Cardiac positron emission tomographic (PET) myocardial perfusion imaging offers patients and referring health-care providers a superior product with high diagnostic accuracy and risk stratification. As with SPECT, the interpretation of the images is extremely important to the resulting product. Physicians with experience in interpreting single-photon emission computed tomography myocardial perfusion imaging (SPECT MPI) are familiar with the imaging artifacts that reduce confidence in the interpretation of SPECT MPI studies (eg, soft tissue attenuation, gastrointestinal (GI) tracer activity in close proximity to the heart, and patient motion). Interpretation of PET MPI studies requires an understanding of the fundamental differences between PET and SPECT (Table 3-1) and the imaging artifacts unique to PET MPI. As rubidium-82 (82Rb) is the most commonly used radionuclide tracer for PET MPI, the discussion pertains primarily to interpretation of 82Rb PET MPI studies. This chapter examines key aspects to successful interpretation, including differences in interpretation between SPECT and PET, artifacts unique to PET, approaches to interpretation, and, finally, reporting.
Traditional SPECT | 82Rb PET | |
Spatial resolution (mm) | 12-15 | 4-6 |
Photon energy (keV) | 68-167 | 511 |
Attenuation correction | Rarely used | Routine and robust |
Scatter correction | Rarely used | Routine and robust |
GI tracer interference | Common | Minimal |
Timing of stress imaging | post-stress | Peak-stress |
Acquisition of angular projections | Sequential | Simultaneous |
As a result of the robust methods for correction for soft tissue attenuation and photon scatter, a normal PET MPI study will generally appear “more normal” than a normal SPECT MPI study. That is, in normal subjects, image quality will typically be good to excellent1 and myocardial tracer activity will be more homogeneous with PET MPI than SPECT MPI. Unlike SPECT MPI (in which breast attenuation results in variability in normal perfusion patterns for women and men), normal PET MPI perfusion patterns for women and men are similar to one another. Owing to the superior myocardial uptake kinetics of PET MPI tracers and the ability to measure left ventricular systolic function at peak stress, an abnormal PET MPI study will appear “more abnormal” than an abnormal SPECT MPI study. Therefore, interpretative confidence is higher for PET MPI studies than for SPECT MPI studies.1 However, confident interpretation of PET MPI studies requires that the PET MPI study is performed properly and that study quality is confirmed with automated quality control software. The use of quality control software is mandatory for PET MPI, because it is impossible to exclude artifacts related to patient motion or incorrect attenuation correction (misregistration of transmission and emission datasets) solely by reviewing the reconstructed tomographic slices. Unlike traditional SPECT MPI in which the angular projections are acquired sequentially and the rotating raw planar images are available for review, PET MPI images are acquired simultaneously by circular arrays of detectors. As a result, there are no rotating raw images for visual assessment of study quality with PET MPI.
There are common normal variants that need to be recognized when interpreting PET MPI studies. Owing to the partial volume effect and the superior spatial resolution of PET, the apical region will frequently demonstrate a mild reduction in tracer activity on both resting and peak-stress images in normal subjects (Figure 3-1A). The apical defect is a result of the normal anatomic thinning of the apical myocardium relative to the mid- and basilar myocardial regions. This is illustrated in Figure 3-1B. The peak-stress images are displayed directly above the corresponding resting images. There is mildly reduced 82Rb activity in the apical region on both the rest and peak-stress images. There is GI uptake of 82Rb evident on the resting images, but the GI tracer activity does not interfere with image interpretation.
Although there are commercially available quantitative PET MPI software programs that include gender-specific normal databases for assessment of regional 82Rb distribution, the software programs can fail to recognize the normal “apical thinning variant” (Figure 3-1C). The mild reduction in apical tracer activity is incorrectly designated as a true perfusion defect by the quantitative perfusion software program. Apical thinning is associated with normal wall motion on peak-stress and resting images. Regional wall thickening is normal in the apical region on both resting and peak-stress confirming the normal apical thinning variant. In this case, the left ventricular ejection fraction (LVEF) increased 5% during stress acquisition, compared to rest. A normal “LVEF reserve” (peak-stress LVEF minus resting LVEF) of 5% is a typical finding in normal subjects.
The apical thinning variant is even more commonly observed during 13N-ammonia imaging because of the higher spatial resolution of 13N-ammonia compared to 82Rb, and fluorine-18 (18F) labeled perfusion tracers (in clinical development) would be expected to demonstrate apical thinning because of the very high spatial resolution of 18F. Another normal variant to recognize is the occasional reduction in lateral wall tracer activity on 13N-ammonia PET images.2 To date, this defect is unexplained, but may be incorrectly interpreted as being positive for ischemia.
The spatial resolution for PET perfusion tracers varies because of differences in the energy of the positrons emitted from the nucleus during radioactive decay. Positrons with higher energy travel longer average distances before interacting with an electron, resulting in mutual particle annihilation and generation of two 511 keV photons. This distance traveled by the positron from the nucleus to the site of interaction with an electron is referred to as the positron range of the PET radionuclide. Table 3-2 lists the half-lives and positron ranges of PET radionuclides. Perfusion images acquired with radionuclides with a long positron range (eg, 82Rb) demonstrate lower spatial resolution and mild image blurring. Images acquired with radionuclides with a short positron range (eg, 18F) demonstrate higher spatial resolution and sharper delineation of the left ventricular cavity and endocardial surface. Despite these apparent limitations, it is clear that the overall image quality of any PET tracer is superior to the lower-energy technetium (SPECT) tracers.
All PET MPI studies are routinely processed using robust attenuation correction, either with a radionuclide line source or CT. Thus, the routine artifacts of soft tissue attenuation from body habitus are no longer present in the vast majority of patients. The three primary causes of artifact with PET are discussed in the next sections.
Similar to SPECT MPI, patient motion during image acquisition produces motion artifacts on PET MPI studies (Figures 3-2, 3-3, and 3-4). Patient motion is a more significant problem for PET MPI than for SPECT MPI, primarily because PET motion cannot be “corrected”3 owing to the continuous acquisition of the PET data, which means that one cannot isolate where the motion occurred. Thus, recognition of this artifact with PET, particularly if it affects the stress study alone, is important in reducing false-positive interpretations.
Table 3-3 outlines the factors influencing the impact of patient motion on PET and SPECT MPI. As mentioned previously, PET MPI images are acquired simultaneously by circular arrays of detectors, and, because of the continuous acquisition of data on each detector, visual identification of patient motion during image acquisition cannot be identified by reviewing rotating images. Therefore, patient motion is harder to detect on PET MPI studies, although some software programs can alert the reader to its presence. Positive identification of patient motion is left to evaluation of the processed images. Thus, being able to recognize the presence of motion on PET images is very important. Visually, the image appears distorted and blurred, similar to an extreme example of motion artifact during SPECT. Two examples of patient motion during cardiac PET MPI with rubidium-82 are shown in Figures 3-2, 3-3, and 3-4.
Traditional SPECT | Rubidium-82 PET | |
Acquisition of angular projections | Sequential | Simultaneous |
Review of cine images for motion | Yes | No |
Time per image acquisition (min) | 12-15 | 5-6 |
Continuous time under camera (min) | 12-15 | 30-40 |
Motion detection software | Yes | Yes |
Motion correction software | Yes | No |
Able to repeat image for motion | Yes | No |
Figure 3-2 is an example of substantial motion artifact on the peak-stress data. This is characterized by a distortion of the entire image, with elongation of the ventricle on the short axis images. There are both anterior and inferior abnormalities, which cannot be mistaken for CAD but are consistent with motion artifact. This defect is so severe that the study should be repeated, rather than assuming that the study is normal (with motion artifact) or abnormal. A second example of less severe patient motion artifact is shown in Figure 3-3. This, too, affects only the peak-stress data. There are “contralateral” defects in the anterior and inferior regions, with distortion of the entire image. The patient motion produces a “slit-like” appearance, which does not appear typical for CAD. This can be interpreted as “probably normal” if the reporting physician were comfortable with this. Also note that the TID ratio may be spuriously abnormal (TID >1.13) because of the patient motion affecting the measured cavity volumes. A third example of motion is shown in Figure 3-4. In this case, patient motion is present on both peak-stress and resting images. In both “cool” and gray-scale, the left ventricle appears square rather than round on short axis projections. There are slit-like deformities in the septal, lateral, and inferior segments that do not have the typical appearance of CAD, with similar characteristics on peak-stress and resting images. This study can be considered “probably normal” or the study can be repeated.
Quality-control software is capable of detecting and quantifying patient motion, but robust methods for motion correction are not yet available for PET MPI and are difficult because of the continuous acquisition of data, such that individual datasets cannot be corrected. On account of the short 75-second half-life of 82Rb, the presence of severe patient motion requires repeat image acquisition, which can be accomplished easily on the rest image, but more difficult on stress image due to the short-lived hyperemic phase. Therefore, prevention of patient motion is much more important for PET MPI than for SPECT MPI. The shorter scan times for emission image acquisition for PET (5–7 minutes) compared to traditional SPECT (12–14 minutes) reduces the likelihood of patient motion during imaging. However, unlike traditional SPECT in which the patient leaves the imaging table between rest and poststress image acquisitions, the patient remains continuously in the PET scanner for the full 30–40 minute duration of a standard rest-stress 82Rb PET MPI protocol. Therefore, patient comfort and patient cooperation are critically important for PET MPI. It is particularly important for the technologists to coach the patient to not move specifically during the short acquisition phases of rest and stress PET. One approach to addressing the problem of patient motion is to acquire dynamic sequential images rather than a single static image. In this way, the frames degraded by patient motion can be rejected and the remaining frames that are free of patient motion can be used for image reconstruction.4
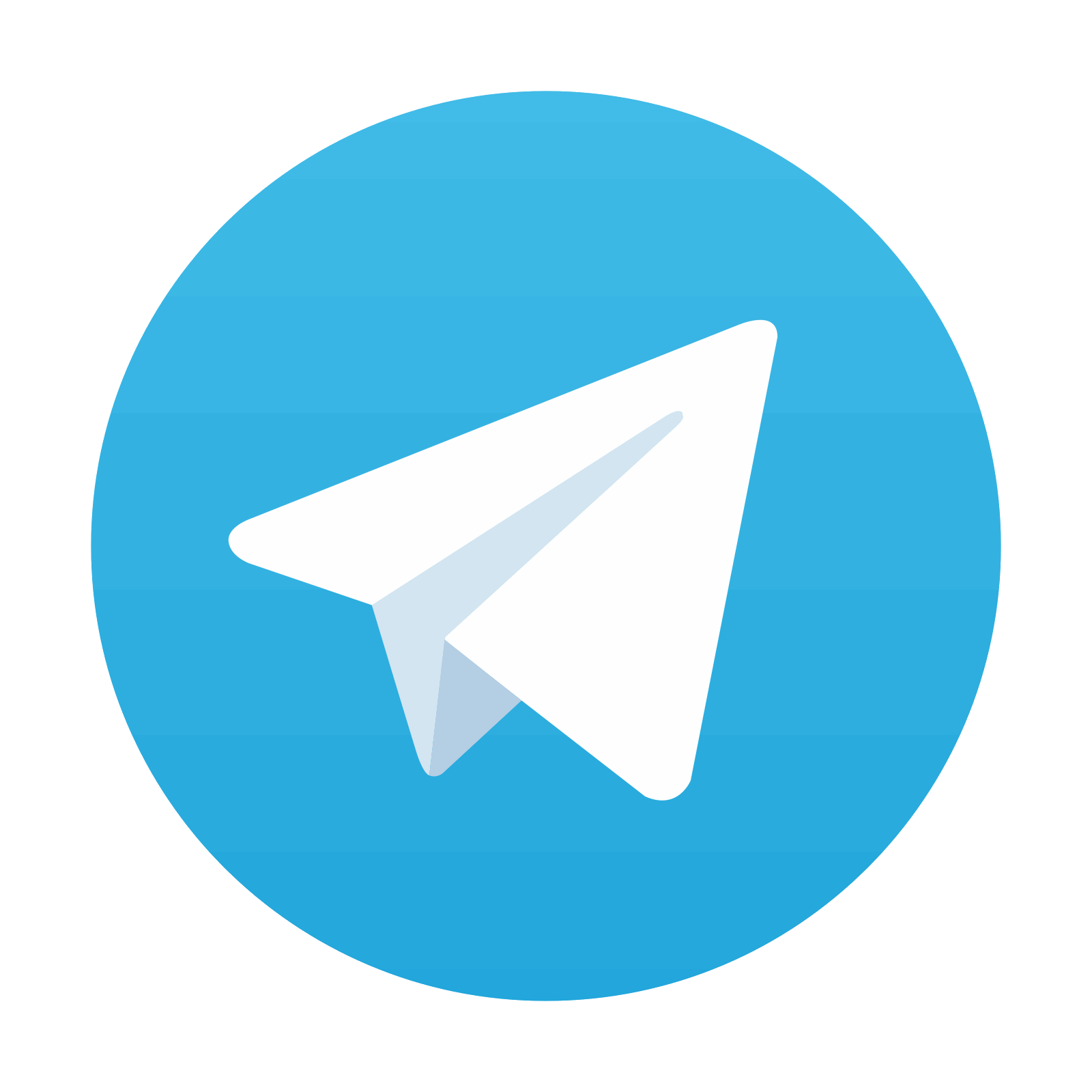
Stay updated, free articles. Join our Telegram channel

Full access? Get Clinical Tree
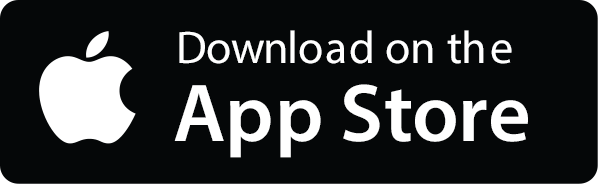
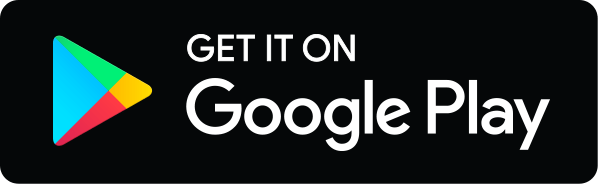