© Springer International Publishing Switzerland 2015
Kristina Orth-Gomér, Neil Schneiderman, Viola Vaccarino and Hans-Christian Deter (eds.)Psychosocial Stress and Cardiovascular Disease in Women10.1007/978-3-319-09241-6_1212. Inhibited Breathing and Salt-Sensitive Hypertension in Women
(1)
Division of Nephrology, Department of Medicine, University of California, San Francisco, CA, USA
(2)
Osher Center for Integrative Medicine, Division of Medicine, San Francisco, CA, USA
Abstract
Psychosocial and dietary factors can contribute to the development of primary hypertension, but the physiological mechanism mediating this most common cardiovascular disorder remains elusive. It is often assumed that the effects of stress are mediated by the sympathetic nervous system, and an interaction between high sodium intake and behavioral stress has been documented in the development of sustained hypertension in animal studies. A growing literature suggests, however, that stress-induced suppression of respiration may also play an important role, particularly in women. Specifically, in older women, salt sensitivity of blood pressure and resting blood pressure level are both associated with high resting levels of pCO2—an important respiratory variable because it reflects the relationship between breathing and metabolic activity, and is a determinant of plasma acid–base balance. Studies are needed to assess the extent to which training in deep breathing can reset resting pCO2, and prevent or reverse salt-sensitive forms of hypertension, especially in women.
Keywords
Blood pressureBreathingCarbon dioxideHypertensionRespirationStressPrimary hypertension is the most common cardiovascular disorder and a major risk factor for cardiovascular disease (Kannel 1996). Much is known about the nature and time course of the hemodynamic derangements that constitute hypertension (Lund-Johansen 1994; Guyton 1989; Izzo et al. 2008), but its ultimate environmental and behavioral causes continue to evade clarification. That hypertension is a “disease of civilization” is clear from epidemiological findings that some pre-industrial societies show virtually no increases in blood pressure with age, and little or no incidence of hypertension (Poulter and Sever 1994). The mechanisms mediating the interactive effects of environmental stresses and dietary influences on blood pressure need to be clarified, so it will be possible to develop behavioral interventions that can prevent or reverse hypertension on a rational basis.
That such interventions are needed is clear from the costs and side effects of antihypertensive medications, and the challenges associated with adherence to them (Linden & Moseley 2006). Moreover, the results of clinical trials of antihypertensive medications do not extend to long-term benefits. For example, in 20,000 patients followed for 10 years, treatment with antihypertensive medication did not decrease mortality rates, but was actually associated with greater mortality in all but those with the most malignant hypertension (Thürmer et al. 1994). In any event, while these medications can lower blood pressure, they do not address the preventable origins of hypertension. The present chapter suggests that an inhibited breathing pattern is the key to understanding how salt sensitivity of blood pressure emerges that can lead over time to the development of chronic hypertension. It will be proposed that an ambulatory breathing monitor that can detect variations in end tidal CO2 (PetCO2) might provide a means to prevent or reverse salt-sensitive forms of hypertension, especially in women.
Gender differences in blood pressure regulation have been identified that provide differential baselines from which to look for differential causes and mechanisms of hypertension in men and women. At rest, premenopausal women exhibit less sympathetic influence on the heart and peripheral vasculature than age-matched men (Reckelhoff 2001; Sanada et al. 2001). Accordingly, the sympathetically mediated cardiovascular response to mental arithmetic and other behavioral stressors is less marked in women than in men (Lawler et al. 1995; Steptoe et al. 1996). Sex hormones have differential effects on the vascular system. Estrogen decreases vascular tone in women via greater nitric oxide production than occurs in men (Barnett et al. 1999). Conversely, testosterone stimulates the renin–angiotensin system to increase plasma renin activity and vascular tone in men (Evans et al. 2001). Resting blood pressure of premenopausal women tends to be lower than that of men, and they have a lower incidence of hypertension (Gudmundsdottir et al. 2012).
After menopause, when estrogen levels are diminished, blood pressure of women increases to levels comparable to those in men, and their incidence of hypertension becomes comparable to that in men (Kotchen et al. 1982; Whelton et al. 1994). Longitudinal studies have shown that the rise in hypertension incidence does not occur immediately after menopause, but is delayed by a decade or more (Oparil 2006). Thus, it is unlikely that the loss of estrogen is a sufficient cause of increased hypertension in older women.
Blood pressure of both men and women tends to increase with age, and hypertension tends to run in families, so structural biologists seek to identify genes that predispose to hypertension (Weder 2008). Rare forms of hypertension have been linked to specific genes, but geneticists have concluded that hypertension is only “moderately heritable” (Kelly and He 2012). By contrast, epidemiological studies have shown that industrialized societies have higher rates of hypertension than pre-industrial ones (Whelton et al. 1994), and that migration from a rural to an urban environment increases the prevalence of hypertension (Poulter and Sever 1994). As a result, functional biologists have focused more on the environmental, behavioral, and dietary determinants of hypertension.
Psychological Determinants of Hypertension
As long ago as the 1930s, it was postulated that hypertension was a “psychosomatic” disorder; that is, emotional processes were involved in the pathogenic process, and in this case, an inhibition in the expression of anger (Alexander 1939). Over the ensuing decades, the preponderance of evidence from personality studies supported the conclusion that hypertensive patients are characterized by “repression of anger” (Jorgensen et al. 1996; Mann and James 1998), “submissiveness” (Perini et al. 1994), or a flatness of affect termed “alexithymia” (Jula et al. 1999). This personality characteristic is in marked contrast to the anger and hostility that constitutes the core of the Type A personality, which has been implicated in the development of coronary heart disease (Friedman and Rosenman 1974; Chesney et al. 1988; Williams and Barefoot 1988).
The original psychosomatic hypothesis directed attention to early experience that molds individual personality. But evidence is also available that pervasive environmental stresses in adulthood can contribute to the development of hypertension. For example, higher blood pressure levels have been observed in men living in high stress urban neighborhoods than in age- and race-matched men living in lower stress environments (Harburg et al. 1973). Conversely, nuns living in seclusion showed no rise in resting blood pressure over a 20-year period, while blood pressure of a control group of women residing in urban settings did increase (Timio et al. 1988). This latter study was distinguished by the fact that no group differences in diet, body weight, body mass index, family history of hypertension, or serum cholesterol levels were observed.
Experimental studies of laboratory stress effects have reported that hypertensive subjects tend to show larger, sympathetically mediated increases in blood pressure and heart rate than normotensive subjects (Matthews et al. 1986). These findings suggested that hypertensive patients may have been characterized by “cardiovascular hyper-reactivity” before they became hypertensive, and that this “trait” played a role in the pathogenic process (Frederickson and Matthews 1990). Some (Matthews et al. 1993; Light et al. 1999; Ming et al. 2004) though not all (e.g., Fauvel et al. 2003) studies reported that people with high reactivity are more likely to become clinically hypertensive in subsequent years. However, cardiovascular reactivity to laboratory stressors was found not to be consistent across tasks or predictive of blood pressure variability in the natural environment (reviewed by Pickering 1994). The hyper-reactivity theory was thus stalled in semantic and operational complexities regarding the nature and stability of reactivity itself.
Attempts were also made to generate chronic hypertension in laboratory animals exposed to residential stresses in aversive environments, and some successes were reported with mice and rats (Brown et al. 1999; Folkow 1982; Henry 1994). Attempts to develop chronic hypertension in larger animals via behavioral conditioning of sustained sympathetic arousal were less successful, and generally did not support the hypothesis that sympathetic arousal sustained over even many months by behavioral conditioning is sufficient to generate hypertension in large animals. The sympathetic arousal theory of hypertension pathogenesis has retained its appeal for some, however, due to the fact that people in the early stages of hypertension often show increases in indices of efferent sympathetic nervous system activity (Brown et al. 2000; Grassi 1998).
Salt Sensitivity of Blood Pressure
Epidemiological studies have found that societies with higher dietary sodium intake show larger increases in blood pressure than those with lower dietary sodium intake (reviewed by Muntzel and Drueke 1992). In some pre-industrial societies with diets high in fruits and vegetables, virtually no increase in blood pressure with age occurs. Experimental studies have investigated the acute effects of changes in dietary salt intake on blood pressure; generally, sodium loading in normotensive subjects and sodium restriction in hypertensive subjects. Unless salt intake is extremely high, no group changes in blood pressure occur within days (Miller et al. 1987). However, a spectrum of blood pressure responses are observed within a group, with some persons showing an increase in blood pressure to sodium loading or a decrease to sodium restriction.
This has resulted in the concept of the salt-sensitive individual. Salt sensitivity of blood pressure is more prevalent among older than younger persons (Weinberger et al. 1986), among women than men (Nestel et al. 1993), and among Afro-American than Euro-American subjects (Grim et al. 1980). However, sodium loading itself decreases sympathetic nervous system activity (Muntzel and Drueke 1992), increases heart rate variability (McNeely et al. 2008), and decreases plasma renin activity (Young & McCaa, 1981). It also increases circulating concentrations of asymmetric dimethylarginine in salt-sensitive but not salt-resistant Euro-American (Scuteri et al. 2003) and Afro-American subjects (Schmidlin et al. 2007). This compound is an inhibitor of nitric oxide, an endogenous vasodilator that is decreased in hypertensive humans (Hermann et al. 2006). Thus, salt-sensitive individuals do not exhibit the compensatory vasodilation in response to sodium loading that occurs in salt-resistant persons.
A psychological component of salt sensitivity was revealed in research showing greater emotional responsiveness in salt-sensitive than salt-resistant subjects during acute mental stress (Deter et al. 1997). Salt-sensitive subjects also showed more pronounced startle reflexes to acoustic stimuli than salt-resistant subjects (Buchholz et al. 2001). The extent to which this attribute is innate or acquired remains to be clarified, but as will be detailed in the next section, salt sensitivity of blood pressure can be generated in genetically normotensive laboratory animals by exposure to specific kinds of behavioral stress.
Inhibited Breathing in Salt-Sensitive Hypertension
Coleman and Guyton (1969) showed long ago that when large quantities of saline were infused into healthy, intact dogs, they excreted the sodium, maintained a stable body sodium balance, and showed no changes in blood pressure. A subsequent series of studies showed, however, that salt sensitivity of blood pressure could be created in healthy dogs by behavioral stress; specifically, progressive 24-h hypertension developed over periods of days when saline was infused into subjects that had previously been trained to perform avoidance tasks (reviewed by Anderson 1987). Twenty four hr blood pressure did not increase when saline was infused into subjects with no behavioral stress, or in response to repeated performance of the behavioral task under conditions of normal sodium intake. Thus, the effects of the two procedures were synergistic, not additive. The development of the experimental hypertension was accompanied by renal sodium retention and increased plasma volume over the first few days, resulting in a new, higher stable level of total body sodium. A progressive 24-h hypertension developed over the next 7 days or so. This hypertensive adaptation could be sustained over periods of weeks and months as long as the sodium intake was increased.
It was expected that the sodium retention would be shown to be a consequence of increased activity of the sympathetic nervous system and/or the pituitary–adrenocortical system. However, the development of the hypertension was not prevented by either renal denervation or pharmacological blockade of sympathetic activity via adrenergic blocking drugs. Moreover, plasma renin activity and plasma aldosterone were decreased during the application of the stress/salt conditioning. Thus, no support was obtained for the hypothesis that this form of experimental hypertension was mediated by sympathetic arousal or increases in salt-retaining hormones.
During the intervals of hours before the onset of each avoidance session, however, a preparatory cardiorespiratory pattern emerged each day that could have played a decisive role in the hypertensive adaptation. This pattern consisted of gradual decreases in breathing rate below resting levels (with no compensatory increases in tidal volume) that intensified as the onset of the next task approached. Subsequent studies showed that pCO2 increased above resting levels as breathing rate decreased, while hematocrit also decreased, indicative of expansion of plasma volume (Anderson et al. 1996b). It is known that a suppression of breathing that elevates pCO2 dilates cerebral blood vessels and increases cerebral blood flow, and could thereby contribute to increased alertness (Grossman 1983; Pardo et al. 1991). That the subject was alert at the time of session onset was signaled by the fact that avoidance behavior was reliably initiated promptly after each session started. If the avoidance tasks were scheduled randomly with respect to time, however, no such pattern of inhibited breathing developed.
Hypercapnic breathing of the kind that occurred preceding the onset of avoidance tasks has been studied in people. Given the dilation in cerebral vessels that occurs when pCO2 increases, it may be surprising to find that voluntary inhibition of breathing elevates human blood pressure (reviewed by Anderson 1998). For example, when subjects increased their PetCO2 4–5 mmHg above resting levels by breathing into a respiratory gas monitor that gave them digital breath-to-breath feedback, systolic and diastolic pressure increased by about 10 mmHg with no increases in heart rate. Blood pressure was increased regardless of whether the PetCO2 was elevated by low frequency/normal tidal volume breathing or by low tidal volume/normal frequency breathing. Thus, it was the changes in pCO2 rather than mechanical aspects of breathing that increased the blood pressure level. It should be emphasized that the use of the term hypercapnic in this context means relative to preceding levels, but not above those levels that trigger chemoreceptor reflexes to stimulate breathing and wakefulness in sleeping persons.
Mild hypercapnic breathing of this kind also elicits increases in digitalis-like circulating compounds that inhibit Na+/K+-ATPase and increase vascular tone (Bagrov et al. 1995), providing one possible mechanism whereby hypercapnic breathing might elevate blood pressure acutely. It is interesting, therefore, that higher concentrations of digitalis-like compounds have been found in the blood of hypertensive humans than in normotensive controls (DeWardener and Clarkson 1985).
When the same persons decreased PetCO2 below resting levels, that is, engaged in hypocapnic breathing, the opposite effect was observed; that is, blood pressure decreased. The sequence of presentation of the breathing tasks is critical to the observed cardiovascular effects. If hypercapnic breathing occurs first and hypocapnic breathing second, blood pressure first increases, and then decreases. However, if the sequence of breathing tasks is reversed, a different result is obtained. Hypocapnic breathing decreases blood pressure as before, but hypercapnic breathing does not then increase it. Apparently, some neurochemical compound(s) that dilates blood vessels locally must persist over a period of time after the cessation of the hypocapnic breathing.
It has been known for many years that voluntary deep breathing can decrease blood pressure acutely. The depressor response is not accompanied by changes in muscle sympathetic activity (Anderson et al. 1991), and can even be observed following total surgical sympathectomy (Burnum et al. 1954). The reality of this phenomenon is further evidenced by the fact that some clinicians have advised that patients refrain from deep breathing exercises prior to clinic blood pressure measurement because of the likelihood of producing “artificially” low levels (Mori et al. 2005).
Resting PetCO2 and Salt Sensitivity of Blood Pressure
The textbook value of arterial pCO2 in healthy humans is 40 mmHg. The concentration of CO2 in expired air (PetCO2) is on average about 3 mmHg below pCO2. Breath-to-breath variations in PetCO2 closely follow those observed in pCO2. Individual variability in resting PetCO2 has been identified in a large sample of humans in the Baltimore Longitudinal Study on Aging (Dhokalia et al. 1998). The population distribution approximates a normal curve, skewed to the right, with a modal value of about 37 mmHg, and a range between about 30 and 40 mmHg. Individual values tend to be stable over time, though test–retest correlations are lower after 6 months than they are after 6 days. People who tend to maintain higher resting PetCO2 tend to breathe more slowly than those with lower resting PetCO2, while those who maintain lower resting PetCO2 tend to breathe more deeply than those with higher resting PetCO2 (Grossman 1983).
Resting PetCO2 of young men is higher than that of young women. However, PetCO2 of men decreases significantly over the life-span, while women maintain their levels, so that after age 65, PetCO2 of women is as high as or higher than that of men (Dhokalia et al. 1998). Comparable age-associated changes in blood pCO2 have been found by Frassetto and Sebastian (1996). Resting PetCO2 is higher in Afro-Americans than Euro-Americans (Anderson et al. 2001). Finally, high resting PetCO2 has been associated with higher scores on the Neuroticism Scale of the NEO Personality Inventory, which measures the tendency to worry (Dhokalia et al. 1998). High resting PetCO2 in women over age 50 has been associated with higher resting systolic blood pressure than in those with low resting PetCO2 (Anderson et al. 1999). This association was more marked in women with low scores on the trait anger and anger-out components of the Spielberger Anger Expression Inventory (Scuteri et al. 2001). No such associations were observed in men.
Experimental studies with normotensive humans maintained on low salt diets for 7 days followed by high salt diets for 7 days have shown that both slow breathing rate (Anderson et al. 2007) and high resting PetCO2 (Anderson et al. 1996a) are predictors of salt sensitivity of blood pressure. These effects are more marked in women than men, and especially older women. It seems reasonable to speculate that a habitual pattern of inhibited breathing that increases the set point at which pCO2 is maintained is a risk factor for salt sensitivity of blood pressure and sodium-dependent hypertension in older women. Inhibited breathing that increases pCO2 acidifies the plasma transiently (Anderson 1998) and salt-sensitive humans have lower plasma pH than salt-insensitive humans (Sharma et al. 1993). Increased plasma acidity signals the kidneys to increase H+ excretion even at the cost of increased retention of sodium and water that expands plasma volume (Rosskopf et al. 1993; Siffert and Dusing 1995).
Inhibited Breathing in Ambulatory Humans
Breathing normally varies in proportion to metabolic need for oxygen, with increases in ventilation during aerobic exercise and emotional excitement, and decreases during sleep. Previous research with healthy humans has shown substantial differences between individuals in the relative contribution of breathing rate and depth (tidal volume) to ventilation at rest. Some humans tend to breathe more rapidly and shallow, while others tend to breathe more slowly and deeply (Grossman 1983). Fast breathers tend to report higher anxiety levels than slow breathers (Blechert et al. 2013). However, slow breathers who do not breathe more deeply report higher levels of chronic perceived stress than faster breathers (Anderson and Chesney 2002). Considerable stability has been reported in these individual differences in breathing style (Shea et al. 1987), which can persist over periods of years (Benchetrit et al. 1989).
While human breathing has been investigated extensively in laboratory settings (Tobin et al. 1983), noninvasive monitoring of respiration in the natural environment has posed significant technical challenges, which may account for the relative lack of experimental attention to breathing patterns in stress-related disorders. Assessment of breathing rate has long been available via bands around the chest, but it was not until the introduction of respiratory inductive plethysmography that breath-to-breath tidal volume became available (Sackner et al. 1980). By connecting a wearable microprocessor to a tiny electrical current passed through elasticized bands around the thorax and abdomen, it became possible to assess tidal volume in ambulatory humans over periods of hours (Anderson and Frank 1990). Studies with the device built on what had been learned in laboratory settings (reviewed by Anderson 1998). Breathing rate tended to be on average about the same during the daytime as at night, while tidal volume was substantially lower at night. In addition, occasional episodes of inhibited breathing were observed during the daytime that were characterized by subnormal rates and normal tidal volume. These episodes were more likely to be observed in social situations (e.g., at work) than when subjects were alone (e.g., at home). Systolic blood pressure tended to be higher during episodes of inhibited breathing in the natural environment. Ultimately, a similar device was integrated in a commercially available vest that could monitor multiple physiological functions concurrently (Wilhelm et al. 2003). Meanwhile, studies with seated hypertensive humans had reported a greater incidence of episodic breathing inhibition than was observed in normotensive controls (Novak et al. 1994).
Behavioral Interventions in Hypertension
The preceding discussion has focused on inhibited breathing as part of a psychophysiological state that can contribute to the development of hypertension in women. However, attempts to treat hypertension via behavioral interventions have not evolved from this orientation. Rather, they focused on interventions presumed to reverse a state of sympathetic nervous system arousal thought to maintain blood pressure at an elevated level (Dickinson et al. 2008). The methods employed have included various forms of relaxation, meditation (focused attention, transcendental meditation, and contemplative meditation), yoga, cognitive behavioral therapies, guided breathing exercises, biofeedback, stress management, and others.
< div class='tao-gold-member'>
Only gold members can continue reading. Log In or Register a > to continue
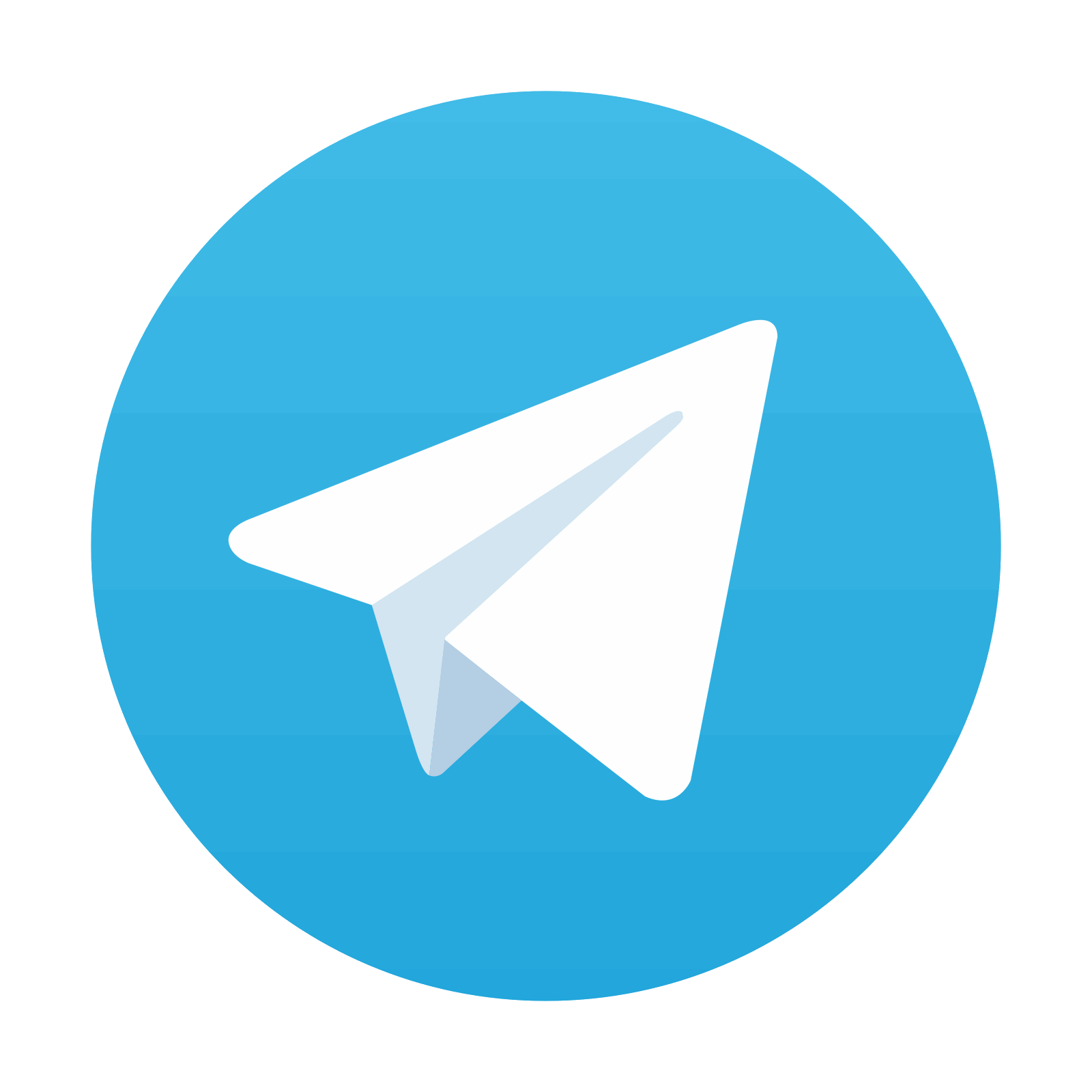
Stay updated, free articles. Join our Telegram channel

Full access? Get Clinical Tree
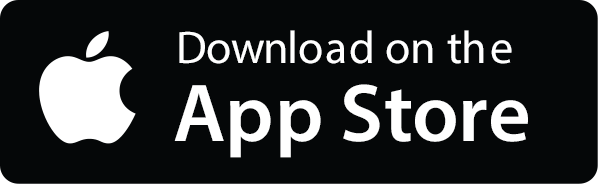
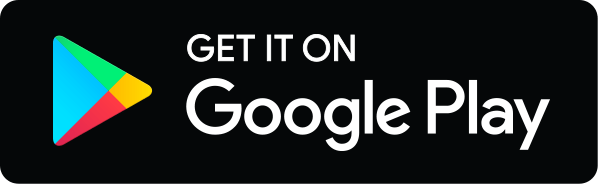