In recent years, significant progress has been made in refining our understanding of the evolution and anatomy of the venous system. The exploration and identification of genes linked to growth factors that affect normal and abnormal development of blood vessels has spurred our insight into this field. Furthermore, rapid advances in ultrasound techniques and imaging protocols coupled with major strides in endovenous intervention have revolutionized the diagnosis and treatment of a variety of acute and chronic venous disorders. The old paradigms advocating conservative “observational” management of venous disorders appear to be rapidly falling away as the therapeutic pendulum in venous disease now swings from palliation toward cure.
This chapter discusses the embryology, histology, anatomy, and major clinical disorders affecting the inferior vena cava (IVC) and provides an overview of the clinical features, diagnosis, and treatment of caval disease.
In the seventh week of fetal gestation, the posterior cardinal veins, which drain the caudal portion of the fetus, begin to involute and form the subcardinal and supracardinal venous system.1 The infrarenal segment of the IVC arises from the caudal right supracardinal vein. The renal segment of the IVC arises from a venous network located around the aorta in a collar-like configuration, called the subsupracardinal anastomosis (the so-called renal collar). The anterior segment of the renal collar gives rise to the left renal vein. The suprarenal segment of the IVC arises from the right subcardinal vein, with the exception of the hepatic venous segment, which arises directly from the hepatic sinusoids.2
The walls of all veins are composed of three layers: intima, media, and adventitia. A combination of endothelial cells and a connective tissue layer make up the intima. Venous valves that are bicuspid in configuration are formed by the endothelium. The next layer is the internal elastic lamina (IEL), which are thick elastic fibers. The media, rich in collagen and smooth muscle cells, lies between the IEL and adventitia. The media of both the superior vena cava (SVC) and IVC are primarily composed of connective tissue. The adventitia consists of loose connective tissue with nerve fibers and is difficult to differentiate from the adjacent media, particularly in the respective vena cava. This outer layer protects and anchors the vessel to the surrounding tissues.3,4
The IVC begins at the junction of the right and left common iliac veins at the level of L4–L5 and ascends to the right of the aorta in the retroperitoneum. The IVC lies behind the head of the pancreas, the superior portion of the duodenum, and the portal vein. It traverses the diaphragm, coursing in a groove on the posterior hepatic surface, and then passes through the pericardium and enters the right atrium. The Eustachian valve is located on the lateral aspect of the IVC at the junction of this vessel with the right atrium. The IVC is otherwise a valveless vessel. Tributaries of the IVC include the paired lumbar and renal veins and the hepatic veins. In addition, the right gonadal, suprarenal, and inferior phrenic veins also drain into the IVC from the right side of the body. The left gonadal and suprarenal veins drain into the left renal vein, and the left inferior phrenic vein drains into the left suprarenal vein.1 Kaufman et al5 reported caval diameters not exceeding 28 mm in a magnetic resonance angiography–based study of 150 patients. () The widest point of the cava, in their study, at the confluence of the iliac veins had an average maximal diameter of 31 +6 mm. In addition, the cross-sectional profile of the IVC was usually ovoid rather than circular in shape. The incidence of megacava has been estimated to be 2% to 3% in some studies.6,7
The importance of collateral pathways cannot be overemphasized in any discussion of the IVC. Important collateral pathways include communication between the thoracic and abdominal wall veins, the lumbar azygos anastomosis, and the vertebral pathways; these potential communications assume importance in the case of IVC obstruction. The renal veins enter the IVC at the L1–L2 level, and the hepatic veins usually enter the IVC just below the diaphragm. In rare cases, the right lobe of the liver is drained by an inferior right hepatic vein that joins the IVC below the other three main (right, middle, left) hepatic veins.
Duplication of the IVC occurs in 2% to 3% of cases and results from a persistence of the inferior segment of the left supracardinal vein. IVC transposition (left IVC) also results from the same vestigial developmental anomaly, along with involution of the inferior segment of the right supracardinal vein. This anomaly occurs in 0.2% to 0.5% of cases and is usually found at necropsy. The left IVC usually joins the suprarenal IVC at or just inferior to the left renal vein but may occasionally join the azygous or hemiazygous system.
IVC interruption with azygous or hemiazygous continuation occurs in approximately 0.6% of necropsy specimens and results from a failure of the right subcardinal vein to join the intrahepatic venous complex. Blood then flows from the suprarenal IVC into the “continuation” and then into the heart. In these cases, the hepatic veins drain directly into the right atrium. Of the several pathways potentially available to return blood to the heart in case of IVC stenosis or occlusion, the most common is the ascending lumbar venous plexus. These vessels communicate with an extensive vertebral venous network and eventually drain into the azygous or hemiazygous systems and then the SVC. Periureteric and gonadal venous communications can also potentially bypass the IVC. Other potential networks include the superficial abdominal wall veins, the systemic to portal venous pathway, and pathways via the superficial epigastric and circumflex iliac veins.8,9
Given the diversity of pathology that may involve the IVC, a full complement of imaging modalities may be used, either independently or in many cases as a combination of complementary techniques. Ultimately, it is incumbent upon the clinician to appropriately tailor the diagnostic strategy based on the availability of institutional expertise and resources.
Currently available radiographic options to evaluate the IVC include duplex ultrasonography, computed tomographic (CT) imaging, magnetic resonance imaging (MRI), and contrast venography or phlebography. This section provides a general overview of each venous imaging modality and its appropriate application in specific clinical disorders related to the IVC. As in the treatment of any disease entity, the operational caveat when dealing with disorders of the IVC centers around an individualized assessment of the particular abnormality in question and the development of a tailored treatment strategy sensitive to the nuances of each individual patient.
The normal IVC is situated anterior to the spine and lies to the right of the aorta. The upper abdominal portion is easily visualized where the liver serves as an acoustic window.10 The inferior portion, however, may prove to be more challenging to image, depending on the body habitus of the patient and the degree of overlying bowel gas. The IVC size varies with respiration and throughout the normal cardiac cycle. During inspiration, the IVC maximally dilates because of decreased venous return, with the opposite effect observed during expiration. During the Valsalva maneuver, flow in the IVC may be temporarily suspended. Furthermore, the dimensions of the IVC depend on the size of the patient and magnitude of right atrial pressure. Although Doppler flow signals are phasic in the distal vena cava, similar to the pattern seen in extremity veins, they may be pulsatile in proximity to the heart because of reflected right atrial pulsations.11 Because the IVC transmits both respiratory and cardiac pulsations as the transducer moves closer to the heart, the pattern of the Doppler tracing may adopt a characteristic “sawtooth” configuration. There are no valves in the IVC (except for the Eustachian valve at the IVC–right atrial junction) and usually no valves in the common and external iliac veins.
Indications to evaluate the IVC with ultrasonography are mainly to determine whether the vessel is patent and to discern the cause of any obstructive process. Ultrasound evaluation of the IVC should be carried out in both longitudinal and transverse planes and is best accomplished with a 3.5- or 5-MHz curved array transducer. The entire IVC should be examined, including the suprahepatic, intrahepatic, and infrahepatic portions. The mean diameter of the cava in normal individuals is 17.2 mm when measured below the renal veins during quiescent respiration. The diameter increases proportionately approximately 10% during deep inspiration.12
The development of spiral CT has greatly enhanced our understanding of venous disorders. Intravenous (IV) contrast administered through an arm vein is usually necessary in this study for optimal evaluation of vascular structures. A large territory of body zones may be imaged over a short period of time with a single breath-hold. IV iodinated contrast material opacifies the lumens of all patent venous structures. It outlines their pathways; identifies thrombotic occlusions, collaterals, and anomalous routes; and has the ability to distinguish between adjacent lymph nodes and venous structures.
Because of the disparity in physical properties of contrast dye compared with blood, filling defects may be produced at major venous junctions because of the wash-in of unopacified blood that does mix homogeneously with opacified blood. These potential filling defects may be large and falsely construed as representative of a thrombus or mass. This phenomenon may be evident within the IVC at the levels of the renal and hepatic veins.
The major advantage afforded MRI is the ability to demonstrate flow and flow dynamics without the need for nephrotoxic contrast agents. There are few contraindications to MRI scanning, including patients with cerebral aneurysm clips, metallic foreign bodies, pacemakers, and implantable cardiac defibrillators. The contraindication to contrast may represent the most common scenario when MRI demonstrates its advantages over CT or contrast venography. Magnetic resonance venography may also have an advantage over CT or venography in detecting thrombus burden in small branching veins, such as in pelvic deep venous thrombosis (DVT). MRI is also an excellent modality for identifying the venous drainage of venous malformations and other soft tissues. Disadvantages include its cost, which exceeds that of venous CT and ultrasound imaging.13 Although a noninvasive examination, compliance may prove challenging for many patients such as obese, elderly, and very ill patients, because of the requirement to breath-hold and lay motionless for several minutes.
Contrast venography or phlebography has historically been considered the “gold standard” against which all other modalities are measured. However the availability of duplex ultrasonography and other cross-sectional imaging techniques, such as CT and MRI has dramatically diminished the role of venography in the diagnosis of venous disorders.14,15 Venacavography is usually reserved for rare cases that are deemed to be cross-sectional imaging diagnostic failures, for patients being considered for IVC filter placement, or as the initial diagnostic component for endovascular intervention. Appropriate indications for the use of contrast venography include situations in which iliofemoral or caval venous thrombosis is encountered and catheter-directed thrombolysis or thrombectomy is contemplated. Venography in these cases can provide important information about the proximal extent of the thrombus and can remove the imaging barriers caused by overlying bowel gas and body habitus that often obscure the vena cava on ultrasound examination.16
Complications. Although contrast venography is a relatively safe procedure in capable hands, it does introduce additional expense and discomfort and pose risks related to vascular access and obligatory dye use that need to be taken into account in considering its first use in the evaluation of patients with venous disorders. These include reactions to iodinated contrast and the potential risk of postprocedural vein thrombosis (~1%–2%).16,17 These complications may be reduced by aggressive flushing of the veins with saline, diluting the contrast when appropriate, and concurrent administration of heparin during selective catheterization. A rare complication is extravasation of dye, which may result in skin necrosis, and may be avoided by meticulous monitoring of the vascular access site with fluoroscopy.
The age-and gender-adjusted incidence of venous thromboembolism (VTE) in the United States has been estimated to be 77.6 per 100,000, corresponding to more than 350,000 new cases per year.18 One-third of patients will sustain a recurrent VTE within 10 years. At least 100,000 to 200,000 deaths each year in the U.S. may be directly or indirectly related to this disease. The clinician faces the challenge not only of accurate but expeditious diagnosis of the disorder in concert with providing patients with the most appropriate, cost-effective treatment options currently available. Anticoagulation remains the cornerstone of treatment for DVT and pulmonary embolism (PE).
Etiology. A patient presenting with iliocaval obstruction, whether acute or chronic, serves as one of the more challenging scenarios encountered in the spectrum of venous disorders. The available endovenous reconstruction options are relatively unproven and are unfortunately wedded to a paucity of data in the vascular literature. Options for device therapy are often culled from anecdotal experience or by extrapolation of data generated from device application in other vascular beds. These limitations are frequently encountered in patients with chronic obstruction, and they immediately conspire to raise obstacles to achieving technically and clinically successful outcomes in the treatment of patients with this disorder.
Among the most symptomatic patients are those who have incurred the combination of iliac vein and IVC obstruction. The usual pattern in these patients is the association of bilateral iliac vein thrombosis with accompanied infrarenal IVC occlusion. Isolated thrombotic occlusion of the IVC is relatively uncommon, and its causes are usually varied. The vast majority of cases are secondary to clot extension from iliac vein thrombosis, transvenous extension of abdominal or pelvic tumor thrombus, and IVC filter thrombosis. Other causes accounting for obstruction of the IVC include central venous or tunneled catheters, trauma, prothrombotic states, tumors, aneurysms, radiation therapy, extrinsic compression, and membranous webs.
Clinical Presentation. Patients with IVC obstruction often report that leg elevation does little to improve the swelling in their affected limbs. The clinical picture manifests itself in a wide spectrum of signs and symptoms all commensurate with venous hypertension. Depending on the time course of evolution of the thrombosis and the development of collateral vessels, as many as 10% of individuals may remain symptom free.19 Chronic IVC obstructive lesions tend to manifest with indolent and subtle symptoms. In other instances, previously asymptomatic patients may present acutely with the onset of distal thrombosis. Affected patients usually develop significant collaterals of the abdominal and retroperitoneum to compensate for occlusion of the IVC. If collaterals are adequate, the patient remains clinically asymptomatic unless he or she develops a subsequent thrombotic event, upon which time the issue becomes clinically apparent. When acute caval thrombosis occurs, most often patients present with bilateral lower extremity edema, recent and unexplained weight gain, and back pain. As time goes by, they may complain of a fullness in the groin, severe leg pain, and skin changes consistent with chronic venous stasis. In the more chronic phases of the process, the diagnosis may be more elusive and the physical findings subtle. Prominent collateral veins may be seen on the inferior anterior abdominal wall or in the vicinity of the symphysis pubis. When both renal veins are involved, nephrotic syndrome may occur. A rare but potentially serious complication is the development of phlegmasia cerulea dolens, which may occur when acute IVC thrombosis is associated with iliofemoral DVT. The resultant massive leg edema can lead to venous gangrene secondary to arterial ischemia from extrinsic compression, which, if undiagnosed or treatment is delayed, often leads to major amputation, massive pulmonary embolus, or death. The small subgroup of unfortunate individuals affected with this devastating complication usually have underlying metastatic cancer or the etiology of their thrombosis may be associated with heparin-induced thrombocytopenia. Phlegmasia cerulea dolens represents one of the few bona fide medical emergencies in the management of patients with iliocaval thrombosis.
Diagnostic Evaluation. The evaluation of patients referred for symptomatic acute or chronic vena cava obstruction is relatively similar. Duplex ultrasonography is the initial imaging tool used to assess for the extent of venous thrombosis, distribution, and the acquisition of segmental flow velocities and waveforms. Accurate knowledge of the extent of the disease process is mandatory before any consideration of potential therapeutic procedures. The patency of the infrainguinal venous system, the status of the common femoral and iliac veins (common and external), and the proximal extension of the thrombus within the vena cava are important determinants of the final therapeutic outcome in these cases. Common iliac and distal caval obstructions are suggested by loss of phasicity and low velocities at the femoral level. If large collaterals or a prominent hypogastric iliac system is present, the flow velocity may be preserved. However, even under these circumstances, the femoral waveform will demonstrate little or no phasicity.
The ultrasound findings in acute IVC thrombosis are distension, absence of flow, and the presence of thrombotic material within the vein lumen.20 The echogenicity of thrombus varies with its age. Recently formed thrombus appears anechoic. As thrombus ages, the echogenicity increases slightly, but the intensity of the echoes remains low. The pitfalls commonly encountered in sonographic diagnosis include acute thrombus that may be so hypoechoic that it may be missed in the absence of a color-flow examination and false-positive diagnosis of thrombosis, which may occur in very low-flow states in which Doppler flow detection is difficult.
The adjunctive role of CT reconstitutions and MRI also merits mention. These modalities can provide important information about coexistent pelvic vein and iliac vein compression syndromes. Acute thrombus within the vena cava presents as a filling defect within the lumen, often expanding the lumen in the acute phase, which appears round and large in cross-section. Old thrombus that does not dissolve or recanalize may convert to a fibrotic scar and obliterate the residual lumen to a thin cord. In rare instances, old thrombus may calcify and appear as a mass within the occluded lumen. MRI may also demonstrate congenital anomalies, thrombosis, extrinsic compression, and tumor involvement noninvasively and without contrast. Collateral formation in caval obstruction is often better appreciated with MRI than with venography or ultrasonography.21,22
Contrast venography of the vena cava or venacavography is reserved for rare cases in which the diagnosis cannot be confirmed by cross-sectional imaging. The most important role of venography is as an adjunct imaging modality used in concert with interventional endovenous procedures, including IVC filter placement. Transfemoral venography may show definitive iliocaval obstruction and delineate collateral pathways. Thrombus produces a discrete and characteristic angiographic filling defect. In the context of long-standing or chronic occlusions, the IVC may not opacify at all, and only collateral vessels are seen. The level and extent of occlusion influence the pattern of collateral development. If there is a suspected isolated stenosis or membranous web, increased accuracy may be achieved with multiple oblique projections despite a relatively “benign” anteroposterior (AP) view. The presence of collaterals in a symptomatic patient should be considered an indication of obstruction. In some cases, venography from the internal jugular vein approach may be warranted to determine the upper extent of the occlusion if it is ill defined on other imaging studies.
Therapeutic Strategies. The treatment goals for DVT are to mitigate the severity and duration of symptoms; prevent PE; minimize the risk of recurrence; prevent the development of postthrombotic syndrome (PTS) related to residual obstruction and valvular incompetence, which frequently results in disability; and obviate the sequelae of chronic thromboembolic pulmonary hypertension. The complications associated with proximal (iliofemoral, vena caval) DVT occur at a frequency commensurate with the time frame in which they present. As an example, PE develops in 50% of untreated patients within the first few days after the occurrence of proximal DVT. Up to 75% of pulmonary emboli are asymptomatic; furthermore, as many as 25% to 52% of patients with documented DVT without symptoms of PE have high-probability lung scans or positive pulmonary CT angiogram results.
Prospective natural history studies have shown that patients with DVT develop progressive valvular damage with incompetence over time (months to years), with a resultant 66% incidence of PTS manifested by pain, edema, skin hyperpigmentation, or ulceration.23 The underlying pathophysiology of PTS is ambulatory venous hypertension caused by residual venous obstruction and valvular incompetence, with this pathophysiologic combination shown to be particularly potent in the genesis of this disorder. Patients with more extensive DVT involving the proximal segments, including the iliac veins and IVC, are more likely to develop PTS. Recent studies indicate that pharmacologic lysis and surgical or mechanical thrombectomy may improve clinical outcomes by preserving valve function and restoring the patency of the venous vasculature, thus in turn mitigating the clinical complications of the disease.24,25
The origins of endovenous reconstruction of iliocaval obstruction dates back to the mid-1980s, when venous stents were successfully used to treat and palliate obstruction associated with malignancies and Budd-Chiari syndrome.26 This relatively noninvasive approach offered a safe and viable alternative to surgical bypass or prosthetic replacement of the IVC, which were in vogue during that time. The pioneer work performed by César Gianturco in 1969 represented the seminal experience for stenting of the venous system.27 Through the collaboration of Gianturco and industry sponsors, the stainless steel Gianturco Z stent (Cook, Bloomington, IN), was successfully deployed to treat Budd-Chiari–related hepatic and IVC obstruction, although it was fraught with a high incidence of restenosis and stent migration.28
Obstructive symptoms in the extremities are variable as to the type and magnitude, often with only unilateral manifestations. In the largest series of patients (n = 120), reported by Raju and colleagues, the female-to-male ratio was 2:1, as was the limb involvement, left-to-right ratio. Clinical features were confined to the unilateral limb in 67% of patients, with one-third of patients manifesting symptoms in both limbs. Other series have observed that up to 5% to 10% of patients may remain asymptomatic even in face of IVC occlusion. Eighty-two percent of the IVC lesions were infrarenal, 14% were suprarenal although confined to below the diaphragm, and 4% extended cephalad into the thoracic IVC. In 93% of limbs, the thrombus extended into the common iliac vein, distal into the external iliac vein in 37%, and 31% into the common femoral vein. Total occlusions represented 14% of these lesions, with 86% noted as partial (>60% stenosis).29,30
Procedural success for stenotic lesions was excellent and reported to be 100%, with a 66% success rate for occlusions. The safety of the stent procedure as reinforced with no periprocedural (30-day) or late stent-related mortality without PE as a complication. There was, however, postoperative mild to moderate back pain that was commonly observed in approximately 20% of patients, which was readily controlled with nonsteroidal antiinflammatory drugs.30
Cumulative primary and primary-assisted patency rates were 58% and 82%, respectively, at 2 years. At 3.5 years, 74% of patients were pain free, and 51% of limbs demonstrated resolution of edema. The overall clinical outcomes were 70% of limbs with an excellent (+3) or improved (+2) clinical outcome at the median follow-up of 11 months (range, 3–59 months).29
Despite standard anticoagulant therapy, approximately 29% to 79% of patients with DVT develop some symptomatic manifestations of PTS. Severe complications and ulceration occur in only 7% to 23% and 4% to 6% of patients, respectively. The rapid and complete thrombus dissolution commonly achieved with thrombolytic therapy potentially could reduce the incidence of this dreaded complication. The benefits of early thrombus removal supported by experimental studies show that thrombolysis of acute DVT preserves valve and endothelial function. Furthermore, when early (<90 days) spontaneous lysis is restored, patency of veins and valvular function was preserved most of the time. A recent systematic review of thrombolytic therapy showed increased rates of early vein patency, but major hemorrhagic rates were increased compared with standard unfractionated heparin treatment.31 Because of methodologic flaws in small, single-center trials, it is not possible to draw definitive conclusions about the effects of thrombolytic therapy on the incidence of PTS. The National Venous Thrombolysis Registry, a prospective, multicenter study, enrolled 473 patients in 63 sites in an effort to assess the efficacy of catheter-directed pharmacologic thrombolysis (CTD) in the treatment of patients with DVT.32 The results reported complete lysis in 31% and partial lysis in 52%; 17% of patients achieved less than 50% lysis. The primary patency rate at 6 months was 65% and at 1 year was 60% with the degree of lysis found to be a significant predictor of early and continued venous patency. Of the patients who experienced complete clot resolution, 78% had patent veins at 1 year compared with 37% of patients with less than 50% clot resolution after lytic therapy. In addition, early success of thrombolytic therapy directly correlated with 6-month valvular function. Sixty-two percent of the patients with less than 50% of their clot burden lysed exhibited valvular incompetence compared with 72% of the patients who had complete lysis and resultant normal valve function (P < 0.02). An important observation was made in the subgroup of patients with acute, first-time iliofemoral DVT who had initial successful thrombolysis: 96% of the veins remained patent at 1 year. Major bleeding was observed in 11%; 39% of these occurred at the access site, and 13% were retroperitoneal bleeds. Minor bleeding was reported in 16% of patients. Major neurologic complications occurred in 0.4% of patients. PE occurred during treatment in 1% of patients, and two deaths were reported for a mortality rate of 0.4% in the entire series. These studies suggest that CDT for DVT achieves more rapid lysis, may reduce pain and edema, preserves valvular competence, and completely restores vessel patency compared with standard anticoagulation or systemic thrombolytic therapy, although at the expense of a higher rate of hemorrhagic complications, compared with anticoagulation alone.
At present, CDT should be reserved for exceptional circumstances, such as patients with limb-threatening ischemia caused by phlegmasia cerulea dolens and in young patients with extensive iliofemoral or IVC DVT (i.e., those with a favorable risk-benefit ratio). Other candidates who potentially might benefit from CDT are those with multisegment DVT, those with expected long-term survival, and individuals who remain symptomatic despite therapeutic anticoagulation. Additional randomized trials are warranted to specifically address these issues.
In an era when all clinicians seek adherence to evidence-based guidelines, with few exceptions, patients with DVT or PE are treated similarly. Specifically, regarding the role of adjunctive therapy with pharmacologic thrombolysis, the recently published American College of Chest Physicians (ACCP) guidelines are as follows:33
The routine use of systemic lytic therapy is not recommended in patients with DVT or PE (grade 1A).
The routine use of CDT is not recommended in patients with DVT (grade 1C).
In selected patients with extensive acute proximal DVT (e.g., iliofemoral or IVC, symptoms <14 days, good functional status, life expectancy >1 year) who have a low risk of bleeding, CDT may be used to reduce symptoms and patient morbidity if appropriate expertise and resources are available (grade 2B).
After successful CDT in patients with acute DVT, correction of underlying venous lesions using balloon angioplasty and stents is recommended (grade 2C).
Pharmacomechanical thrombolysis (e.g., inclusion of thrombus fragmentation, aspiration, or both) in preference to CDT is done to shorten treatment time if appropriate expertise and resources are available (grade 2C).
After successful CDT in patients with acute DVT, the same intensity and duration of anticoagulation treatment as for comparable patients who do not undergo CDT is recommended (grade 1C).
Contraindications to thrombolytic therapy are stratified into two categories, absolute and relative. These are shown in Table 24-1.
Absolute Contraindications | Relative Contraindications |
---|---|
Stroke (<2 months) Bleeding diathesis Active or recent GI bleeding (<10 days) Neurosurgery (intracranial, spinal; <12 months) Intracranial trauma (<3 months) or neoplasm | CPR (<10 days) Major nonvascular surgery or trauma (<10 days) Uncontrolled hypertension (systolic >180 mm Hg or diastolic >110 mm Hg) Puncture of noncompressible vessel Intracranial tumor Recent eye surgery Pregnancy Bacterial endocarditis |
Various thrombolytic agents, including streptokinase, urokinase, alteplase, reteplase, and tenecteplase are currently available in the United States, however none are not currently FDA approved for venous disorders. Each agent has its own unique characteristics with respect to fibrin affinity, specificity and selectivity, half-life, and average time to clot lysis. Despite the availability and increasing use of these agents, no dosage regimens have been universally validated to guide therapy; the most frequently reported regimens are shown in Table 24-2.
Agent | Infusion |
---|---|
Alteplase (tPA) | 1–3 mg/hr |
Reteplase (rPA) | 0.5–1.0 U/hr |
Tenecteplase (TNK) | 0.25–0.5 mg/hr |
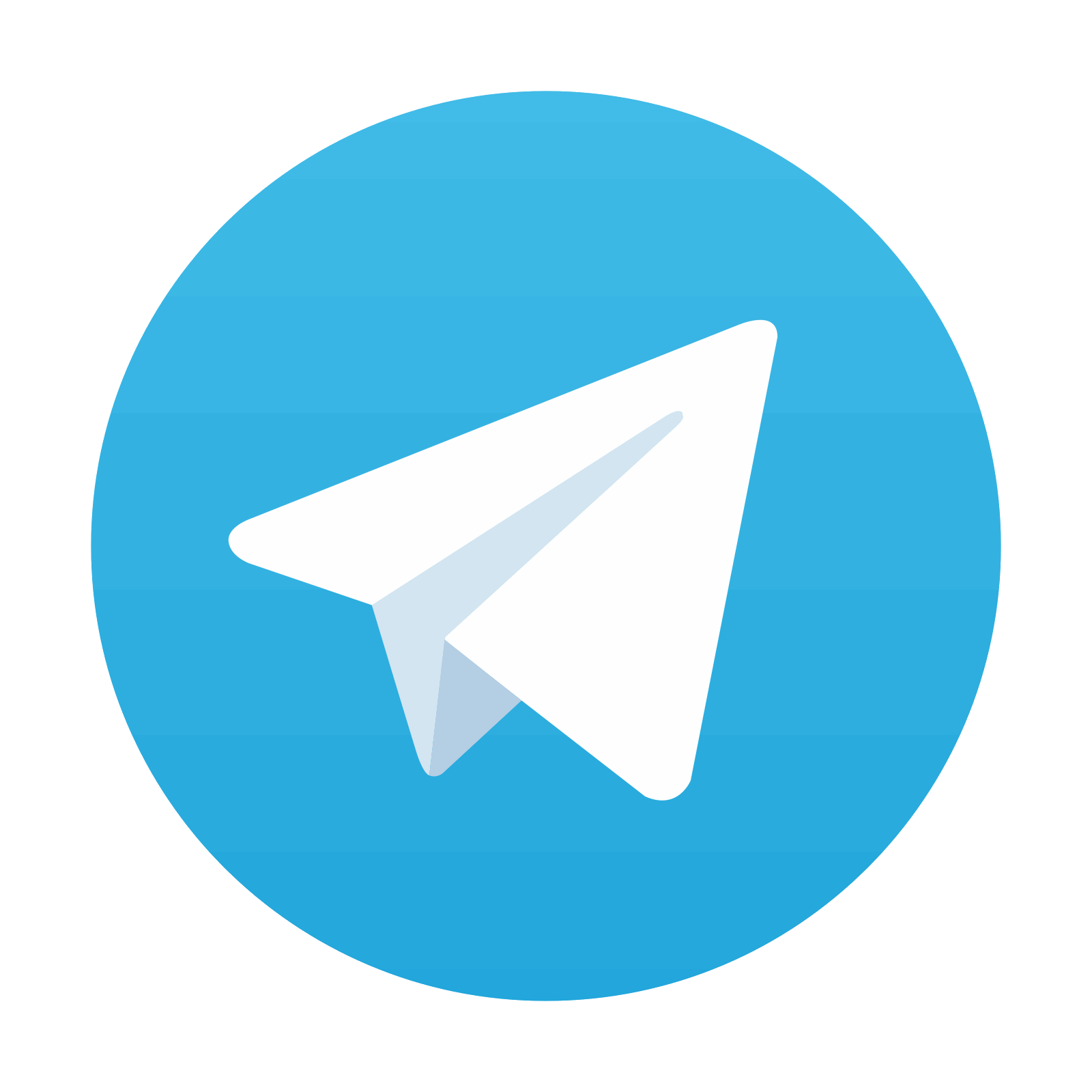
Stay updated, free articles. Join our Telegram channel

Full access? Get Clinical Tree
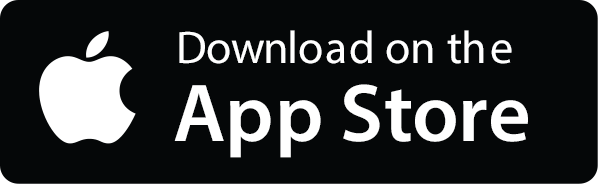
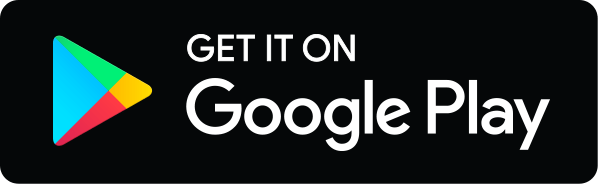
