Fig. 5.1
The Renin-Angiotensin-Aldosterone System. The major components of RAAS are shown in the above figure. As depicted, an increase in plasma renin levels initiates the cascade. Multiple organ systems are involved (Reproduced with permission of Informa Healthcare, Haroonur [4], © 2008, Informa Healthcare. Reproduced with permission of Informa Healthcare)
Activation of the Renin-Angiotensin-Aldosterone System
The RAAS is activated in heart failure. The exact mechanisms by which activation occur are yet to be fully elucidated. Most likely, though, the combination of decreased perfusion to the kidney, decreased cardiac output, and low arterial pressure activate RAAS.
As a compensatory mechanism to decreased cardiac output, renin is released from the kidneys and is responsible for converting angiotensinogen to angiotensin I. Angiotensin I is then converted to angiotensin II by angiotensin-converting enzyme (ace). Angiotensin II stimulates the receptors AT1 and AT2.
Heart failure is defined as the pathological state in which the heart is unable to pump blood to adequately supply tissues to affect metabolism, or can do that but only with an increase in filling pressures. In the presence of an acute or subacute insult to the myocardium, which effects contractility, the heart depends on adaptive strategies to maintain pumping ability and cardiac output. Among the most important mechanism is activation of neurohormonal systems, including the sympathetic nervous system and the RAAS. The activation of RAAS can occur rapidly, over minutes to hours, to maintain cardiac function. The RAAS does this acutely by retaining salt and water to augment preload or cardiac filling. Additionally, RAAS activation can cause vasoconstriction, to help perfuse organs and tissues. Although, these mechanisms are adaptive in the short term, they are maladaptive in the long term.
As a result of activation of the RAAS, sympathetic nervous system and release of norepinephrine, myocardial hypertrophy and remodeling develop over weeks, months and years. With chronic activation of these pathways, these mechanisms become maladaptive. Although able to sustain cardiac function for some time, high filling pressures over time will ultimately cause worsening cardiac performance effecting organ perfusion and circulation. Many of the symptoms of heart failure, lower extremity swelling, dyspnea, orthopnea, paroxysmal nocturnal dyspnea, abdominal bloating are a consequence of the retention of fluid causing high filling pressures.
When the RAAS is activated in low cardiac output states, it is closely associated with the adrenergic system to maintain arterial pressure. Stimulation of beta1 adrenoceptors in the juxtaglomerular apparatus of the kidneys is a principle activator of renin release in acute heart failure. Fig. 5.2 shows the activation of the Renin-Angiotensin-Aldosterone System [5] .


Fig. 5.2
Activation of the Renin-Angiotensin-Aldosterone System. Scheme picturing the primary hemodynamic mechanisms by which the RAAS is activated (Reproduced from Jackson et al. [5], © 2000, with permission from BMJ Publishing Group Ltd)
Aldosterone is predominantly produced by the adrenal glands and, to a lesser degree, the endothelial and vascular smooth muscle cells in the heart and blood vessels. Aldosterone is regulated by the RAAS as well as potassium levels. Angiotensin II is the principal stimulator of aldosterone in the adrenal cortex [6]. Plasma levels of aldosterone can increase dramatically in patients with heart failure. This increase is attributed mainly to increased synthesis of aldosterone in response to activation of RAAS.
Aldosterone has been implicated in several harmful effects contributing to heart failure. Aldosterone vasoconstricts and also potentiates catecholamines. It upregulates inflammatory mediators such as macrophages, cytokines and chemokines. Aldosterone also plays a role in myocyte necrosis and scarring. Chronically elevated aldosterone levels contribute to myocardial fibrosis and deleterious remodeling. More recent studies have shown that aldosterone promotes platelet aggregation and activation [7, 8]. For these reasons, decreasing aldosterone levels with aldosterone inhibitors remain a therapeutic target and goal of modern heart failure therapy.
Treatment with ace-inhibitors results in an acute reduction in aldosterone levels. In chronic ace-inhibition, the reduction in aldosterone levels is weaker and more variable. This finding is referred to as aldosterone “escape” [9].
Effects of RAAS Activation
The actions of RAAS are beneficial in some circumstances. In heart failure, though, RAAS is chronically activated and results in deleterious effects. Chronic activation and up regulation of RAAS causes increased circulating angiotensin I, angiotensin II and aldosterone. Acute activation of RAAS maintains vascular tone or vasoconstriction. As a result, renal function is also maintained in the short term. In addition, cardiac output is preserved and left ventricular filling pressures are reduced.
Chronic activation of RAAS results in maladaptive remodeling of the myocardium. This includes fibrosis formation and myocyte hypertrophy.
Angiotensin II has several effects that contribute to the syndrome of heart failure and the progressive nature of left ventricular dysfunction. In particular, angiotensin II promotes hypertension, atherosclerosis and hypertrophic changes in the walls of arteries as well as the left ventricle [10].
Aldosterone promotes the retention of sodium and the loss of magnesium and potassium. Aldosterone also promotes sympathetic activation, parasympathetic inhibition myocardial and vascular fibrosis. Table 5.1.
Table 5.1
Major actions of RAAS components
Chemical | Actions |
---|---|
Aldosterone | Cardiac fibrosis, sodium and water retention, magnesium and potassium loss, vasoconstriction, sympathetic activation, parasympathetic inhibition, impairs arterial compliance, platelet activation and aggregation, activates macrophages, stimulates growth of fibroblasts and synthesis of collagen |
Angiotensin converting enzyme | Proliferative and proinflammatory signaling |
Angiotensinogen | Releases Angiotensin II, substrate for renin |
Angiotensin I | Largely inactive precursor to angiotensin II, weak vasoconstrictor |
Angiotensin II | Arterial vasoconstriction, pro-inflammatory, salt and water retention by the kidneys, stimulation of thirst, release of anti-diuretic hormone, augments norepinephrine release, remodels heart and blood vessels, induce cardiac myocyte hypertrophy, stimulates aldosterone, vasopressin, catecholamines and endothelin |
Renin | Activates RAAS by cleaving angiotensinogen |
Bradykinin | Vasodilator, anti-proliferative, cough, angioedema |
Inhibition of Raas
Earlier segments of this chapter described activation of RAAS and the detrimental effects that activation of RAAS has on the ailing and/or failing heart. The rationale for combination therapy is to inhibit the production of angiotensin II, the major hormone involved in RAAS, at every step. Angiotensin II has several harmful effects. The reduction of angiotensin II by inhibiting RAAS activation is responsible for the many positive effects of ace-inhibition, angiotensin receptor blockade and aldosterone inhibition.
The current standard therapy for heart failure and cardiomyopathy is designed to interrupt the RAAS and the sympathetic nervous system (SNS) by using a combination of medications. Multiple trials have demonstrated the beneficial effects of using medications that target different hormones. Pharmacologic approaches to blocking the effects of angiotensin II, have been aimed at decreasing its production by inhibiting angiotensin converting enzyme or by direct inhibition at the level of the angiotensin receptor 1.
Ace-Inhibition
An angiotensin-converting-enzyme inhibitor (ace-inhibitor) is considered the first line therapy in treating patients with heart failure and left ventricular dysfunction. Ace-inhibitors have shown improved survival in patients with mild to severe chronic heart failure.
Ace-inhibitors block the conversion of angiotensin I to angiotensin II and prevent the degradation of bradykinin. The efficacy of ace-inhibition is due not only to blocking the harmful effects of angiotensin II, but also due to the promotion of bradykinin, a vasodilator. It is the bradykinin that causes some of the side effects of ace inhibitors (angioedema and cough). Ace-inhibitors also block the negative feedback in renin release by decreasing renin release and the rate of angiotensin I formation. Studies have shown multiple (pleiotropic) effects of ace-inhibition.
Ace-inhibitors have been shown to decrease left ventricular hypertrophy and smooth muscle proliferation. They decrease coronary vasoconstriction and have anti-macrophage effects. Ace-inhibitors also have been shown to stabilize arterial plaque. In addition, ace-inhibitors also prevent ventricular remodeling. This has been shown clinically in patients with hypertension, symptomatic heart failure, elderly patients with heart failure and patients status-post myocardial infarction [11, 12].
Angiotensin Receptor Blockade
Angiotensin Receptor Blockers or ARBs have their effect further down the RAAS cascade. Unlike ace-inhibitors, ARBs have little effect on bradykinin, therefore no cough or angioedema. ARBs block angiotensin II at the receptor site.
While initiation of ace-inhibitors lead to a rapid decline in circulating angiotensin II, chronic use of ace-inhibitors can lead to an increase in angiotensin II, from initial decline. This increase in angiotensin II or “ACE escape” is thought to be due to conversion of angiotensin I to angiotensin II by means other than ACE. ARBs would avoid this phenomenon, based on their mechanism of action. In theory, ARBs would have a more complete blockade of angiotensin II [13].
ARBs have shown benefit in patients that are intolerant to ace-inhibitors or as an alternative to ace-inhibitors. The addition of ARBs to ace-inhibitors has shown benefit in decreasing hospital admissions, their benefit may be attenuated by increased risk of hyperkalemia. In patients intolerant to ace-inhibitors, valsartan, losartan and candesartan have shown benefits in mortality reduction [14–16].
Aldosterone Inhibition
Since the RALES trial was published in 1999, aldosterone inhibitors have been used for patients with heart failure. In the RALES trial, patients were assigned to receive aldosterone inhibitor (spironolactone) 25 mg daily or placebo. To enter the trial, patients had to have ejection fraction < 35 % and NYHA III–IV heart failure symptoms. The trial was stopped early due to significant benefits in the spironolactone arm. There was a 30 % reduction in deaths in the spironolactone group. This was due largely to a decreased risk of progressive heart failure and a decrease in sudden cardiac death [17].
Aldosterone inhibitors block the effect of aldosterone by binding to mineralocorticoid receptors. The two major aldosterone inhibitors are spironolactone and eplerenone. Spironolactone is a non-selective mineralocorticoid receptor antagonist. It also up-regulates progesterone receptors and down-regulates androgen receptors. Some of the side effects of spironolactone-gynecomastia, changes in libido and menses-are due to this effect. Spironolactone also causes hyperkalemia, which needs to be monitored closely.
Eplerenone is a selective mineralocorticoid receptor antagonist. It has fewer side effects than Spironolactone. Importantly, it can cause hyperkalemia as well, and must be also monitored closely.
Aldosterone can stimulate angiotensin II production, leading to fibrosis. The myocardium has mineralocorticoid receptors. The amount of aldosterone produced is proportional to the degree of heart failure.
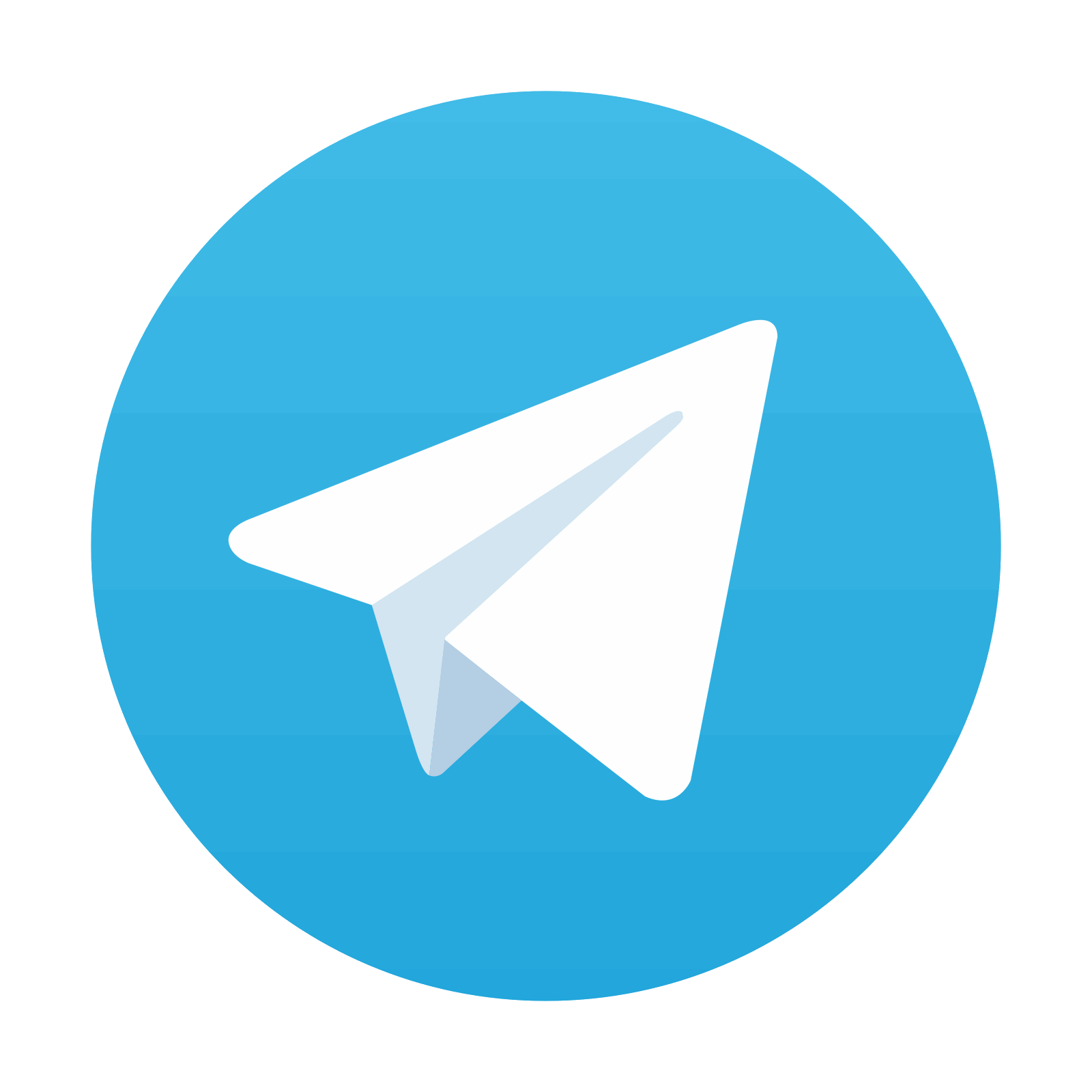
Stay updated, free articles. Join our Telegram channel

Full access? Get Clinical Tree
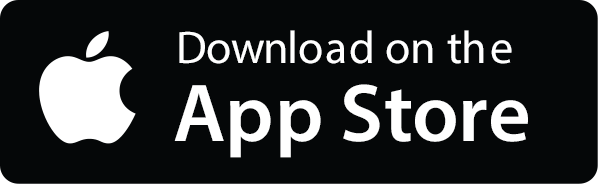
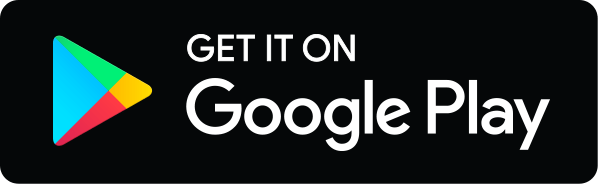