Type of CHD
Father with CHD
Mother with CHD
Recurrence risk for offspring (%)
Affected births/live births among offspring
Recurrence risk for offspring (%)
Affected births/live births among offspring
TOF
Chin-Yee et al. [14]
3.4
6/179
6.3
11/174
Burn et al. [15]
1.6
2/124
4.5
6/132
Nora [16]
1.5
2.5
Drenthen et al. [2]
3
6/202
APVC
Burn et al. [15]
0
0/10
5.9
1/17
Abnl connection
Burn et al. [15]
4.5
1/22
5.9
1/17
TGA
Burn et al. [15]
0
0/14
0
0/6
Drenthen et al. [2]
0.6
1/176
ccTGA (l-TGA)
Therrien et al. [17]
3.7
1/27
Drenthen et al. [2]
3.6
1/28
AVSD
Burn et al. [15]
7.7
1/13
7.9
3/38
Nora [16]
1
14
Drenthen et al. [2]
8
7/88
AS
Nora [16]
5
18
Driscoll et al. [18]
1.2
3/251
1.4
1/72
Drenthen et al. [2]
4.1
5/121
PS
Nora [16]
2
6
Driscoll et al. [18]
1.7
3/176
3.9
8/205
Drenthen et al. [2]
2.8
3/164
ASD II
Nora [16]
1.5
6
Drenthen et al. [2]
2.1
6/291
VSD
Nora [16]
2.5
9.5
Driscoll et al. [18]
2.7
2/74
Drenthen et al. [2]
3
10/334
2.9
11/384
CoA
Nora [16]
2.5
4
Drenthen et al. [2]
4
10/251
Ebstein
Drenthen et al. [2]
4
5/126
In general, it is stated that mothers with a CHD have a greater risk of CHD in the offspring than fathers (Table 4.1). The ratio for affected offspring born to affected mothers versus those born to affected fathers is about 2:5. In a national cohort study based on a Swedish population registry, it was even stated that men with both complex and simple CHD did not have an increased risk of having children with a CHD [20]. However, this was not supported by other studies [16, 18]. The excess of malformations in the offspring of affected women might be explained by the recognition that some of the key genes in cardiac development are imprinted. A maternal copy of the gene is necessary for normal heart formation, and therefore a deleterious allele of maternal origin would have a greater effect than those of paternal origin.
4.2.1.1 Diagnostics in Non-syndromic CHD
Traditional genetic testing involved Sanger sequencing of a number of genes in a stepwise manner to try to identify the causal mutation. Currently, many genetic laboratories have introduced next-generation sequencing (NGS) into service, which changed the whole field of genetics. With NGS, or massive parallel sequencing, millions of small DNA fragments can be sequenced at the same time, creating a massive pool of data. Bioinformatic analyses are used to piece together these fragments by mapping the individual reads to the human reference genome providing accurate data on DNA variation. NGS can be used to sequence all 22,000 coding genes (whole exome) or targeted to small numbers of individual genes (panels).
The contribution of the known CHD genes in adults with non-syndromic CHD without a family history of CHD is small. In some countries, target NGS of a panel of genes involved in CHD or whole exome sequencing (WES) is available. However, its usefulness is a matter of debate since many variants of unknown significance (VUS) will be found, and, in the absence of other affected family members, it is challenging to assign causality with certainty to the found variants. The use of a targeted NGS panel for genes involved in CHD might, at this point, be most useful when a monogenetic cause is suspected, for instance, in the case of parental consanguinity or familial CHD [21, 22]. Parental consanguinity is associated with a two- to threefold increased offspring risk of CHD. This is most likely due to the shared genetic variants among parents fitting autosomal recessive inheritance [23, 24].
Traditional G-banding karyotyping has been widely used to look for large (3–5 Mb) deletions or duplications of the genome (copy number variations, CNV) in CHD patients. This technique is gradually being replaced by techniques with higher-resolution, chromosomal microarray testing. Several chromosomal microarray platforms can be used for genome-wide CNV detection, including comparative genomic hybridization (CGH) arrays and single-nucleotide polymorphism (SNP) arrays. The resolution of these platforms has increased tremendously in the past year to up to 5–10 Kb. With these new techniques, smaller deletions and duplications can be found that may be pathogenic, also in isolated CHD cases.
Although the yield of chromosomal microarray testing is higher in patients with CHD and extracardiac anomalies, detection of CNVs by microarray testing can be considered in all adults with non-syndromic CHD. The diagnostic yield for non-syndromic CHD is around 4 % [25, 26]. Some chromosomal microarray platforms can also reveal regions of homozygosity, which might lead to the causal recessive gene underlying the heart defect.
In order to estimate the recurrence risk in offspring of a CHD patient, it might be useful to perform cardiac screening in family members. This is particularly true for left ventricular outflow tract obstructions (LVOTOs), ranging from bicuspid aortic valve to hypoplastic left heart syndrome. LVOTOs have been recognized as a group of CHDs with “high heritability” [27, 28]. Therefore, it is advised to perform cardiac screening of first-degree relatives (parents, siblings, and children) of patients with LVOTO in order to better estimate the recurrence risk in offspring [29].
4.2.2 Non-syndromic CHD with a Significant Family History of CHD
In familial non-syndromic CHD, the inheritance pattern can be autosomal dominant, autosomal recessive, or X-linked recessive. A person affected by an autosomal dominant condition has a 50 % chance of passing the mutated gene to each child. The chance that a child will not inherit the mutated gene is also 50 %. In families with autosomal dominant inheritance of CHD, reduced penetrance and variable expression of CHD are observed. Reduced (or incomplete) penetrance refers to the proportion of persons who are carrier of a mutation in a specific gene that do not exhibit signs and symptoms of the genetic disorder. Variable expressivity refers to the range of signs and symptoms that can occur in different patients with the same genetic condition. In autosomal recessive inheritance, a mutation in both copies of a gene must be present in order for the disease to develop. Someone who has a mutation in one copy of the gene (but no symptoms) is called a carrier. X-linked recessive diseases usually occur in males and are caused by a mutation in a single-recessive gene on the X chromosome. Mothers can be carriers of the disease and may be mildly affected.
In the following paragraphs, we will summarize a subset of genes involved in non-syndromic familial CHD, categorized in different subgroups of CHD. This list is incomplete since new CHD genes are identified in a rapid pace. A nice recent review of all non-syndromic and syndromic genes involved in CHD is given by Lalani et al. [11].
Septal Defects
The first single-gene mutation causing non-syndromic CHD was described in the transcription factor gene NKX2.5 in families with inherited atrial septal defect (ASD) and atrioventricular block [12]. Subsequently, mutations in GATA4 were found in two kindreds with apparent non-syndromic septal defects [13]. Mutations in the T-box gene TBX20 have been implicated in cardiomyopathy and septal defects [30]. Recently, deleterious variants in MESP1 were identified in patients with ventricular septal defects (VSD) or tetralogy of Fallot (TOF) [31]. Missense mutations in CRELD1 are found in nearly 6 % of isolated atrioventricular septal defects (AVSD) and AVSD associated with heterotaxy syndrome [32]. Septal defects are also caused by mutations in several genes encoding for sarcomeric proteins like ACTC1, MYH6, MYH7, and MYBPC3 [33].
Left Ventricular Outflow Tract Obstruction (LVOTO)
In familial left ventricular outflow tract obstruction (LVOTO), including aortic valve stenosis (AS), bicuspid aortic valve (BAV), coarctation of the aorta (CoA), and hypoplastic left heart syndrome (HLHS), Garg et al. initially described the first pathogenic mutations in the NOTCH1 gene [34]. In the past years, a limited number of NOTCH1 mutations were published in several small cohorts of LVOTO patients. Recently, a large cohort of 428 LVOTO patients was screened for NOTCH1. Pathogenic mutations in NOTCH1 occur in 1 % of sporadic LVOTO and in 7 % of familial LVOTO patients [35]. Reduced penetrance occurs in many non-syndromic CHD families, as is exemplified by families with NOTCH1 gene mutations; cardiovascular malformations are found in 75 % of NOTCH1 mutation carriers. Also marked variable expressivity is seen in the NOTCH1 families described by Kerstjens et al. as the spectrum of disease does not only involve left-sided heart defects but also right-sided heart defects affecting the pulmonary valve, conotruncal disease including pulmonary atresia, Fallot’s tetralogy and truncus arteriosus, thoracic aortic aneurysm, and other CHDs, such as anomalous pulmonary venous return, ASD, and VSD [35].
Other genes involved in non-syndromic LVOTO are GJA1, NKX2.5, GATA5, SMAD6, MYH6, GATA4, GATA6, and MEF2C, but the number of patients with mutations in these genes is low indicating that many genes need to be discovered [36].
Conotruncal Defects
Homozygous deleterious mutations in the NKX2.6 gene have been associated with persistent truncus arteriosus (PTA) and might be accompanied by an absent thymus [37]. Also, mutations in GATA6 have been reported in PTA and TOF and can be associated with pancreatic agenesis [38]. A minority of patients with TOF have a mutation in the FOG2 gene [39]. Also, rare mutations in FOXH1, TDGF1, GDF1, and THRAP2 cause isolated conotruncal defects.
Laterality Defects
Laterality disorders refer to a broad group of disorders caused by the embryonic disruption of normal left-right patterning, including situs inversus totalis and heterotaxy (or situs ambiguus). Situs inversus totalis is the mirror image reversal of all visceral organs including the lungs, spleen, liver, and stomach, whereas heterotaxy is the abnormal orientation of one or more organs along the LR axis. The CHDs common in laterality disorders include atrioventricular septal defects (AVSD), transposition of great arteries (TGA), double outlet right ventricle (DORV), abnormal systemic and/or pulmonary venous connection, abnormal position of the heart (dextrocardia), and isomerism of the atrial appendages. A marked proportion of patients with laterality disorders are born to consanguineous parents. Therefore, it is suggested that laterality disorders are more often than other CHDs characterized by autosomal recessive inheritance [40]. Also, X-linked recessive inheritance is more prevalent in patients with laterality disorders [41]. Recently, recessive mutations in MMP21 were identified in 5.9 % of non-syndromic heterotaxy cases [40]. Other recessive genes involved in heterotaxy are CCDC11, WDR16, and GDF1 [42–44].
X-linked recessive inheritance of heterotaxy was first described by Gebbia et al. who detected ZIC3 mutations in familial and sporadic heterotaxy patients [41]. ZIC3 mutations typically result in a variable phenotype ranging from situs abnormalities and heart defects to more syndromic heart defects involving additional midline, gastrointestinal, urogenital, and/or central nervous system anomalies. ZIC3 mutations have also been found in both males (5.2 %) and females (1.8 %) with isolated non-syndromic heterotaxy spectrum CHD such as TGA [45].
4.2.2.1 Diagnostics in Non-syndromic CHD with a Significant Family History of CHD
In familial non-syndromic CHD, it was shown that massive parallel sequencing of a panel of known cardiac genes increased the diagnostic value. In a cohort of families with non-syndromic CHD, targeted NGS of 57 genes known to be involved in CHD identified likely pathogenic mutations in 31 % of the families [21]. Jia et al. found potential causative variants in less than half of the families (46 %) by using the same technique [22]. However, even in familial non-syndromic CHD, it remains challenging to interpret the large number of identified variants. A chromosomal microarray is also advised in familial non-syndromic CHD patients since small microdeletions or duplications encompassing CHD genes can be the underlying cause [48].
4.2.3 Syndromic
CHD can occur in many genetic syndromes and have been found to be associated with mutations in a variety of single genes associated with syndromic disease. These monogenic syndromes with CHD are present in about 5 % of newborn babies with CHD. For the carer who advises adults with CHD in the preconceptional counseling setting, it is important to be aware of monogenic syndromic conditions that might have very subtle characteristics, as these conditions often have a high recurrence risk in the offspring of the patient. Some recognizable syndromes with CHD as a major feature of the condition are discussed below. In Table 4.2, different types of CHD and associated chromosomal causes and monogenic syndromes are listed.
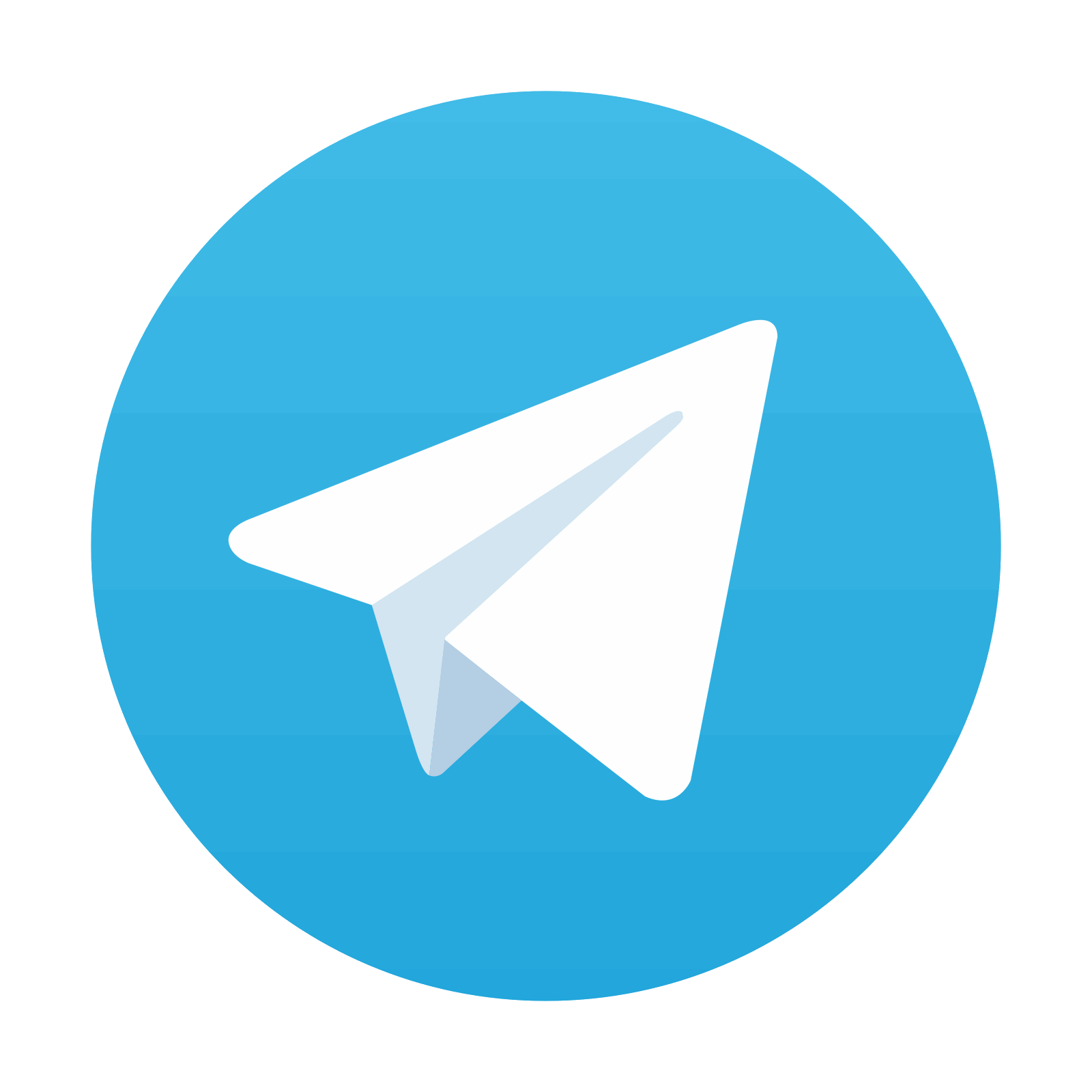
Table 4.2
Syndromes to consider in different types of CHD
TOF | 22q11 microdeletion syndrome, Alagille syndrome, CHARGE syndrome |
Interrupted aortic arch | 22q11 microdeletion syndrome |
Truncus arteriosus | 22q11 microdeletion syndrome |
LVOTO | Jacobsen syndrome, Turner syndrome, Kabuki syndrome, Potocki-Lupski syndrome |
PS | Noonan syndrome, Mowat-Wilson syndrome |
PPS | Alagille syndrome, Williams syndrome |
SVAS | Williams syndrome |
AVSD | Down syndrome, CHARGE syndrome |
VSD | Holt-Oram syndrome |
ASD | Holt-Oram syndrome, Noonan syndrome, Wolf-Hirschhorn syndrome |
PDA | Char syndrome, Mowat-Wilson syndrome |
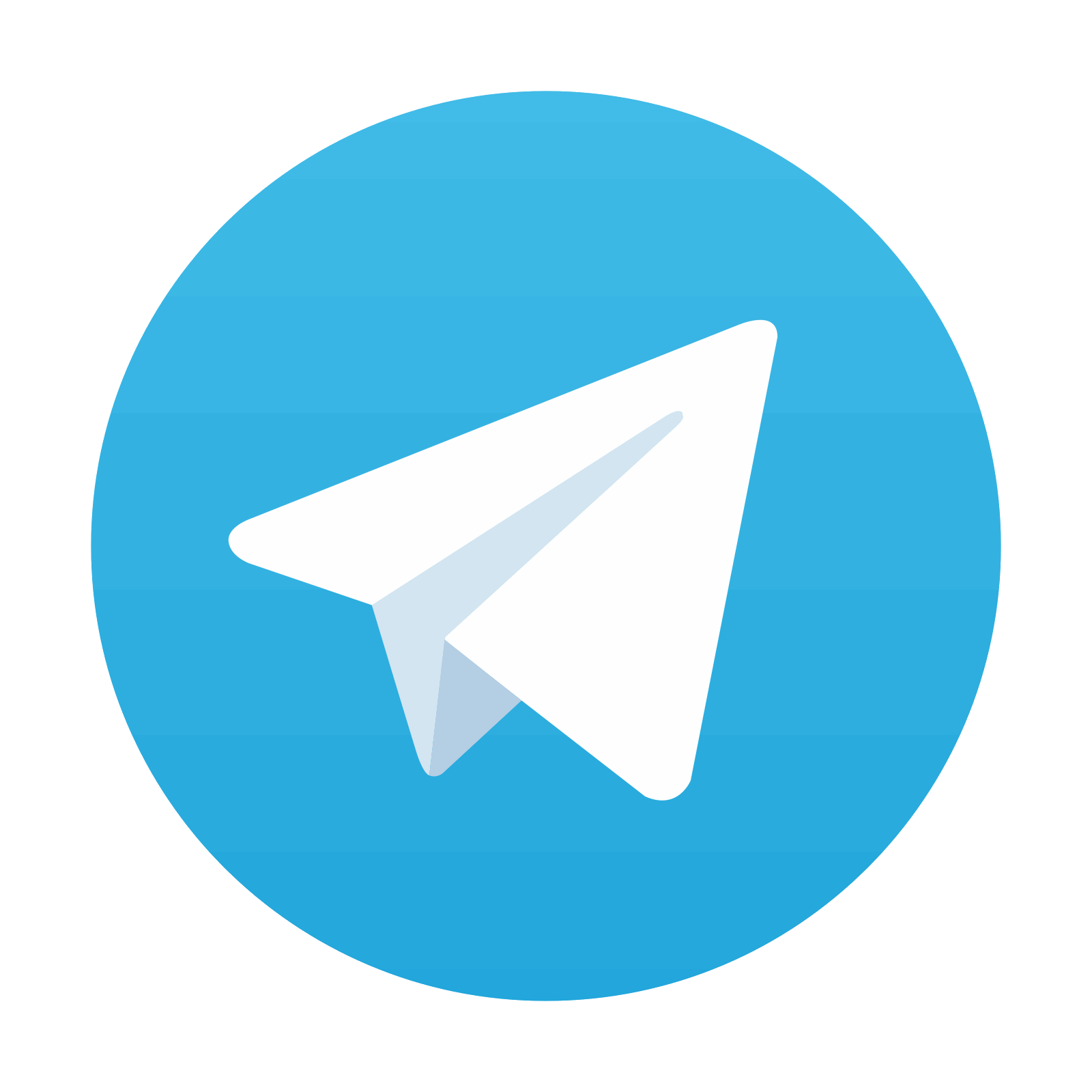
Stay updated, free articles. Join our Telegram channel

Full access? Get Clinical Tree
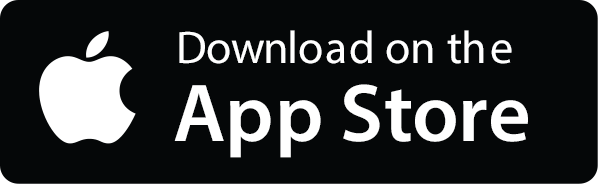
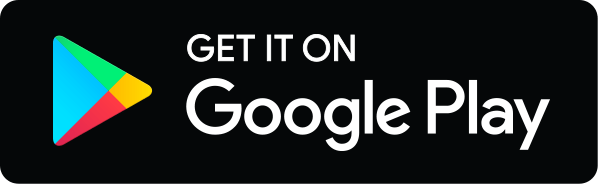