Intracerebral Arteries and Brain
6 Intracerebral Arteries and Brain
6.1 General Remarks
There are certain thin-walled areas in the skull where low-frequency ultrasound can penetrate the bone. Owing to the availability of these acoustic windows, the basal cerebral arteries can be interrogated with pulsed Doppler ultrasound, and the course of the arteries can be superimposed over the gray scale image of the brain parenchyma with color duplex sonography. The squamous portion of the temporal bone provides the most important acoustic window. Other windows are also available for transcranial imaging, such as the transorbital and transfrontal approaches. The foramen magnum provides a suboccipital window for transforaminal imaging of the vertebral and basilar arteries. These techniques are generally referred to as transcranial color-coded sonography (TCCS).
6.2 Transtemporal Approach
6.2.1 Examination Technique and Normal Findings
Transtemporal color duplex sonography is performed with low-frequency sector transducers. The transmission frequency is from 1 to 3 MHz, and the gray scale dynamic range should be at least 75 dB. These transducers are also used in echocardiography. The relatively low transmission frequency is a tradeoff between penetration of the cranial bone and spatial resolution. The thin bony layer of the temporal squama permits transtemporal imaging of the brain parenchyma, basal cerebral arteries, and large dural venous sinuses.
A good way to evaluate the acoustic conditions for transtemporal ultrasound is to start with an imaging depth of 16 cm and assess penetration based on visualization of the opposite calvarium. With a good acoustic window, this scan should also demonstrate the parenchymal brain structures with the basal cisterns and ventricular system. To evaluate the brain parenchyma, the contralateral hemisphere is imaged to ensure that the largest possible sound field is available for examination. Vascular structures are generally imaged ipsilateral to the selected acoustic window so that the relatively weak Doppler signals can be optimally detected.
Once orientation has been established from the parenchymal structures, velocity-based color Doppler imaging is switched on and, if necessary, the pulsed flow velocities of the basal cerebral arteries are measured.
The transtemporal approach also permits the evaluation of cerebral parenchymal perfusion. This requires the use of a low-frequency transducer (1–3 MHz) that can detect nonlinear echoes from ultrasound contrast agents. The physiology of the cerebral microcirculation, with a greatly reduced concentration of red blood cells compared with the larger basal cerebral arteries, plus the very slow flow velocity in the capillary bed (approximately 1 mm/s) pose insurmountable technical problems for conventional ultrasound. These problems can be overcome by utilizing the harmonic signature of ultrasound contrast agents in the microcirculation. The echo signal enhancer provides an optimum intravascular indicator of flowing blood in this setting.
Sonography of Brain Parenchyma
The examination begins with an axial B-mode image in the orbitomeatal plane. The scan plane lies on an imaginary line connecting the inferior orbital rim with the superior border of the external acoustic meatus. Frontal structures are displayed on the left side of the image, occipital areas on the right. Three standard planes have proven best for gray scale imaging of the brain parenchyma (Fig. 6.1).
Fig. 6.1 Mesencephalic plane (a), diencephalic plane (b), and cella media plane (c) demonstrated by magnetic resonance imaging (MRI) (left) and transcranial color-coded sonography (TCCS) (right). (a) Mesencephalon with the cerebral peduncles, substantia nigra (outlined in red), and raphe nuclei (arrow). Posterior to them is the aqueduct (magnified view in a2). (b) Hyperechoic pineal gland posterior to the third ventricle (yellow bar) with the hyperechoic ventricular walls (2) and hyperechoic choroid plexus of the contralateral occipital horn (3). (c) Hyperechoic choroid plexus of the ipsilateral occipital horn (4). Hypoechoic contralateral cella media with the echogenic ventricular walls (5) (diameter determined at the level of the yellow double arrow). Interhemispheric fissure (6) and frontal horn of the lateral ventricle (7).
The butterfly-shaped brainstem can be identified at the level of the mesencephalon. The dark brain parenchyma is rimmed by a light border representing the basal cisterns. Posterior to these structures is the cerebellum, where transverse gyri are occasionally depicted as hypoechoic features. Anterior to the brainstem and aligned with the transducer is a hyperechoic band, the lateral fissure, which transmits the M1 segment of the middle cerebral artery (MCA, Fig. 6.2).
Fig. 6.2 Axial mesencephalic planes for imaging the basal cerebral arteries: magnetic resonance angiography (MRA) and axial transcranial color-duplex sonography (TCCS). The different ultrasound planes are caused by different angulations (Fig. b to e angulation is more caudal). Insonated side: (a) MRA. (b) TCCS insonated from A1. Middle cerebral artery (MCA): M1 segment (1). Anterior cerebral artery (ACA): ipsilateral A1 (3) and A2 segments (4) and contralateral A1 segment (5). PCA: P1 (6), P2 (7), and contralateral P1 segment (9). (c) TCCS insonated from A1, same plane as b without color Doppler. Brain parenchyma: mesencephalon (13), lateral fissure (14), and interhemispheric fissure (15). (d) TCCS insonated from A2. MCA: division of the M1 segment into M2 branches (2). PCoA (10). ICA: C1 (11) and C3 segments (12). (e) TCCS insonated from A2, with the plane in d tilted basally. PCA: ipsilateral P1 (6), P2 (7), and P3 segments (8).
The mesencephalic plane is essential for parenchymal sonography in patients with movement disorders. The substantia nigra (SN) can be distinguished in the mesencephalon as a hyperechoic bean-shaped structure. It is particularly distinct when the mesencephalon is displayed with a magnification factor of 2 to 3 (Fig. 6.1, a2). Another important structure is the hyperechoic red nucleus, which appears farther medially. The mesencephalic brainstem is divided at its center by the aqueduct and raphe nuclei. When parenchymal sonography is done to investigate movement disorders, a relatively poor acoustic window with inadequate sound penetration may lead to misinterpretation due to an inability to detect increased SN echogenicity. At the same time, hyperechoic calcifications or fresh microhemorrhages should not be misinterpreted as pathognomonic signs of movement disorders.
Angling the transducer 10 degrees up will bring the plane of the thalamus into view (diencephalic plane). Normally the thalamus appears as a hypoechoic structure bordering on the third ventricle. Between both thalami a bright double outline that is parallel to the transducer face could be displayed. This is the third ventricle. This plane is useful for measuring the diameter of the third ventricle (Table 6.1). Posterior to these structures is the pineal gland, which often appears hyperechoic due to the presence of calcifications. Just posterior to the pineal gland and farther from the transducer is another hyperechoic structure, the choroid plexus of the occipital horn of the contralateral lateral ventricle.
The transducer is angled another 20 degrees up to display the plane of the cella media. The posterior, hyperechoic structure located in the brain parenchyma of the ipsilateral hemisphere is the choroid plexus of the ipsilateral lateral ventricle. The interhemispheric fissure is seen running parallel to the transducer face, passing through the whole plane of section. The frontal horn of the ipsilateral lateral ventricle is visible anteriorly, and the lateral border of the contralateral lateral ventricle can be seen at the center of the scan plane, at the level of the cella media, running parallel to the interhemispheric structures.
These three scan planes serve to establish orientation in handheld transtemporal axial parenchymal sonography and are initially confusing for the computed tomography (CT)-accustomed eye because they are oblique. With some initial practice in normal subjects, however, they can easily be located, recognized, and reproduced. In the imaging of cerebral hemorrhage or tumors, a coronal view of the pathology should be obtained to allow for volume determination.
Color Duplex Sonography
After the imaging depth has been shortened to 10 cm, velocity-based color Doppler is switched on in the axial plane of the mesencephalon (Fig. 6.2, Fig. 6.3). This will demonstrate the M1 segment of the MCA in the lateral fissure, with flow directed toward the transducer (coded in red by convention). The anterior cerebral artery (ACA, A1 segment) runs toward the midline in continuity with the MCA and is coded in blue, indicating flow away from the transducer.
Fig. 6.3 Axial and coronal transcranial color-coded sonography (TCCS) planes for imaging the basal cerebral arteries: basilar artery (BA), anterior cerebral artery (ACA), internal carotid artery (ICA), middle cerebral artery (MCA), and posterior cerebral artery (PCA). (a) Magnetic resonance angiography (MRA). Left: MCA, ACA, and ICA. Right: PCA and BA. (b) Axial TCCS. MCA: division of the M1 segment into M2 branches (1) and M1 segment (2). ACA: ipsilateral A1 segment (3) and contralateral A1 segment (5). (c) Coronal TCCS. MCA: M1 segment (2). ACA: ipsilateral A1 (3) and A2 segments (4) and contralateral A1 segment (5). ICA: C1 segment (11). (d) Axial TCCS. PCA: ipsilateral P1 (6), P2 (7), and P3 segments (8). (e) Coronal TCCS. PCA: ipsilateral P1 segment (6) and contralateral P1 segment (9). BA: tip of the BA (10).
The head of the basilar artery (BA) can be demonstrated in the coronal plane, which is oriented perpendicular to the axial plane.
The basal vein of Rosenthal is commonly found at the level of the P3 segment of the PCA. Like the P3 segment of the PCA, it is coded blue in the color Doppler image. As the vein runs occipitally, it drains first into the vein of Galen and then into the straight sinus. The tip of the transducer can be raised at this point to demonstrate the transverse sinus and the proximal portion of the superior sagittal sinus.
Pulsed Doppler can be activated to selectively measure flow velocity in the basal cerebral arteries, where marked differences can be found in different vessels (Table 6.2). Angle correction should always be used for accuracy if a sufficiently long vascular segment could be imaged. In practical terms, the segment interrogated by color duplex sonography should be approximately 2 cm long and angle correction should not exceed 30 degrees so that the measurement error will be small. The flow velocities in the cerebral veins and sinuses can vary substantially in different patients due to the high variability of individual venous anatomy.
Table 6.2 Normal values for mean angle-corrected flow velocities in various segments of the basal cerebral arteries without the use of ultrasound contrast agents.4 , 46 , 60 , 61 On average, measured velocities are 24% higher with contrast agents than without contrast agents.
Vessel | Flow velocities (in cm/s, followed by range of mean values) | |
Systolic | End-diastolic | |
Middle cerebral artery | 110 (100–119) | 50 (40–55) |
Anterior cerebral artery | 95 (80–105) | 40 (30–50) |
Posterior cerebral artery | 70 (55–75) | 35 (30–35) |
Vertebral artery | 55 (40–60) | 25 (20–30) |
Basilar artery, proximal | 60 (50–70) | 35 (30–35) |
The ability to demonstrate the basal cerebral arteries and cerebral veins depends greatly on the quality of the acoustic window. The acoustic window is inadequate in 14% to 20% of the elderly Caucasian population. By using an ultrasound contrast agent, however, the arteries can be evaluated in 76% to 84% of patients with a poor acoustic window. In this way the basal cerebral arteries can be successfully imaged through a temporal window in 95% to 98% of all cerebrovascular patients.71 The contrast agent does affect blood velocity measurements, with the result that measured flow velocities are approximately 24% higher (±7.4%) on average than when a contrast agent is not used.34 , 67 This occurs because the agent increases the number of fast-flowing scatterers that are not detected in unenhanced scans because their signals are too weak.
Sonography of Parenchymal Perfusion
The transtemporal acoustic window can also be used to evaluate cerebral parenchymal perfusion. This requires the use of a low-frequency transducer that can detect nonlinear echoes from ultrasound contrast agents. Various kinetic analyses can be performed. The analysis of bolus kinetics is analogous to the method used in perfusion CT and magnetic resonance imaging (MRI). It involves imaging an ultrasound contrast bolus in a gated mode as it passes through the microcirculation.52 , 66
A newer alternative technique is flash replenishment imaging in which a contrast agent administered by infusion72 or bolus injection is destroyed in the microcirculation by a high-energy ultrasound burst, whereupon the replenishment of the contrast-depleted tissue volume is displayed in real time (“flash replenishment kinetics”).33 This process takes less than 10 s per imaging plane, and therefore motion artifacts are significantly reduced.
Analysis of the displayed kinetics relies on mathematical models, and modern ultrasound systems have software tools available for this purpose. These tools can also generate a parametric display of individual parameters in the kinetic models, which greatly simplifies display and documentation.
The imaging planes for perfusion scans are the standard gray scale imaging planes described above. The thalamic plane affords the best view. Approximately 80% of cerebrovascular patients have an acoustic window that is adequate for successful transcranial color duplex sonography without contrast agents. Accordingly, the method currently works in only about 60% of cerebrovascular patients and is still experimental in nature.
6.2.2 Vascular Pathology
Stenosis
An occlusion or stenosis of intracranial large arteries can be detected in the acute phase of ischaemic stroke in about 42% of patients. The diagnostic accuracy of TCD and TCCD for detecting stenosis or occlusion of intracranial large arteries in people with acute ischaemic stroke was evaluated in a recent Cochrane review.44
Sensitivity and specificity estimates for TCD and TCCD were high (95% (95% CI = 0.83 to 0.99) and 95% (95% CI = 0.90 to 0.98), respectively).
Intracranial stenoses of the basal cerebral arteries most commonly result from atherosclerosis and are identified as the cause of ischemia in 5% to 20% depending on age of stroke30 patients in the chronic phase.59 , 84 During the acute phase of cerebral infarction, moreover, transient stenoses are found in association with recanalizing embolic occlusions. In rare cases, cerebral vasculitis49 , 54 or vasospasms related to various underlying diseases are found to be causative of stenosis.
General Doppler and color duplex criteria for intracranial stenosis and occlusion are reviewed in Table 6.3 and Table 6.6. The most important general Doppler criterion for stenosis is the presence of circumscribed flow acceleration with an increase in blood flow velocity (BFV) by at least 20%, or preferably 50%,56 combined with an intra- and poststenotic flow disturbance.
Hyperperfusion in the MCA can be excluded by the MCA/ICA index (ratio of peak systolic angle-corrected flow velocities in the MCA and extracranial ICA). Values greater than 2 are borderline, and values greater than 3 are typical of stenosis or vasospasm. This is indicated in color duplex by circumscribed aliasing when the pulse repetition frequency (PRF) of the system has been optimized for the prestenotic vascular segment. In this case the abnormal color signals serve as indicators of stenosis.
Several other Doppler criteria of stenosis should be applied even when a color duplex system is used: the absolute value of flow velocity, its comparison with the corresponding contralateral segment, spectral abnormalities such as low-frequency symmetrical frequency components indicating vessel wall vibrations due to a high-grade stenosis, and low velocities in vascular segments distal to the stenosis (Table 6.3).
Transcranial color duplex sonography, unlike conventional transcranial Doppler (TCD), can also provide etiologic clues to the cause of an intracranial stenosis. Initial studies suggest that stenoses with a high echo return are due to atherosclerosis,11 as distinguished from a brain embolism undergoing recanalization (Fig. 6.4, Fig. 6.5). It should be added, however, that most atherosclerotic stenoses are not echogenic.
Fig. 6.4 Bilateral stenoses of the middle cerebral artery (MCA) (right ≥ 50% and left < 50% based on Baumgartner criteria22) in a patient with primary cerebral vasculitis.
Fig. 6.5 Stenoses in the M1 segment of the middle cerebral artery (MCA). (a) Small hyperechoic stenosis. (b) Hyperechoic stenosis (arrow) consistent with excentric calcified plaque at the stenosis site. (c) Hyperechoic stenosis (arrow) consistent with concentric calcified plaque at the stenosis site.
To date there has been no accurate and angiographically validated system for grading stenosis based on Doppler ultrasound parameters comparable to the grading system used for proximal internal carotid stenosis. This is mainly due to a lack of precision in the angiographic measurement of intracranial stenosis. Caliber estimates based on transcranial color duplex images are inherently imprecise due to inadequate spatial resolution and the dependence of the color signal on system parameters. The key advantage of TCCS over TCD lies in its more accurate localization of Doppler signal abnormalities given the very close proximity of basal cerebral vascular segments to one another. This has raised problems in examinations with conventional transcranial Doppler ultrasound, making it difficult, for example, to distinguish between proximal MCA and distal ICA stenosis or determine the identity of a collateral vessel.
Segment-specific stenosis criteria that have been described in the literature are summarized in Table 6.4, where the most commonly used criteria are shown in boldface print. Many laboratories use the Baumgartner criteria 6 , 14 for angle-corrected transcranial color duplex sonography. If angle correction cannot be performed, the TCD criteria can be used; again, every laboratory should determine which criteria to use. The SONIA criteria 87 (Stroke Outcomes and Neuroimaging of Intracranial Atherosclerosis criteria) differentiate ≥ 50% stenosis and ≥ 70% stenosis, a system that is particularly useful in detecting the progression of stenosis.
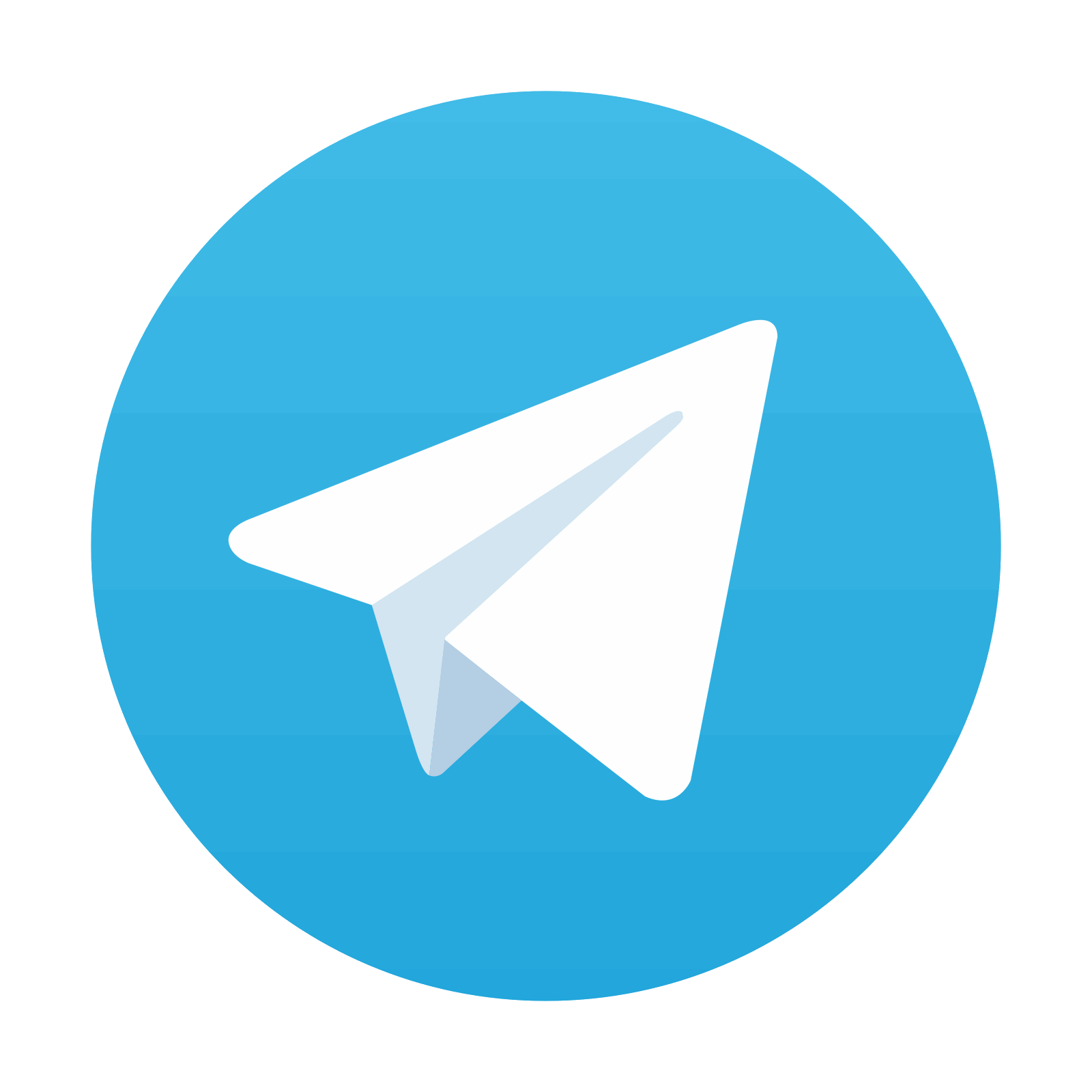
Stay updated, free articles. Join our Telegram channel

Full access? Get Clinical Tree
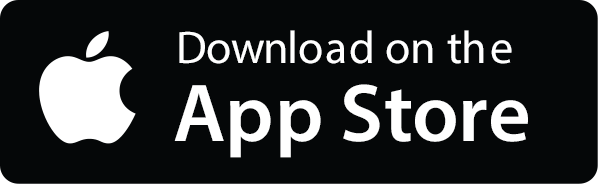
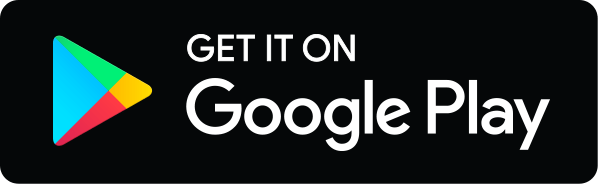