Injury to the Brain
INTRODUCTION
Traumatic brain injury (TBI) is a disruption or alteration of brain function due to external forces. The disruption of function may be transient or long lasting and may vary in severity. The external forces creating the injury may be the result of a variety of insults including acceleration or deceleration, direct compression, penetrating objects, combined effects, and complex mechanisms such as in blast. It may produce subtle effects not discernible on radiological imaging, focal injuries such as fractures, contusion, subarachnoid hemorrhage (SAH), subdural hemorrhage (SDH), epidural hemorrhage (EDH), or intraparenchymal hemorrhage (IPH), or more widespread damage such as diffuse axonal injury (DAI). All injuries and symptoms, even if apparently minor on initial presentation, should be taken seriously since injuries may rapidly progress and become life-threatening.
EPIDEMIOLOGY
The exact number of people suffering TBI is unknown since many individuals suffering mild or moderate TBI do not seek medical attention, and some who suffer severe traumatic injuries do not survive to receive medical attention.
Of those who do receive medical attention in an emergency department, approximately 1.4 million people per year suffer TBI. Of these patients, approximately 1.1 million are treated and released, 240,000 are hospitalized, and 50,000 die.1
Common causes for TBI are falls (28%), motor vehicle accidents (20%), pedestrian impact (19%), and assault (11%). TBI has a bimodal age distribution with the greatest risk in 0–4 and 15- to 19-year-olds. Males have 1.5 times the risk of females. The younger group is often the victim of abuse and cannot protect itself. The older group practices greater risk-taking behavior, and includes the population of new drivers and teenagers exposed to drugs and alcohol. Military personnel comprise a statistically small number of the overall TBI injuries per year, but have a higher incidence of penetrating and blast injuries resulting from combat operations.
PATHOPHYSIOLOGY
TBI is a dynamic process and management must be tailored throughout the patient’s course. Primary injuries of the brain result from the forces imparted at the time of the accident. This includes disruption of scalp (lacerations), bone (cranial vault, skull base, facial bones), vasculature (SDH/EDH/IPH/intraventricular hemorrhage [IVH], traumatic aneurysm), or brain parenchyma (contusion, DAI).
Secondary injuries occur after the initial impact and may be more insidious and more difficult to control. They are often due to failure of autoregulation and loss of normal homeostasis. These injuries include hypoxemia, ischemia, initial hyperemia, cerebral edema, and expansion of hemorrhages leading to increased intracranial pressure (ICP), seizures, metabolic abnormalities, and systemic insults.
GENERAL PRINCIPLES
Systemic Evaluation and Resuscitation
Assessment and treatment of head-injured patients often begins in the prehospital setting with family, bystanders, and off-duty medical personnel. Care continues with the primary care physician or emergency medical technician (EMT), transfers to the physician in the emergency department, and eventually involves the trauma team, neurologist, neurosurgeon, and neurointensivist. Treatments may be started at any point along the patient’s journey based on recognition of neurological signs and availability of appropriate medication, equipment, and personnel.
The nervous system does not exist in a vacuum and patients with TBI may have additional injuries. Treatments specific to TBI are often complementary or adjunctive to the treatment of the trauma patient without neurological injury. The basic principles of trauma resuscitation should be adhered to and include rapid assessment and maintenance of an airway, breathing, and circulation.
A detailed medical and surgical history should be obtained including the events preceding a trauma, a description of the accident scene, accurate description of the patient’s neurological baseline, and any subsequent changes to the neurological status. Chronic medications and medications given in the prehospital setting should be determined. Special attention should be paid to medications with the ability to alter the neurological examination including sedatives or psychopharmacologics (to restrain the altered patient), paralytics (for intubation or transportation), atropine (for cardiac resuscitation), and other mydriatics (for evaluation of ocular trauma).
Primary and secondary surveys should evaluate for systemic injuries including discrete injuries to the head and cervical spine. Open lacerations and vigorous scalp hemorrhage may lead to hypovolemia. In the newborn or premature infant, cephalohematoma may allow enough displacement of blood to produce hemodynamic instability. Raccoon’s eyes (periorbital ecchymosis), Battle’s sign (postauricular ecchymosis), and otorrhea/rhinorrhea suggest a basilar skull fracture. Palpable fractures or depressions may indicate bony injury with a higher likelihood of underlying hemorrhage or parenchymal injury. Periorbital edema or proptosis may suggest local ocular or orbital trauma. Puncture wounds may indicate a more serious, penetrating injury to the brain, spinal cord, sympathetic plexus, or vasculature. Bruits of the carotid artery or globe of the eye may represent carotid dissection or carotid-cavernous fistula, respectively. Multiple areas of swelling or bruising may indicate prior seizure activity.
Neurological Examination
An accurate neurological examination is essential to determine diagnosis, treatment strategies, and prognosis in TBI patients. The exam may be limited due to the patient’s age, level of education, native language, presence of sedative or paralytic medication, illicit drugs, hypotension, hypoxia, hypothermia, or hypoglycemia. Examination of the pediatric patient may include further limitations due to overall neural development and degrees of myelination, inability to visualize the pupils or fundi of the premature or newborn infant, and limited cooperation.
It is critical to monitor the overall trend of the neurological examination over time. It must be understood that these examinations can and will fluctuate based on the patient’s improving or declining condition, the evolution of disease processes, and the ability of medical personnel to minimize or eliminate factors that confound an accurate neurological assessment.
In the uncooperative patient or unconscious patient with severe TBI, the exam may be limited to the Glasgow Coma Scale (GCS), pupillary reactivity, and testing of various reflex actions (Table 19-1). As the patient becomes more alert and cooperative, a more complete neurological examination will provide greater sensitivity for assessment of neurological change. The extent of the examination must be tailored to each patient’s neurological ability.
TABLE 19-1 Neurological Examination for Trauma
Pupillary Response
The parasympathetic, pupilloconstrictor, and light reflex (pupillary reflex) can be easily and rapidly assessed in the unconscious patient. Damage to the Edinger–Westphal nucleus or uncal compression of CN III at the tentorial notch will result in pupillary dilatation (≥4 mm). If severe enough (i.e., cerebral herniation), the pupil will be fixed in this dilated position and is unresponsive to a light stimulus. Direct orbital trauma can also result in pupillary dilation/fixation in the absence of temporal lobe herniation or intracranial hypertension (IC-HTN). It should be considered (quickly) before assuming that a dilated pupil is due to brainstem compression.
In cases of orbital or periorbital trauma, facial fractures, or abnormal eye movement, ophthalmology may examine the patient and wish to instill mydriatics. To avoid confusion, this should only be permitted after concerns regarding elevated ICP have been addressed. Clear notation should be made, in the chart and at the bedside, as to when mydriatics have been used and for how long they will last.
Glasgow Coma Scale
The GCS has become the standard for objective measurement of TBI severity.2 The patient is assessed in three parameters (best motor function [M], best verbalization [V], and best eye opening [E]) and the summation of these individual scores represents their overall GCS score (Table 19-2). A neurologically intact patient can achieve a maximum of 15 points and the most severely injured patient achieves a total of 3 points. If the patient is intubated, he or she receives a score of 1 for the verbal component and the overall scored is annotated with a “T.” For example, an intubated patient with eye opening to pain and extremity withdrawal to pain would have a GCS score of . The same GCS score can be derived from different values of the motor, verbal, and eye components. Therefore, it is beneficial for practitioners to denote each of the subscores and not just the overall number.
TABLE 19-2 Glasgow Coma Scale (Recommended for Age ≥4)
The GCS allows practitioners to communicate quickly and reliably regarding a patient’s general condition. The postresuscitation GCS is also effective in stratifying patients into groups for definition of injury severity and overall prognosis. Patients with are defined as having mild TBI, are usually awake, and have no focal deficits. Patients with
have moderate TBI and usually have altered sensorium and may have focal deficits.
have severe TBI, and usually will not follow commands and meet the generally accepted definition of patients in a comatose state.
As with all neurological assessments, confounding factors must be investigated and eliminated. Paralytics are used for patient transport, restraint, or cardiopulmonary stabilization and will limit the motor exam. Similarly, unrecognized spinal cord injury can produce a lower motor component score of the GCS and should be sought if suggested by the mechanism of injury. Hearing deficits, lack of hearing aids, or impairment of language function may limit the verbal component score. Finally, the pediatric population represents a special subset where modifications to the verbal component score better reflect the limited language skills of the young child3 (Table 19-3).
TABLE 19-3 Glasgow Coma Scale for Children (Recommended for Age <4)
RADIOGRAPHIC EVALUATION FOR TRAUMA
Plain X-Rays
Plain x-rays are most useful in the trauma setting for evaluating and clearing the cervical spine. The spine is imaged from the occiput to T1 and a C-collar is maintained until instability has been ruled out. In the C-spine AP, lateral and odontoid views are the most useful; T- and L-spine films are obtained based on mechanism of injury, degree of neurological deficits, and pain. Additionally, if CT or MRI scans are unavailable, plain x-rays can be used to determine pneumocephalus, skull fractures, and the tract of penetrating objects. It must be remembered that intracranial ricochets of projectiles may occur, and CT is the imaging modality of choice.
CT Scan
Overwhelmingly, the CT scan has become the initial study of patients presenting after head trauma, or with a new neurological deficit. In a single, rapid pass, without patient repositioning, scans of the head, neck, chest, abdomen, and pelvis can be performed. From these, cervical, thoracic, and lumbar spine images can be reconstructed without additional radiation. Administration of contrast allows for CT angiogram reconstruction to evaluate vasculature of the head and neck and be used for diagnosis and operative planning.
CT scan findings after trauma include SDH, EDH, SAH, IPH, and IVH, contusions, hydrocephalus, cerebral edema or anoxia, skull fractures, ischemic infarction (if >12 hours old), mass effect, or midline shift.
Indications for an initial post-traumatic CT scan include , unresponsiveness, focal deficit, amnesia for the injury, altered mental status, and signs of basilar skull fracture.
MRI
MRI scans have better parenchymal resolution and can evaluate infarction, ischemia, edema, and DAI. MRI is also helpful to determine ligamentous injury of the spine or traumatic cord injury. It is generally performed after the initial trauma evaluation and resuscitation have been completed. MRIs have limited availability, slower image acquisition time, and increased cost. Their use in the initial assessment of trauma is not routinely recommended since intracranial surgical lesions seen on MRI are also identified on CT scan.4
Angiography
In penetrating trauma when the tract of injury is near a known vessel distribution or when a delayed intracerebral hemorrhage occurs, angiograms are used to look for direct vessel injury or pseudoaneurysms. When CT or MRI scans are unavailable, angiograms may be used to look for mild vessel shift indicative of compressive mass lesions (e.g., SDH, EDH, IPH). More significant vessel shift may be indicative of transtentorial or subfalcine herniation and suggest the need for more rapid treatment.
CLASSIFICATION AND SURGICAL MANAGEMENT OF SPECIFIC INJURIES
Skull Fractures
Skull fractures can be described by the state of the overlying scalp (closed or open), the number of bone fragments (simple or compound), the relationship of bone fragments to each other (depressed or nondepressed), whether the fracture enters or widens an existing cranial suture (diastatic, more common in children), and whether it involves the cranial vault or skull base. In general, lower force impacts (falls from standing) will create fractures that are more linear, closed, and without dural laceration. Higher force impacts (MVA, falls from heights, penetrating trauma) will produce compound, open fractures with a greater likelihood of underlying dural or cerebral injury. “Ping-pong” fractures are greenstick-type fractures usually seen in newborns due to the plasticity of the skull (Fig. 19-1). They show a local concavity of the skull, without sharp edges, and usually do not require intervention as the skull remodels during growth and smooths out the cosmetic deformity.5
FIGURE 19-1 CT bone windows showing ping-pong skull fracture. The multiple nondisplaced linear lucencies are normal sutures.
Associated clinical signs suggestive of calvarial skull fractures include gross deformity and palpable skull fracture in patients with open scalp lacerations. Basilar skull fractures may show postauricular or periorbital ecchymosis, hemotympanum or laceration of the external auditory canal, and CSF rhinorrhea or otorrhea. Cranial nerve injuries may be seen with fractures of the cribriform plate (CN I, anosmia), optic canal (CN II, visual deficit), and temporal bone (CN VII, facial weakness; or CN VIII, hearing loss). Severe basilar skull fractures may result in pituitary gland injury and resultant endocrinopathies. Direct injury to vasculature that penetrates the skull base may result in arterial dissection, traumatic aneurysm formation, or traumatic carotid-cavernous sinus fistula with symptoms of cranial neuropathies, chemosis, bruits, and strokes. Fractures of air sinuses or mastoid air cells may rarely present with meningitis, even years after the initial event.
Radiographic Diagnosis
Skull fractures can be discovered on isolated plain x-rays or as part of a skeletal survey for abuse. They can be differentiated from vascular grooves or normal cranial sutures by characteristics listed in Table 19-4.6
TABLE 19-4 Differential Diagnosis of Fractures on Skull X-Rays
Most skull fractures are discovered by CT scan due to its overwhelming use in the initial evaluation of trauma patients. Plain films may be superior to CT scan in discovering linear calvarial fractures parallel to the skull base (in the plane of CT slice acquisition). CT scans provide better visualization of facial and orbital fractures, temporal bone fractures, and pneumocephalus, and better evaluation of air sinuses and mastoid air cells containing air fluid levels and varying degrees of opacification. Thin cut bone windows can be reconstructed in coronal or sagittal planes or 3D surface modeling to aid in fracture identification and surgical planning. CT angiograms/venograms are useful for assessing fractures involving skull base foramen containing vasculature (e.g., carotid canal, foramen magnum) or fractures that cross major venous sinuses (superior sagittal or transverse sinuses, jugular foramen).
All fractures must be assessed and treated in concert with the underlying brain. The following discussion of skull fracture treatment assumes that the underlying brain has been evaluated for subdural or epidural hematomas, parenchymal hemorrhages, or contusions and cerebral edema and that clinical criteria do not separately mandate operative decompression.
Closed, nondisplaced fractures do not require immediate intervention. Open skull fractures should be debrided and carefully inspected and all should receive antibiotics. Those with obvious underlying dural laceration, CSF leakage, or visible brain should be surgically repaired in layers to reduce the risks of meningitis or brain herniation through a dural defect. In the pediatric population laceration of the underlying dura can rarely lead to a growing skull fracture (leptomeningeal cyst) seen in 0.05–0.6% of skull fractures.7 Pulsations of the underlying rapidly growing brain widen the dural laceration and fracture line over time (Fig. 19-2). These are most common in children under 1 year and over 90% occur in children under 3 years old.8 Surgical repair includes wide bony exposure to repair the dural edges that retract beyond the limits of the visible fracture.
FIGURE 19-2 CT revealing growing skull fracture from leptomeningeal cyst in child. Note displaced bone and expansion of CSF-filled soft tissue.
Relative indications for surgical elevation of a depressed skull fracture include depression of more than 8–10 mm or more than the thickness of the skull (Fig. 19-3), a focal neurological deficit clearly attributable to compressed underlying brain, significant intraparenchymal bone fragments (implying dural laceration), and persistent cosmetic deformity after all swelling has subsided. There is no evidence that post-traumatic seizure (PTS) risk is improved by elevation of a simple, depressed skull fracture.9 For children, the growing brain induces remodeling of the overlying skull. If there is no dural violation, there is no difference in seizure risk, neurological outcome, or cosmesis afforded by elevation of a simple depressed skull fracture.10 Fractures that cross a major dural venous sinus may warrant a more conservative approach given the increased risk of bleeding and air embolus that may be incurred during surgical repair.
FIGURE 19-3 CT bone windows showing a depressed skull fracture that required surgical elevation and dural repair. The patient also had an underlying brain contusion and presented with a receptive aphasia.
Current recommendations support surgical repair of open fractures depressed greater than the thickness of the cranium and nonoperative management of open depressed cranial fractures if there is no evidence of dural penetration. Surgery is also supported in cases of significant intracranial hematoma, depression >1 cm, frontal sinus involvement, gross cosmetic deformity, wound infection, pneumocephalus, or gross wound contamination. If there is no gross wound contamination, primary bone fragments may be replaced without excessive infection risk.11
Focal Cerebral Injuries
Trauma patients, and their injuries, are hardly uniform. During a single traumatic event, patients may be subjected to forces of different magnitude, direction, and duration. The following injuries are presented as separate discussions for the sake of clarity. It must be remembered that more than one injury type may, and often does, occur in the same patient.
Cerebral Contusion
Cerebral contusions are injuries to the superficial gray matter of the brain caused by a focal force. External forces striking the head cause acceleration of the intact skull or fractured skull fragments toward the brain surface. Conversely, during a motor vehicle accident, the brain continues to move toward the rapidly decelerating skull and dural folds of the falx or tentorium. “Coup” lesions are ipsilateral to the impact site and can be associated with adjacent calvarial fractures. “Contrecoup” lesions are opposite the coup lesion and result from gyral crests of the rebounding brain striking the inner table of the skull.
Fifty percent of contusions involve the temporal lobes with the temporal pole striking the sphenoid wing. Thirty-three percent involve the frontal lobes with impact of the frontal pole or abrasions of the inferior frontal lobes on the rough floor of the anterior cranial fossa. Twenty-five percent are parasagittal, “gliding” contusions caused by abrasions of the cerebral hemispheres along the fixed falx or tentorium cerebelli. Less likely locations include parietal and occipital lobes, cerebellar vermis, brainstem, and cerebellar tonsils. Ninety percent of cases show multiple or bilateral contusions.12
On CT scans, contusions are patchy, hyperdense lesions with a hypodense background. They are best appreciated on MRI where FLAIR imaging shows the hyperintense edematous background and associated SAH and gradient echo (GRE) series show “blooming” of hemorrhagic foci. Contusions may coalesce or enlarge within the first 12 hours and the associated edema will often worsen over the first several days. Vigilant monitoring of the patient with a contusion is essential, and repeat CT scanning is frequently required.
Intraparenchymal Hemorrhage
IPH or traumatic intracerebral hemorrhage (TICH) is seen in up to 8.2% of all TBI and up to 35% of severe TBI cases. Similar to contusions, TICH and associated edema may increase over time and produce increasing mass effect and neurological deterioration. Delayed traumatic intracerebral hemorrhage (DTICH) will appear in approximately 20% of cases and most occur within 72 hours of the initial trauma.13
If patients develop neurological decline referable to the TICH lesion such as IC-HTN refractory to medical treatment or increasing mass effect with impending herniation, surgical decompression is warranted. Investigation of patient subtypes has shown that surgical decompression is often necessary in patients with , or patients with
who have frontal or temporal contusions >20 cm3 with midline shift ≥5 mm and/or cisternal compression on CT scan.11 Surgical procedures range from localized frontal or temporal craniotomy with resection of underlying focal clot to more extensive craniectomies with duraplasty, evacuation of severely contused brain, or temporal lobectomy.
Epidural Hemorrhage
EDH occurs when blood collects in the potential space between the dura and inner table of the skull. It is seen in 1% of all head trauma admissions and in 5–15% of patients with fatal head injuries. It is more common in males , usually occurs in young adults, and is rarely seen in ages <2 or >60 since the dura is more adherent to the inner table of the skull in these groups. Ninety percent of EDHs are due to arterial bleeding that is often due to a fracture at the middle meningeal artery groove, and 10% are due to venous bleeding, usually associated with violation of a venous sinus by an occipital, parietal, or sphenoid wing fracture. EDHs are usually located at the site of impact over the lateral convexity of a cerebral hemisphere (70%), frontal (5–10%), parieto-occipital (5–10%), or posterior fossa locations (5–10%).
On CT scan, EDHs usually appear as a hyperdense, biconvex (lenticular) mass adjacent to the inner table of the skull (Fig. 19-4). This classic description occurs 84% of the time with the medial edge being straight 11% of the time and crescentic (resembling an SDH) 5% of the time.14 Unless there is sutural diastasis, the EDH is externally bounded by cranial sutures, and may cross the falx or tentorium. Additional associated findings include SDHs and cerebral contusions. Of those managed nonsurgically, 23% showed an increase in size, usually in the first 36 hours, with a mean enlargement of 7 mm. Up to 10% of EDHs are not seen on the initial CT scan and present in delayed fashion.15
FIGURE 19-4 CT showing epidural hemorrhage. Note the biconvex- or lenticular-shaped hemorrhage. On the bone windows this was adjacent to a diastatic left lambdoid suture.
The classic clinical presentation of an EDH is a brief post-traumatic loss of consciousness (LOC) followed by a lucid interval, of varying duration, proceeding to obtundation, contralateral hemiparesis, and ipsilateral pupillary dilatation. Interestingly, this only occurs in 27–50% of cases.16 LOC is seen in only 40% of cases, a lucid interval is seen in 80% of cases, a dilated pupil is seen in 60% of cases, and only 85% of these dilated pupils are ipsilateral. Kernohan’s phenomenon (a false localizing sign) occurs when some cases of EDH produce local hemispheric mass effect with compression of the contralateral brainstem against the tentorial notch and ipsilateral hemiparesis.
Overall mortality is 5–12%17 with a unilateral EDH but is increased in cases of bilateral EDH (15–20% mortality), no lucid interval (20% mortality), posterior fossa location (25% mortality), and concurrent acute SDH (25–90% mortality, seen at autopsy in 20% of patients with EDH).
Rapid diagnosis and intervention when indicated is paramount to optimize the outcome. Surgical guidelines suggest that EDH of >30 cm3 should be evacuated regardless of GCS score. EDH of <30 cm3 and <15 mm of thickness and >5 mm midline shift may be treated conservatively at a neurosurgical center with frequent neurological examinations and serial CT scanning.11 Relative indications exist for resection of EDHs that are neurologically symptomatic or have a maximal thickness >1 cm. Patients with acute EDH in coma and anisocoria should undergo surgical evacuation as soon as possible.11 Most surgeons favor craniotomy for complete clot evacuation with meticulous hemostasis and use of tack-up sutures to decrease the potential epidural space.
Posterior fossa injury is rare, comprising <3% of head injuries; however, the majority of these lesions are EDHs. The limited space of the posterior fossa and the potential compromise of the brainstem and CSF pathways underscore the importance of rapid evacuation via suboccipital craniectomy.11 Patients without signs of mass effect or neurological deterioration may be watched conservatively with serial neurological examinations, CT scans, and a low threshold for surgical intervention.
Subdural Hemorrhage
SDH occurs when blood collects between the arachnoid and inner dural layer and is usually divided into hyperacute (<6 hours), acute (6 hours to 3 days), subacute (3 days to 3 weeks), and chronic (3 weeks to 3 months) variants. It is usually due to traumatic stretching and tearing of cortical bridging veins that cross the subdural space and drain into a dural sinus. The force may be direct (impact) or indirect (nonimpact) and may involve linear or rotational motion. Less common etiologies include coagulopathy, subdural dissection of IPH, and rupture of a vascular anomaly (AVM, aneurysm, cavernoma, dural AV fistula) into the subdural space. Patients with cerebral atrophy, cranial CSF shunts, and large arachnoid cysts (usually in the middle fossa) are predisposed to SDH given the increased traction on cortical veins. Following craniocerebral trauma, SDHs are found in 10–20% of all imaged patients and 30% of autopsies. Over 70% of patients with acute SDHs have other significant associated lesions.
SDHs are commonly located over the hemispheric convexities and may cover part or all of a hemisphere (holoconvexity SDH). Classically, they are crescent shaped, cross suture lines, and layer along the falx or tentorium (Fig. 19-5). Patients present with symptoms of mass effect or more diffuse brain injury. Chronic SDH may present with headaches and/or focal deficits.
FIGURE 19-5 CT showing an acute subdural hemorrhage. Note that crescentic hemorrhage crosses under the right coronal suture.
The appearance of SDHs changes with time. On CT, acute SDHs are hyperdense (60%, homogenous) or of mixed density (40%, may show “swirl sign” of active bleeding), subacute SDHs are isodense with brain, and chronic SDHs become hypodense. Some SDHs may be isodense acutely if there is coagulopathy, significant anemia, or an admixture of blood and CSF. There is inward displacement of the gray/white cortical ribbon and cortical vessels on contrast-enhanced CT. Subdural membranes may appear starting at 4 days and enhance with contrast administration.18 MRI shows varying intensities based on the age of the SDH that is, in turn, based on degradation of blood products from oxyhemoglobin to deoxyhemoglobin to methemoglobin to hemosiderin (Table 19-5).
TABLE 19-5 Appearance of SDH on CT and MRI
SDHs often have worse outcomes when compared to EDHs of similar size/shape. EDHs require force directed toward the skull and epidural vessels (i.e., middle meningeal artery), are usually of arterial origin, and present with symptoms quickly allowing for rapid diagnosis and treatment. It is postulated that SDHs have greater force and impart greater damage to the underlying brain and cortical vessels, have slower onset from venous sources, and have symptoms due to primary brain injury in addition to the midline shift and brainstem compression.
Guidelines suggest that an acute SDH with thickness >1 cm or a midline shift >5 mm should be evacuated regardless of GCS score. Patients with acute SDH <1 cm thick and midline shift <5 mm and in coma (GCS ≤8) should undergo SDH evacuation if the GCS decreases by 2 points between the time of injury and hospital admission, if they present with pupils that are asymmetric or fixed/dilated, or if the ICP ≥20 mm Hg.11 A craniotomy to evacuate an acute clot should be performed as soon as possible, and may require craniectomy and duraplasty for ICP control. A larger craniotomy flap is needed for acute clot that has a texture of thick jam. As the clot breaks down, passing through subacute to chronic stages, it liquefies and may require smaller craniotomies or even burr hole access for appropriate drainage.
Mortality ranges from 50% to 90% and may be related more to the underlying injury rather than the SDH itself. It is increased in the elderly and in patients on anticoagulants.19 Outcome is improved in patients operated on in less 4 four hours with mortality improvements from 66–90% down to 30–59%.20,21
Subarachnoid Hemorrhage
SAH is blood located between the pial and arachnoid membranes. Traumatic subarachnoid hemorrhage (tSAH) results from venous tears in the subarachnoid space.
tSAH is seen in 33% of patients with moderate head injury and is found in nearly 100% of trauma patients at autopsy. It can be seen as a sulcal hyperdensity on CT scan (Fig. 19-6) and as a FLAIR hyperintensity on MRI. It may be confused with FLAIR hyperintensities from 100% FiO2, inflammation, or propofol use. It is often seen embedded in convexity sulci or adjacent to contusions, SDH, or fractures. It may mimic aneurysmal SAH, present in the interpeduncular or other basal cisterns or layers on the tentorium. Patients may complain of headache, emesis, and lethargy and treatment is largely supportive using IV fluids, anticonvulsants, and nimodipine, a Ca2+ channel blocker, to prevent vasospasm.
FIGURE 19-6 CT with arrow pointing to a small traumatic subarachnoid hemorrhage in left central sulcus.
Vasospasm involves narrowing or closure of a vessel with subsequent ischemia or infarct in the vascular territory it supplies. It occurs in 2–41% of trauma cases with tSAH, may occur as early as 2–3 days post-trauma, has heightened incidence from days 3 to 14, and may last up to 2–3 weeks after injury.
Diffuse Cerebral Injuries
Concussion
A concussion, or mild traumatic brain injury (MTBI), is defined as an alteration of consciousness resulting from nonpenetrating injury to the brain. Classic symptoms of concussion include headache, confusion, amnesia, and sometimes LOC. Additional symptoms include deficits of motor function (incoordination, stumbling), speech (slowed, slurred, incoherent), memory or processing (amnesia, short-term memory loss, difficulty concentrating or focusing, inattention, perseveration, easy distractibility), and orientation (vacant stare, “glassy eyed,” unable to orient to time/date), and presence of irritability. When present, LOC is usually brief. The two most common grading systems for concussion are from Cantu22 and the American Academy of Neurology (AAN)23 and are shown in (Table 19-6).6
Physiological responses to concussion include a transient increase in cerebral blood volume due to loss of vascular autoregulation. In mild cases, this may result in mild cerebral swelling, or hyperemia. In more severe cases, malignant cerebral edema24 may occur with elevated ICPs refractory to nearly all measures and 50–100% mortality. This is the presumed cause of “second impact syndrome”25,26 seen in child and teenage athletes who suffer a second concussion before fully recovering from their first one. Classically, the athlete walks off the field, suffers LOC in 1–5 minutes, and develops vascular engorgement with further neurological deterioration, cerebral herniation, and death.
CT findings may be subtle or nonexistent and include mild diffuse swelling secondary to hyperemia. Being more sensitive, MRI may show positive findings in up to 25% of cases where CT scans are normal.27 Pathologic specimens show no gross or microscopic parenchymal abnormalities in patients who have suffered a single concussion.
Most important for the treatment of concussion is the recognition of injury. Many concussions occur during athletic endeavors and more subtle signs of concussion might not prompt the layperson to seek medical attention. It is the responsibility of the athlete, coach, team doctor, teacher, EMT, or other medical professional to understand the nature of concussions, prompt the patient to seek medical attention, and initiate restriction of further head-injury-prone activities until the patient has fully recovered. All experts agree that a symptomatic player should not return to play. According to one publication contraindications for return to contact sports28 include persistent postconcussive symptoms, permanent neurological symptoms, hydrocephalus, spontaneous SAH, and symptomatic abnormalities at the foramen magnum (e.g., Chiari malformation).
If none of the above contraindications apply, then various suggestions exist regarding the timing of return to play after evaluation by a medical professional.
AAN management options for a single sports-related concussion23 are listed in (Table 19-7)6 and recommendations for multiple sports-related concussions in the same season29 are listed in (Table 19-8).6
TABLE 19-7 Recommendations for a Single Sports-Related Concussion
TABLE 19-8 Recommendations for Multiple Sports-Related Concussions in the Same Season
Diffuse Axonal Injury
DAI is a traumatic axonal stretch injury caused by overlying cerebral cortex and underlying deep brain structures moving at different relative speeds. Mild cases result in axonal stretching and transient neuronal dysfunction while more severe cases cause axonal shearing and permanent neuronal damage. DAI does not require head impact and may be caused by rapid acceleration or deceleration in a linear or rotational fashion.30
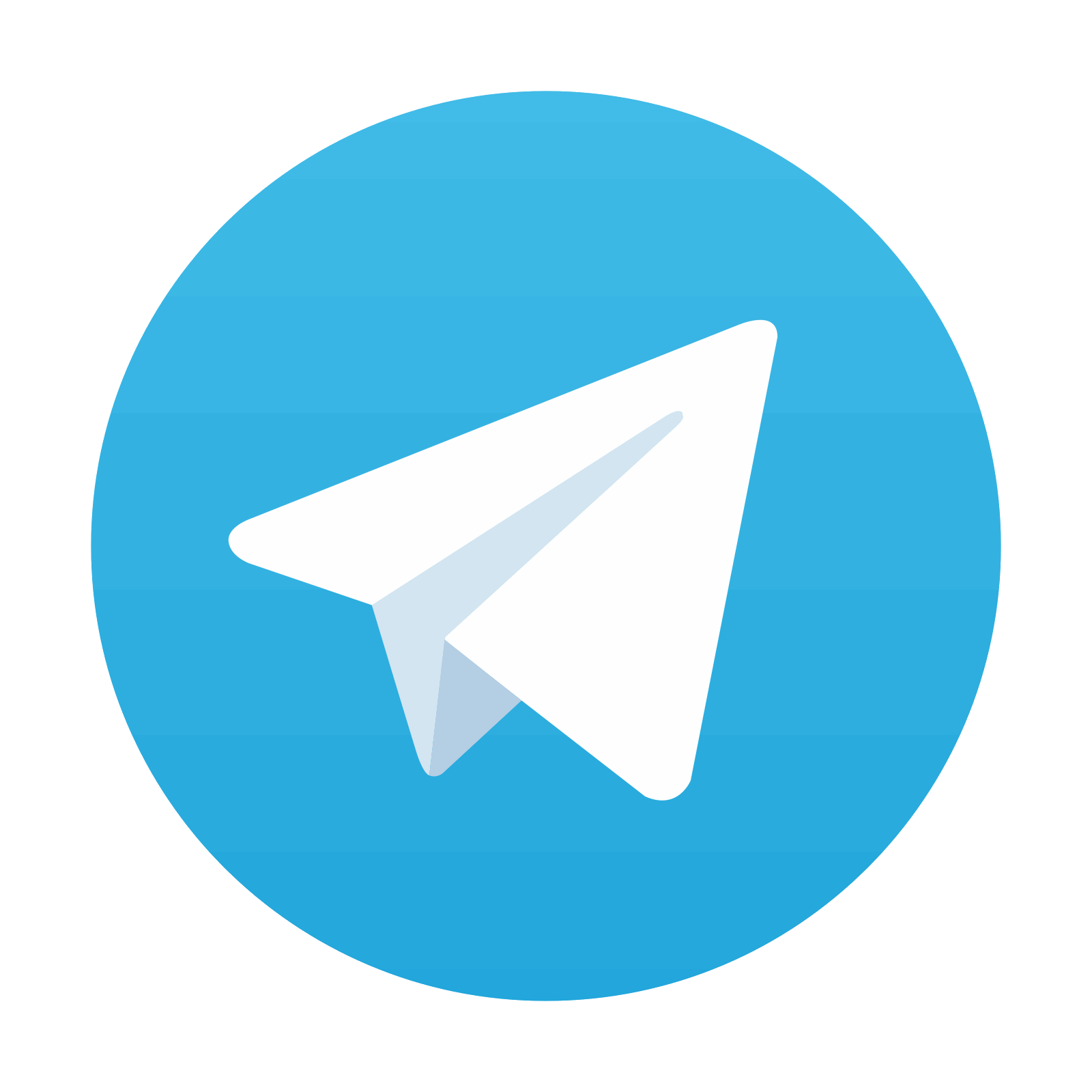
Stay updated, free articles. Join our Telegram channel

Full access? Get Clinical Tree
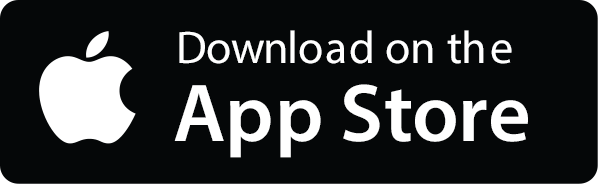
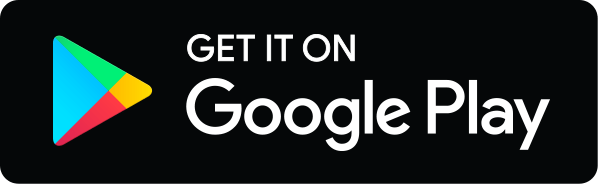