Background
Contrast ultrasound–mediated gene delivery (CUMGD) is a promising approach for enhancing gene therapy that relies on microbubble (MB) cavitation to augment complementary deoxyribonucleic acid (cDNA) transfection. The aims of this study were to determine optimal conditions for charge-coupling cDNA to MBs and to evaluate the advantages of surface loading for gene transfection in muscle and liver.
Methods
Charge coupling of fluorescently labeled cDNA to either neutral MBs (MB N ) or cationic MBs (MB + ) in low- to high-ionic conditions (0.3%-1.8% NaCl) was assessed by flow cytometry. MB aggregation from cDNA coupling was determined by electrozone sensing. Tissue transfection of luciferase in murine hindlimb skeletal muscle and liver was made by CUMGD with MB N or MB + combined with subsaturated, saturated, or supersaturated cDNA concentrations (2.5, 50, and 200 μg/10 8 MBs).
Results
Charge-coupling of cDNA was detected for MB + but not MB N . Coupling occurred over almost the entire range of ionic conditions, with a peak at 1.2% NaCl, although electrostatic interference occurred at >1.5% NaCl. DNA-mediated aggregation of MB + was observed at ≤0.6% NaCl but did not reduce the ability to produce inertial cavitation. Transfection with CUMGD in muscle and liver was low for both MBs at subsaturation concentrations. In muscle, higher cDNA concentrations produced a 10-fold higher degree of transfection with MB + , which was approximately fivefold higher ( P < .05) than that for MB N . There was no effect of DNA supersaturation. The same pattern was seen for liver except that supersaturation further increased transfection with MB N equal to that of MB + .
Conclusions
Efficient charge-coupling of cDNA to MB + but not MB N occurs over a relatively wide range of ionic conditions without aggregation. Transfection with CUMGD is much more efficient with charge-coupling of cDNA to MBs and is not affected by supersaturation except in the liver, which is specialized for macromolecular and cDNA uptake.
Gene-based therapies offer promise for the treatment of cardiovascular disease and lipid disorders. Enthusiasm for this approach has been tempered by the numerous obstacles to its success. One of the major barriers to gene therapy is the ability to effectively and safely transfer genetic material into the target tissue. Ultrasound-mediated cavitation of encapsulated microbubbles (MBs) and other acoustically active particles has increasingly been used in preclinical research to augment cardiovascular delivery of plasmid complementary deoxyribonucleic acid (cDNA) and therapeutic noncoding small ribonucleic acids. This method has been shown to be most effective when the therapeutic genetic material is loaded onto the acoustically active particles. Charge-conjugation of cDNA, which is a polyanion to the surface of cationic lipid MBs, represents a simple and effective approach for contrast ultrasound–mediated gene delivery (CUMGD).
The purpose of this study was to address important knowledge gaps with regard to the optimization of CUMGD. First, we characterized optimal ionic aqueous environment for charge coupling of plasmid cDNA to cationic MBs. We then compared tissue transfection of a reporter gene with CUMGD using either neutral MBs with ambient (noncoupled) cDNA or charge-coupled cDNA on cationic MBs, with and without excess ambient cDNA. This aim was designed to determine whether the presence of excess ambient cDNA either negates the advantage of surface conjugation or is wasteful and contributes little to transfection efficiency. We also compared the relative benefit of charge-coupling cDNA to the MB for the peripheral vasculature and the liver. These studies were performed because liver sinusoidal endothelial cells, which include Kupffer cells, represent a specialized endothelial cell type characterized by fenestrae, enhanced clathrin-dependent colloid uptake, and enhanced cDNA and polyanionic uptake capability, all of which have the potential to partially negate the influence of charge conjugation and CUMGD.
Methods
MBs
Near-neutral charge MBs (MB N ) were prepared by sonication of decafluorobutane saturated aqueous suspension of distearoylphosphatidylcholine (2 mg/mL) and polyoxyethylene-40-stearate (1 mg/mL). Cationic MBs (MB + ) were prepared by the addition of distearoyl-trimethylammonium propane (0.4 mg/mL) to the suspension. The mean diameter and concentration of MBs were determined by using Coulter counter (Multisizer III; Beckman Coulter, Fullerton, CA). Electric surface potential (zeta potential) for MB + and MB N was determined by measurement of their electrophoretic mobility (ZetaPALS; Brookhaven Instruments, Holtsville, NY) in 1 mmol/L KCl at pH 7.4.
Plasmid-MB Coupling
Coupling efficiency of cDNA to the MB surface under variable ionic solution environments was performed by flow cytometry. Plasmid cDNA (pGL4.13; Promega, Madison, WI) was combined with either MB N or MB + at a previously described supersaturation ratio of 20 μg of cDNA per 10 8 MBs in NaCl solution at concentrations of 0.3%, 0.6%, 0.9%, 1.2%, 1.5%, and 1.8%. MBs were washed by flotation centrifugation, and cDNA was labeled with SYBR-Gold (Invitrogen, Carlsbad, CA). Histograms of fluorescent intensity for control and cDNA-incubated MBs were assessed in duplicate by flow cytometry (FACSCanto II; BD Biosciences) at 488-nm excitation, and data were expressed as intensity geometric mean. To ensure that cDNA was at saturating concentrations, flow cytometry was also performed after combining MB + in 1.2% NaCl with cDNA at concentrations of 0, 1, 2, 4, 10, 20, and 40 μg/10 8 MBs.
MB Aggregation
MB aggregation, which can occur by surface coupling of cDNA, was quantified by particle size on electrozone counting of MB + alone or after conjugation with plasmid cDNA (20 μg/10 8 MBs) in the various concentrations of NaCl solutions described previously. Data were quantified in duplicate by the percentage of particle events >5 μm in diameter.
Effects of cDNA Conjugation on MB Acoustic Response
Frequency-amplitude histograms were obtained from MB + (1 × 10 7 mL −1 ) preincubated with plasmid cDNA at 0.3%, 0.9%, 1.2%, or 1.8% NaCl. Ultrasound (1 MHz) exposure was performed in a flow phantom with a 50-cycle pulse produced by a single-element transducer (Olympus NDT; Sonic Concepts Inc, Bothell, WA) at a peak negative acoustic pressure of 1 MPa. Cavitation signals were received by a broadband (10 KHz to 20 MHz) hydrophone interfaced with a receiver (RAM-5000; Ritec Enterprises Inc, Rochester, NY) and an oscilloscope (44MXi-A; Teledyne LeCroy, Chestnut Ridge, NY) and were digitized at 100-MHz sampling frequency. Data analysis was performed using MaATLAB (The MathWorks, Natick, MA), and cavitation was characterized by the broadband signals between the harmonic peaks.
Animals
The study was approved by the Animal Care and Use Committee at Oregon Health & Science University. Transfection of a firefly luciferase reporter gene was studied in studied 42 wild-type C57Bl/6 mice aged 8 to 12 weeks. Mice were anesthetized with inhaled isoflurane (1.0%-1.5%), and a catheter was placed in a jugular vein for administration of MBs. Animals were recovered for in vivo optical imaging of transfection 3 days later.
In Vivo Transfection Protocols
For CUMGD, animals received a single intravenous injection of 2.5, 50, or 200 μg pGL4.13 luciferase plasmid premixed for 5 min with 2 × 10 8 either MB + or MB N suspended in approximately 100 μL 0.9% NaCl ( n = 7 for each of the six conditions). These concentrations were selected to produce subsaturation, saturation, and supersaturation conditions for charge-coupling. MBs were infused intravenously over 1 min. Two separate phased-array ultrasound probes coupled to two separate ultrasound systems (Sonos 7500; Philips Medical Systems, Andover, MA) were fixed in place and used to simultaneously expose both the liver and proximal hindlimb adductor muscles using aqueous ultrasound coupling gel as a standoff. Ultrasound exposure was performed during MB injection and for an additional 9 min using harmonic power Doppler mode at 1.6 MHz, a pulsing interval of 5 sec, a pulse repetition frequency of 9.3 kHz, and a system setting mechanical index of 1.3. The acoustic focus was placed at the level of the tissue of interest. According to needle hydrophone measurements, these settings produce a beam elevational profile of about 6 mm when defined by half-maximal (0.8 MPa) full-width.
Detection of Gene Transfection
In vivo assessment of transfection was determined by optical imaging of luciferase activity 3 days after CUMGD. d -Luciferin (0.15 mg/g) was administered by intraperitoneal injection. Animals were anesthetized with inhaled isoflurane and placed in an optical imaging system (IVIS Spectrum; Caliper Life Sciences, Waltham, MA). Bioluminescent activity was measured 10 min after luciferin injection with an open emission filter and medium binning. Light activity from regions of interest placed over each proximal hindlimb was quantified by total photon flux (p/s).
Statistical Analysis
Differences between conditions for gene loading and aggregation or for transfection efficiency on optical imaging according to pGL4.13 concentration were assessed using a Kruskal-Wallis test. A Mann-Whitney U test was performed for any post hoc differences between two categories and for comparing MB type for transfection efficiency by optical imaging. Differences were considered significant at P < .05.
Results
MB Characteristics and Gene Loading
The mean diameter was similar for MB + and MB N (2.95 ± 0.13 vs 2.68 ± 0.04 μm, P = .07). Zeta potential measured in 1 mmol/L KCl was significantly positive and substantially greater for MB + than for MB N (+38.6 ± 5.1 vs −2.5 ± 1.3 mV, P < .0001). Under conditions in which there were known saturating concentrations of plasmid cDNA, flow cytometry detected little fluorescent cDNA coupled to the surface of MB N , regardless of the ionic conditions ( Figure 1 ). Charge-coupling of plasmid cDNA to MB + was two to three orders of magnitude greater than to MB N under all ionic conditions. The greatest degree of charge-coupling of cDNA to MB + tended to be at 1.2% NaCl, and substantial interference with gene conjugation was seen at the highest NaCl concentration (1.8%). Dose-response cDNA coupling to MB + in 1.2% NaCl demonstrated that these experiments were performed at the saturating concentration point ( Figure 2 ). Because of the potential for DNA-mediated MB aggregation under low ionic conditions, electrozone counting was used to assess the proportion of particles that were >5 μm in diameter. Before cDNA incubation, the percentage of particles that were >5 μm was similar for MB + and MB N (7.0 ± 0.8% vs 6.9 ± 0.8%, P = .82). Only MB + demonstrated a significant ( P = .03, Kruskal-Wallis test) increase in events with low-ionic conditions, indicating aggregation at NaCl of 0.6% and lower ( Figure 3 ).



MB Acoustic Response
Frequency-amplitude histograms during ultrasound exposure (1 MHz, 1 MPa) were used to examine how cDNA loading or aggregation influenced MB acoustic response ( Figure 4 ). Experimental conditions were tested only with MB + , because there was very little cDNA loading on MB N . Incubation at the various concentrations of NaCl that produce different degrees of cDNA coupling to MB + resulted in no significant change in acoustic response compared with MBs without gene. The amplitude and frequency of the harmonic peaks and the “between-peak” broadband signal were identical, indicating similar nonlinear response to both stable and inertial cavitation irrespective of gene loading. Light microscopy illustrated minimal resistance of MB + to acoustic destruction when they were in aggregate form at low ionic strength ( Supplementary Videos ; available at www.onlinejase.com ).
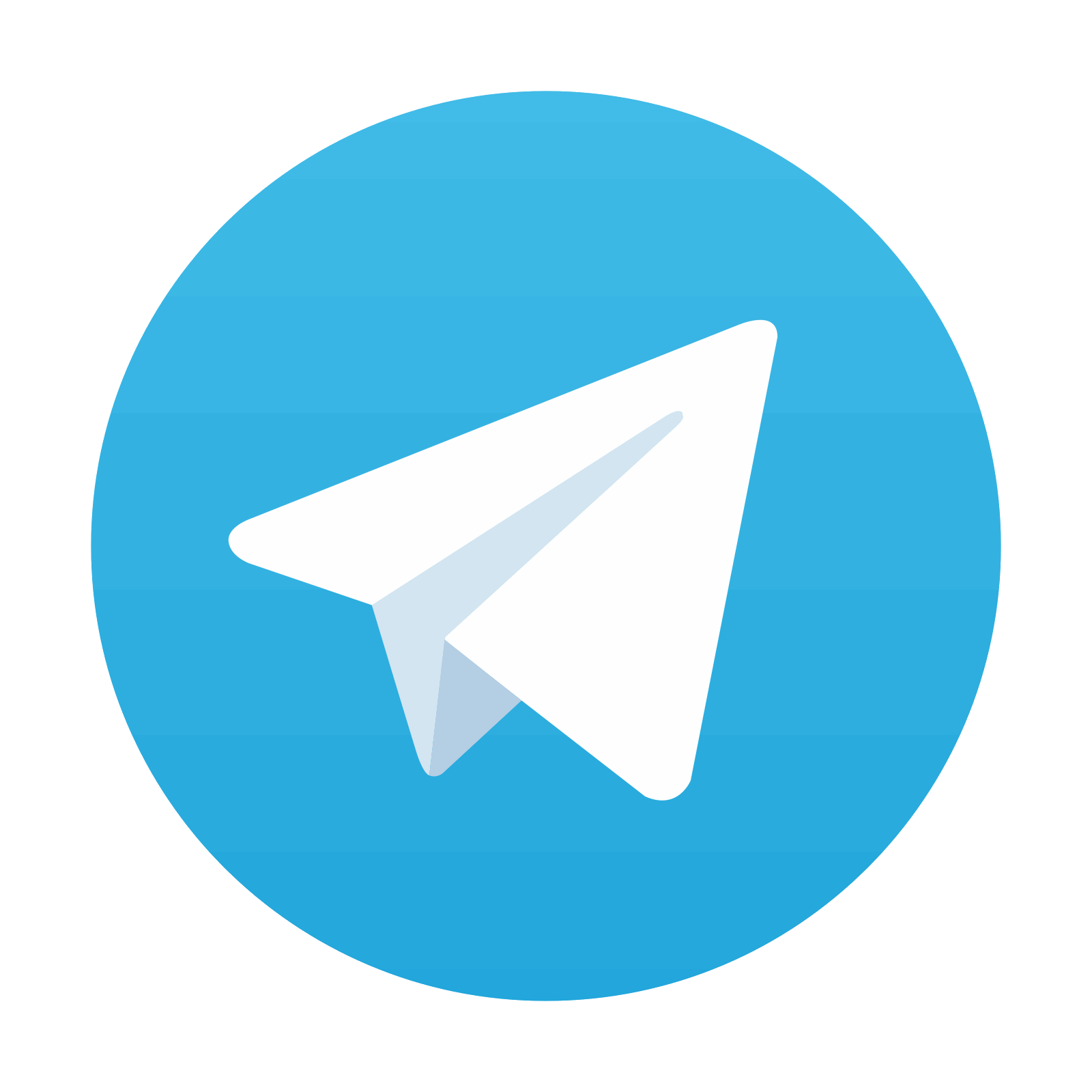
Stay updated, free articles. Join our Telegram channel

Full access? Get Clinical Tree
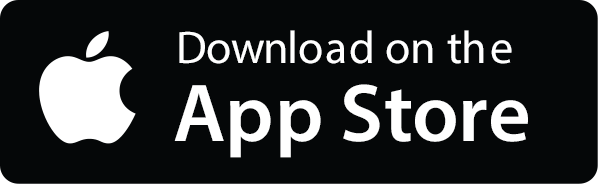
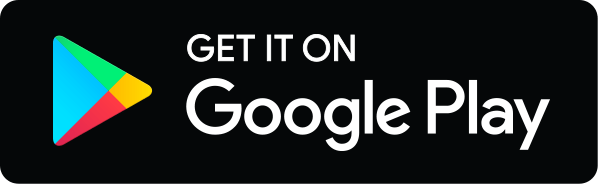
