only 31.6% had good outcomes and 33.4% died. Recanalization was the best predictor of good outcomes (mRS ≤2) but with an age disparity: compared with <60 year olds, patients >79 had an approximately half the probability of achieving a good outcome despite recanalization and 40% mortality. On the other hand there was no possibility (0%) of a good outcome if there was no recanalization. Mortality with successful recanalization in those <60 was 15%. With complete recanalization good neurological outcomes (70% vs. 10%) and mortality (<15% vs. 40-50%) were better in those with NIHSS <16 compared with those with NIHSS >25, respectively. The sICH rate was 7% overall but was lower with successful recanalization (5.4% with TICI flow grade of 3% compared to 9.2% if the final TICI grade was 0-1). Predictors of good outcome in a multivariate analysis included baseline NIHSS (OR 0.88; p <0.0001), age (OR 0.95; p <0.0001), and successful revascularization defined as TICI 2a-3 (OR 4.02; p <0.0001).
The second FDA approved device was the Penumbra (Penumbra Inc., Alameda, California) clot extraction device. Its clot extraction efficacy was validated in a 125-patient study with an 8-hour time window. Revascularization success (TIMI 2-3) and 90-day good outcomes were achieved in 81.6% and 25% of patients, respectively. Ninety-day mortality was 32.8% with 11.2% sICH. There was a trend for benefit with successful recanalization.
The poor clinical outcomes, and too often incomplete revascularization with these earlier devices, led to the development of a new class of devices designed to be safer and more effective. The so-called stent-trievers, or stent retrievers, combine the benefits of stenting (immediate flow restoration) with embolectomy devices (clot extraction) without leaving a stent permanently in the vessel ( Figure 25-2 ). Two randomized, noninferiority trials have been published comparing the recanalization and clinical efficacy of stent retrievers versus the MERCI retrievers in patients treated within 8 hours of stroke onset. The Solitaire With the Intention For Thrombectomy (SWIFT) trial tested the Solitaire device, and TREVO 2 tested the Trevo (Stryker Inc., Kalamazoo, Michigan) device. Enrollment in the SWIFT trial was halted after 113 patients were enrolled when a pre-planned interim analysis showed a major benefit of Solitaire over the MERCI device. The primary efficacy outcome of TIMI 2 or 3 flow was achieved more often with Solitaire (61% vs. 24%; OR 4.87; p <0.0001). In addition good neurological outcome (58% vs. 33%; OR 2.78; p = 0.0001) and 90-day mortality (17% vs. 38%; OR 0.34; p = 0.0001) were more favorable in the Solitaire group. The sICH rate was significantly lower with Solitaire (2% vs. 11%; OR 0.14; p = 0.057).
The TREVO 2 trial results were similar with 178 patients randomized. Recanalization (TICI 2 or greater) was higher with Trevo (86% vs. 60%; OR 4.22; p <0.0001) as was good clinical outcome (40% vs. 22%; OR 2.39; p = 0.013). There was no difference in sICH (7% vs. 9%; OR 0.75; p = 0.78) or 90-day mortality (33% vs. 24%; OR 1.61; p = 0.18). The trials differed in some endpoint definitions and there was a difference in sICH and good outcomes. At this time there are insufficient data to differentiate between stent retrievers, although their superiority over the older MERCI device seems to be real. Certainly the speed of recanalization with the stent retrievers in general is a major advantage (e.g., time to achievement of recanalization from guide catheter placement was 36 minutes with Solitaire vs. 52 minutes with MERCI; p = 0.038). Both the Solitaire and Trevo devices received FDA clearance for clot removal and are currently the defacto devices used by most centers performing EVT. As of this writing two randomized trials of mechanical embolectomy + medical therapy (including IV tPA if appropriate) vs. medical therapy alone have been halted due to “overwhelming efficacy.” Although the full trial results are not yet available the expectation is that mechanical embolectomy with stentrievers will become the standard of care treatment for large vessel occlusion within 12 hours of stroke onset.
Peri-procedural Management
The medical management of patients peri-procedurally has not been well studied during EVT. While basic measures such as supporting the airway and maintaining oxygenation are standard, other variables are not. The use of general anesthesia during EVT appears to be associated with worse neurological outcomes and increased mortality. However if patients are comatose, are unable to handle secretions, or maintain their airway the risk of not performing endotracheal intubation is likely far greater than the potential harm; otherwise all patients should be kept awake during EVT. Since the cerebral vessels are richly innervated they are quite sensitive to manipulation and the resultant headache may be an important sign of impending vessel injury.
An equally critical issue is blood pressure control. In the setting of ischemia cerebral autoregulation results in maximal arterial and arteriolar vasodilation distal to the site of occlusion, as a result cerebral blood flow (CBF) becomes directly proportional to mean arterial pressure. Consequently blood pressure elevation results in an increase in CBF and an increased risk of ICH, conversely lower pressures decrease CBF exacerbating the ischemia. It is therefore critical to keep blood pressure moderately elevated except in patients who have a very high risk for ICH or those with ongoing myocardial infarction for example. Although there are no validated guidelines the generally recommended range is 150-185 mm Hg. After a successful intervention blood pressure should be lowered into the normal range immediately to avoid the cerebral hyperperfusion syndrome.
Post-operatively patients should be transferred to a neurological intensive care until stable. Headache and a change in neurological status should warrant immediate neurological assessment and an emergent CT scan. The care of stroke patients in dedicated neurological units and by stroke specialists have been associated with decreased mortality and improved clinical outcomes.
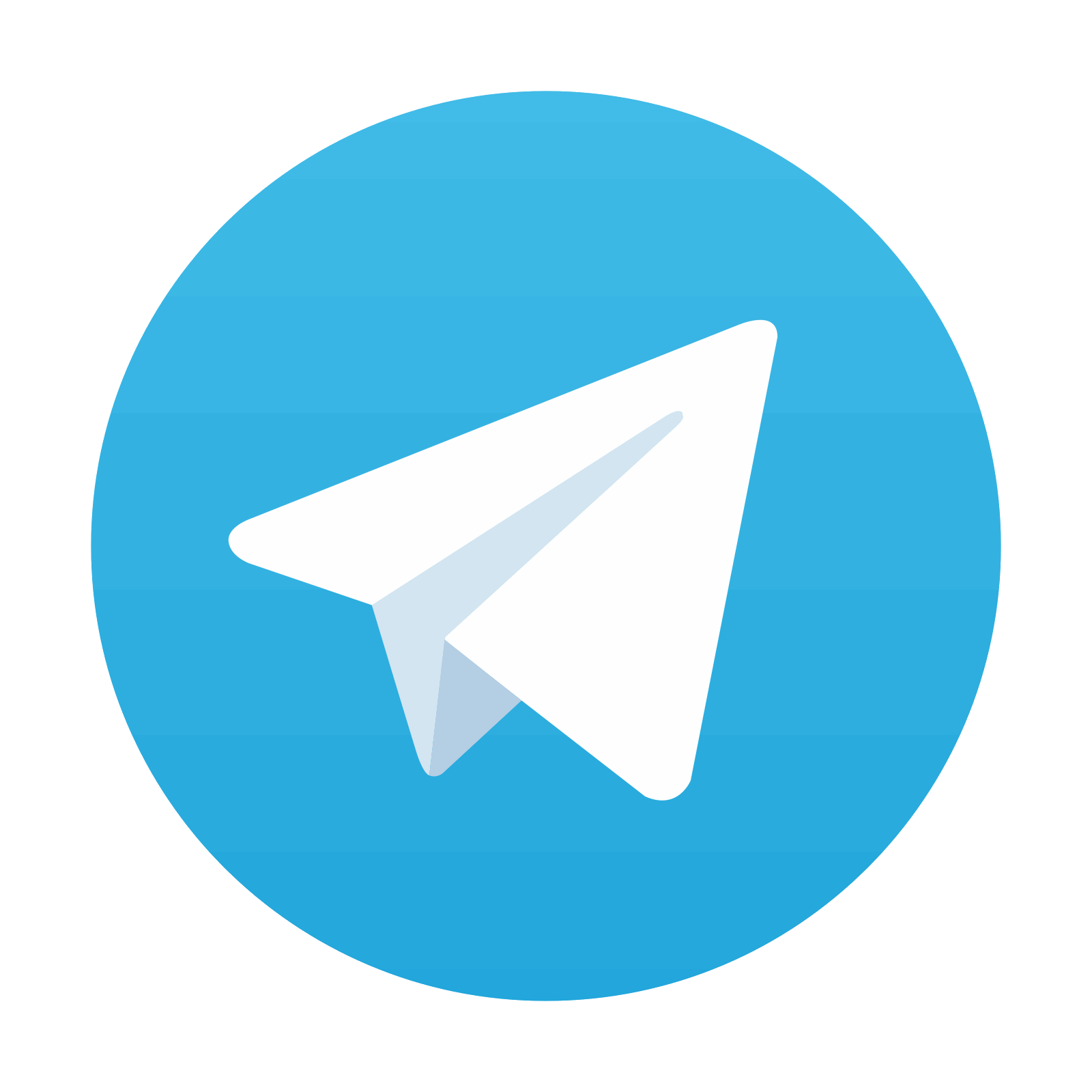
Stay updated, free articles. Join our Telegram channel

Full access? Get Clinical Tree
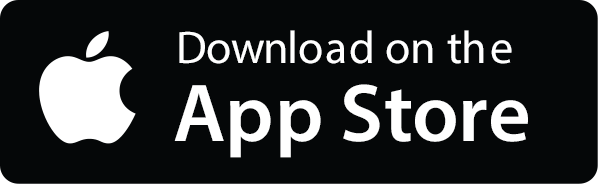
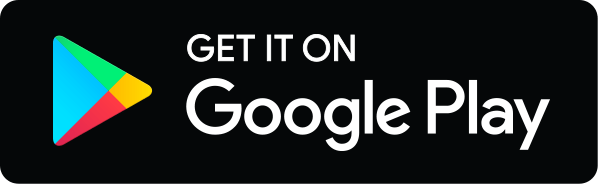