18FDG | 18F-fluorodeoxyglucose |
CAD | coronary artery disease |
EF | ejection fraction |
LAD | left anterior descending |
LCX | left circumflex |
LV | left ventricular |
PET | positron emission tomography |
RCA | right coronary artery |
Heart failure is a common cardiac diagnosis and the prevalence is increasing, with a prediction of more than 8 million affected adults in the United States by 2030.1 Despite recent improvement in survival, prognosis remains poor with a 5-year mortality of 50% following diagnosis.2 The most frequent etiology continues to be coronary artery disease (CAD) in the developed countries.3 Evidence supports the idea that viability imaging to guide revascularization therapy may be the best strategy for patients with multiple comorbidities and severe left ventricular (LV) dysfunction.4–12 Several studies have demonstrated that revascularization in appropriate patients with CAD improves outcomes in these high-risk patients.6 Viable but dysfunctional myocardium caused by abnormal coronary flow may restore its function with normalization of coronary blood flow. In this chapter, we review the approach to the interpretation of viability scans acquired with 18F-fluorodeoxyglucose (18FDG) and positron emission tomography (PET) and reporting with recommendations for clinical management. This topic has been reviewed in the recent PET imaging guidelines cosponsored by the American Society of Nuclear Cardiology and the Society of Nuclear Medicine and Molecular Imaging.13
Viable, stunned, and hibernating myocardium are important concepts that need to be understood for appropriate interpretation of PET viability scans. Viable versus nonviable dysfunctional myocardium is a simple binary classification that refers to dysfunctional myocardium that may recover (viable or stunned/hibernating) or not (nonviable or scar) with revascularization.
Dysfunctional viable myocardium may have a spectrum of different levels of metabolism and flow caused by repeated transient or persistent ischemia.14 Stunned myocardium may occur after ischemia and is characterized by normal rest flow and transiently impaired contractility caused by previous ischemia.15–17 Microscopically, stunned myocardium has minimal structural injury but complex metabolic changes.18–20 The severity of impaired metabolism will depend on the number, extent, and duration of the ischemic episodes.21 Stunned myocardium may completely recover normal function or progress to a hibernation (still viable) or irreversible fibrosis (nonviable). Hibernating myocardium is dysfunctional myocardium with reduced rest flow and function that occurs after chronic repeated episodes of reduced blood supply.21 By definition, hibernating myocardium is considered viable and has the capacity to recover function if adequate revascularization is performed in a timely fashion.22,23 Glucose metabolism is preserved or even enhanced in hibernating myocardium because of preferential use of glucose by ischemic myocardium.24–31 18FDG is a glucose analogue taken up by the myocyte-like glucose using sarcolemmal transporters and phosphorylated by hexokinase to 18FDG-phosphate.32 18FDG-phosphate is “trapped” in the myocyte because it cannot be further metabolized and is used as a marker of glucose transport by viable ischemic (hibernating) or nonischemic myocardium with viability imaging. Thus, preservation of 18FDG uptake in areas of reduced perfusion is consistent with viability (perfusion-metabolism mismatch) and indicates hibernation. Conversely, reduced 18FDG uptake in a region of reduced perfusion and of similar or greater degree indicates nonviability (perfusion-metabolism match) and indicates scar. Of note, regions with normal perfusion are viable, even with reduced 18FDG, and may represent stunned myocardium.
The standard interpretation of metabolic FDG studies is in comparison to rest myocardial perfusion. It is virtually impossible to interpret a metabolic FDG study without such comparison. 18FDG PET images are best interpreted with rest PET perfusion images. Rest PET perfusion images are typically acquired with either 13N-ammonia or 82Rb. Although SPECT perfusion images can be used for comparison, interpretation can be difficult because attenuation artifacts in nonattenuation-corrected SPECT images or possible suboptimal correction when attenuation-correction is available.13
Image interpretation begins with examination of the coregistration of the fused emission and transmission images using the transaxial, coronal, and sagittal slices.13 Misalignment may result in attenuation correction artifacts with undercorrection possibly resulting in anterior or anterolateral perfusion defects or overcorrection resulting in inferior wall “hot spots” on 18FDG imaging and errors in interpretation if not recognized.33 18FDG and perfusion images are reoriented and displayed as short-axis view (SAO), horizontal long axis (HLA), and vertical long axis (VLA) slices.13 All slices need to be aligned and displayed adjacent to each other and interpreted according to the ASNC 17-segment model.13
Generally, the format is that the rest perfusion study (the same position as a “stress/rest” study) be above the 18FDG study. The 18FDG and perfusion images need to be normalized to the same normal myocardial region, which is usually the region on the perfusion image with the highest counts.13 This normal region could also be identified or confirmed as normal from stress perfusion images or angiographic data if available. The electrocardiogram should also be reviewed at this time, because the left bundle branch block may lead to reduced 18FDG uptake in the septum.34 The septal region should not be used for normalization in patients with left bundle branch block (LBBB).13 Persistent blood pool activity may alter myocardial activity and decrease defect severity on perfusion imaging or increase apparent 18FDG uptake on metabolic imaging. Impaired LV systolic function may result in slower blood pool clearance. Blood pool activity should be minimized by optimal timing of the image acquisition or frame selection for the static images using later frames. Alternatively, the display settings for the lower limits of the color or grayscale can be adjusted to correct for blood pool differences.
Regional perfusion and 18FDG metabolic images are evaluated together. Dysfunctional regions can be identified from gated perfusion images or from other clinical data and are the focus of attention. In a given region, perfusion is assessed as reduced or preserved. Four patterns of perfusion-metabolism can be seen (Figures 11-1, 11-2, 11-3, 11-4) and are useful to separate viable from nonviable myocardium13: (1) reduced perfusion and preserved 18FDG (perfusion-metabolism mismatch) identifying hibernating myocardium (viable). (2) Reduced perfusion and 18FDG (perfusion-metabolism match) indicating scar (nonviable). (3) Preserved perfusion and 18FDG showing normal myocardium (viable). (4) Preserved perfusion and reduced 18FDG (reversed perfusion-metabolism mismatch) can be seen in patients with repetitive stunning, in the septum with LBBB,35 with acute coronary syndrome and early following revascularization20 and with diabetes35 (viable). These patterns may coexist in a region or coronary territory. The severity of perfusion reduction can be used to define scar as nontransmural (<50%) or transmural (>50%). The extent of hibernating myocardium or scar (% of LV) can be described using qualitative or semiquantitative approaches as small (<10%), moderate (10% to 20%) or large (>20%). In patients with severe LV dysfunction caused by CAD, moderate or larger extents of hibernating myocardium are associated with improved outcomes with revascularization9 and large amounts of scar predict less recovery of LV function.7 Management recommendations can be suggested on the basis of the extent of hibernating myocardium and scar.
Figure 11-1
Hibernating Myocardium and Scar.The PET 13NH3 perfusion images show reduced perfusion in the septal, mid- and apical anterior and mid- and apical anterolateral regions and apex (A). The 18FDG images show preserved uptake in the mid- and apical anterior and mid- and apical anterolateral regions consistent with hibernating myocardium and reduced uptake in the septum and apex indicating scar (A). Quantitative analysis (B) determined scar (match defect) as 20% LV and hibernating myocardium (mismatch) as 15% LV. The University of Ottawa Heart Institute decision-guiding flow map (C) is used to guide recommendations for management based on the extents of scar and hibernating myocardium and assuming decreased left ventricular ejection fraction (LVEF) is caused by extensive ischemic heart disease (IHD).



Figure 11-2
ScarPET 13NH3 perfusion images show severely reduced perfusion in the inferior regions (A). The 18FDG images show severely reduced uptake in inferior regions indicating scar (A). Quantitative analysis (B) determined scar (match defect) as 27% LV and hibernating myocardium (mismatch) as 2.8% LV.
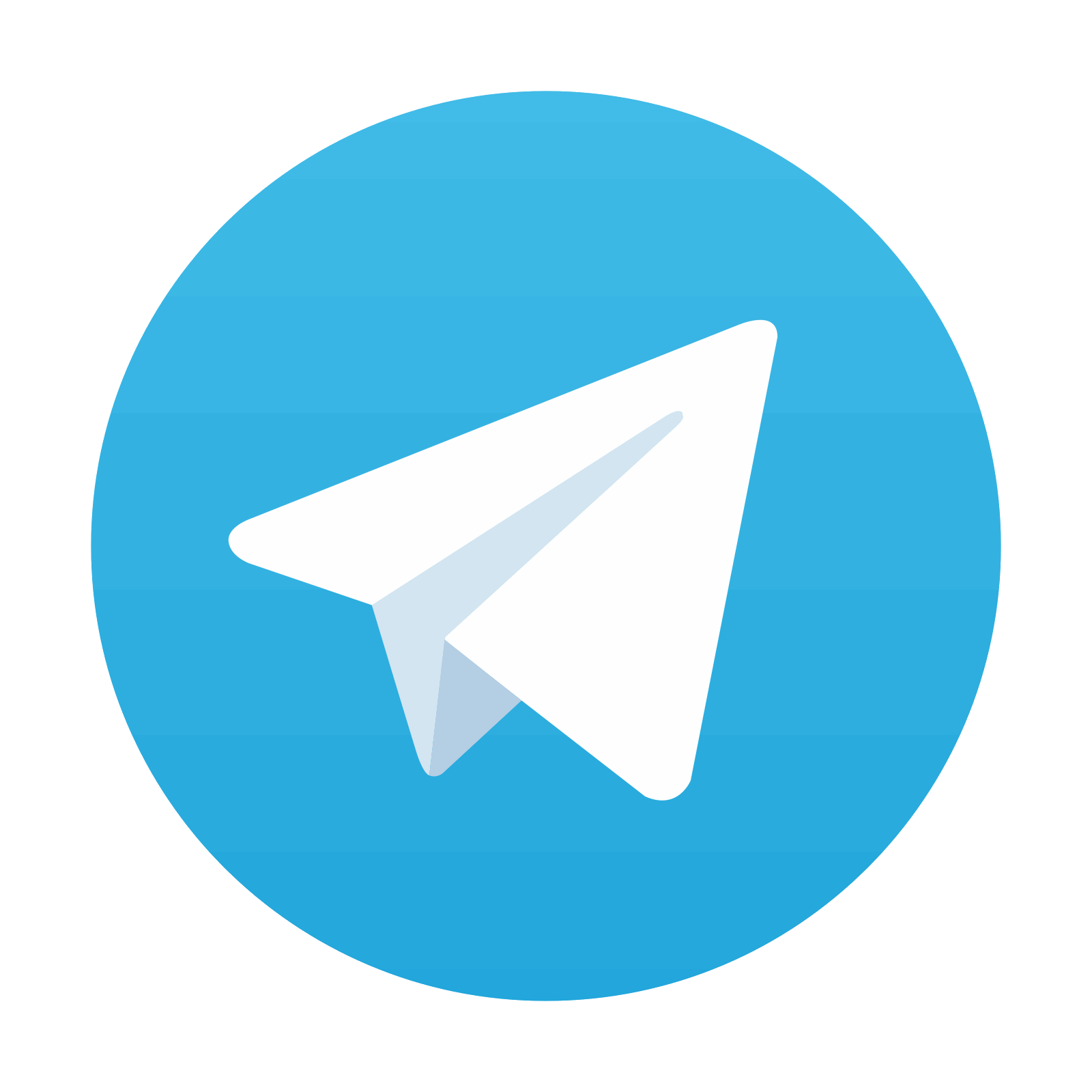
Stay updated, free articles. Join our Telegram channel

Full access? Get Clinical Tree
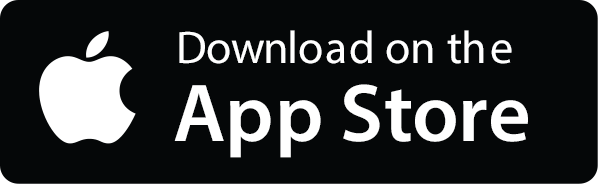
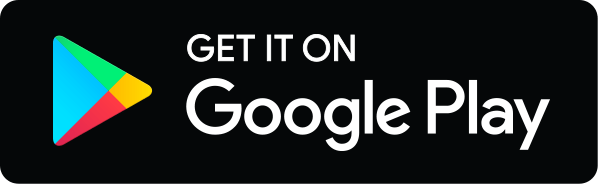
