Infection
Therapy duration
Guidelines
Pneumonia (Community-acquired)
Minimum 5 days, afebrile 48–72 h, stable
IDSA Guidelines [16]
Pneumonia (Hospital-acquired, ventilator-associated, and healthcare-associated)
8 days (if responding); unless Pseudomonas aeruginosa (14–21 days)
ATS/ IDSA Guidelines [17]
Implantable cardiac device infections
(AHA Guidelines) [18]
Pocket infection
10–14 days after device removal
Bloodstream infection
At least 14 days after device removal
Complicated infection
At least 4–6 weeks
AHA Guidelines [18]
Bacteremia (Catheter-related, without other implantable devices)
2–6 weeks depending on organism and clinical circumstances
(IDSA Guidelines) [19]
Infective endocarditis
4–6 weeks (depending on type, organism—although 2-week regimens have been described for certain organisms, transplant candidates should receive longer durations)
(AHA/IDSA Guidelines) [20]
Urinary tract infection
Uncomplicated cystitis in women
3–7 days
(IDSA/ESMID Guidelines) [21]
Uncomplicated pyelonephritis in women
7–14 days (depending on antibiotic used)
(IDSA/ESMID Guidelines) [21]
Catheter-associated UTI
7 days if prompt response; 10–14 days if delayed response
IDSA Guidelines [22]
Cellulitis (non-necrotizing)
7–10 days or more (varies by organism and clinical course)
IDSA Guidelines [23]
For patients who have an infection with a VAD in place, recent guidelines are helpful with duration of therapy and decisions about long-term suppression [2, 4]. Organisms are most frequently staphylococci, but Gram-negative bacilli such as Pseudomonas; other Gram-positive organisms such as enterococci; and fungal infections may occur [14, 15]. Fungemia was described in 7 VAD patients in one series (5 with candidemia, 2 with aspergillosis) for an attack rate of 0.1 infections/1000 days of device support [24]. Most patients with a VAD-related infection can safely undergo transplantation provided that their bloodstream infection is controlled at the time of transplantation, and appropriate antibiotics are administered post-transplant as well as pre-transplant to eradicate any additional remaining foci. In some cases, a bacteremia or candidemia cannot be controlled prior to transplant because of continued presence of the device. In such situations, transplantation may be undertaken, but with the known risk of persistence or recurrence of the infection and with lengthy antimicrobial therapy afterwards. It is a principle of endovascular infections in general that definitive treatment often involves removal of the device (catheter, pacemaker, ICD), but with VAD’s the situation is complicated by the difficulty of removal and replacement of the device, which can only be undertaken in extreme circumstances. Therefore, control of the infection and (in some cases) continuous suppression of previous organisms until transplant may be all that can be achieved prior to transplantation. Unfortunately, as with other healthcare-associated infections, the rise of multiply-resistant bacterial pathogens has complicated therapy and sometimes led to use of newer drugs such as daptomycin [25].
As part of pre-transplant evaluation, the recipient undergoes a serologic screening panel which can help with risk stratification and with prophylaxis post-transplant (Table 23.2) [13]. This generally includes, at a minimum, serologic testing for HIV, HBV, HCV, syphilis, CMV, EBV, and VZV. Patients with a positive HCV antibody screening test should undergo HCV RNA screening and, if positive for HCV RNA, should undergo evaluation by a hepatologist. Patients with positive anti-HBc and anti-HBs but negative HBsAg are those who have had HBV in the past and have resolved it; no further therapy is necessary. Patients with positive HBsAg have active HBV infection and should be seen by a hepatologist. Patients with isolated positive anti-HBs (with negative HBsAg and negative anti-HBc) have received effective vaccination. Patients with positive anti-HBc, negative HBsAg and negative anti-HBs are either those who have resolved infection (with anti-HBs waning below the level of detectability), or those with early active infection in the “window period” (in which case the anti-HBc IgM is positive), or those with false-positive anti-HBc. Further evaluation with HBV DNA and anti-HBc IgM is desirable.
Patients with a positive non-treponemal serologic test for syphilis (e.g. RPR) should have a treponemal test performed for confirmation (e.g. FTA-ABS or MHATP). If the treponemal test is negative, the RPR is most likely a biologic false-positive and is not a contraindication to transplantation. If the treponemal test is positive, the patient should be evaluated for active syphilis and treated according to standard guidelines. Involvement of an infectious disease specialist is highly recommended. Treated syphilis is not a contraindication to transplantation. After treatment the RPR titer may take months to years to resolve, so a negative RPR is not required after treatment of syphilis before proceeding to transplantation.
Serology for VZV is performed primarily to detect the uncommon seronegative transplant candidate, and to help with management of VZV exposures post-transplant. Less than 10 % of adults are seronegative for VZV in most regions. However, VZV-seronegative individuals are at risk for severe primary varicella if exposed post-transplant, and should receive varicella vaccination pre-transplant if they are not on immunosuppression already, and if they are not expected to undergo transplantation within 4 weeks. (If they do receive varicella vaccination pre-transplant and then get a donor offer less than 4 weeks later, they may undergo transplantation but with immediate post-transplant initiation of acyclovir or ganciclovir therapy.) VZV-seronegative candidates who cannot receive varicella immunization pre-transplant (due to being on immunosuppression or being too close in time to transplant) should be counseled extensively on their risk of acquiring varicella post-transplant and should be encouraged to report any exposures immediately so that prophylactic antiviral therapy can be administered.
Serology for CMV and EBV should be performed primarily to determine risk status in conjunction with the CMV and EBV serologies of the donor (see below). The relevant serologies are CMV IgG and EBV VCA IgG. In both cases, the highest-risk status is donor-seropositive, recipient-seronegative (D+/R−) which often warrants special monitoring and in some cases for CMV, may warrant extended prophylaxis [1, 6].
HIV was once considered a contraindication to transplantation, but in recent years, successful transplantation of HIV-positive kidney and liver recipients has been reported through a multicenter study and has become widely accepted practice [26]. Thoracic transplantation of HIV-positive candidates has been less commonly performed, but has been reported [27, 28] and is likely to increase in the future, based on the experience in abdominal transplantation, and based on the large number of individuals with HIV who have controlled viral loads and who are surviving longer with chronic diseases including heart disease. Transplantation of HIV-positive recipients requires careful monitoring of pharmacokinetics by a pharmacist with knowledge of the extensive drug interactions particularly between protease inhibitors and calcineurin inhibitors [29]. Evaluation of HIV-positive candidates should include an assessment of the patient’s HIV-RNA viral load and CD4 count over time as well as any opportunistic infections in the past, and any indications of active infection that might persist or recur post-transplant [29].
The transplant candidate should undergo testing for latent TB infection with an interferon-gamma release assay (IGRA) blood test, or PPD skin testing, and should be considered for latent TB therapy with isoniazid if either of these tests is positive (see below) [13, 30].
Further recommendations on management of latent TB infection [30] as well as pre-transplant non-tuberculous mycobacterial infections [31] are given below.
The pre-transplant evaluation is also an important time to update immunizations, which are more effective when administered prior to transplant [13, 32]. The American Society for Transplantation (AST) has published guidelines for immunizations in pretransplant candidates and post-transplant recipients [32]. Because of the degree of detail of these recommendations, the reader is referred to the AST Guidelines for further information [32]. For pediatric candidates, standard immunization series should be completed prior to transplant whenever possible [32]. For adult candidates, immunizations should be administered according to the recommendations for adult immunizations with the exception that live virus vaccines (e.g. varicella vaccine, zoster vaccine) should only be administered if the patient is not on immunosuppression and transplantation is not anticipated within 4 weeks [32]. The patient should receive yearly influenza vaccine with the injected preparation rather than the live attenuated nasal vaccine [33]. The family members of the transplant candidate should also receive influenza immunization to create a “circle of protection” around the patient [33]. Pneumococcal vaccine should be administered if it has not been received within 5 years and if the patient has not already had 2 lifetime doses. The 3-dose hepatitis B vaccine series should be administered to any candidate who is seronegative for anti-HBs. Although commonly administered at 0, 1, and 6 months, an accelerated course (e.g. 0, 1, and 2 months) can be given to those in whom transplantation is anticipated to occur soon. (Although HBV vaccine can be given post-transplant, it is less effective in patients on immunosuppression.) Tetanus-diphtheria-acellular pertussis (Tdap) vaccine should be administered if not already received (although the standard time interval for repeating tetanus vaccine is 10 years, the Tdap vaccine can be given if the last tetanus vaccine was >2 years previous.) The advantage to Tdap is the additional protection against pertussis, which can cause protracted infection in immunocompromised patients.
HPV vaccine should be offered to patients aged 11–26 of both genders. HPV is a significant issue in long-term survivors of transplantation although the efficacy of pre-transplant HPV vaccine in preventing post-transplant HPV is yet unknown. Zoster vaccine should be offered to candidates age 60 and above who are not on immunosuppression and who are not anticipated to undergo transplantation within 4 weeks. It can also be offered to those aged 50–59 who meet the above criteria (it is FDA-approved but not ACIP-recommended for this age group.)
Early Post-transplant Infections
According to the paradigm initially developed by Rubin [34], there are 3 distinct time periods of post-transplant infection risk: the first month, months 2–6, and after 6 months. At any stage, the risk for infection is a combination of the “net state of immunosuppression” and the patient’s particular environmental exposures [34]. In the first post-transplant month, although immunosuppressive medications are being administered at high doses, the full effects of immunosuppression have not yet taken hold on the immune system, and the vast majority of infections are not opportunistic infections, but rather are those which can occur after any major surgical procedure [34]. These include catheter-related infections, urinary tract infections, pneumonias, empyemas, sternal wound infections, and mediastinitis. Risk factors for these infections include protracted intensive care unit stay, protracted requirement for mechanical ventilation, primary graft dysfunction, technical complications of surgery, need for reoperations (such as exploration and evacuation of hematomas), renal dysfunction and need for renal replacement therapy, multiorgan dysfunction, older age, and diabetes.
Neutropenia associated with transplant medications such as ganciclovir, valganciclovir, mycophenolate mofetil, and azathioprine can also contribute to infection risk. As discussed below, multidrug-resistant organisms are increasingly seen, and occur particularly in those patients with extensive prior antibiotic exposures both pre- and post-transplant [7–9].
While most of the above infections can occur in any solid organ transplant recipient, heart recipients are particularly at risk for intrathoracic infections, including mediastinitis. Sternal wound infections may occur as after any cardiac surgery, but may be more common in transplant recipients than in nontransplanted cardiac surgery patients. A study from a large Spanish database (the RESITRA database) reported an incidence of 4.8 % for incisional surgical site infections in heart recipients, with staphylococci being the most common pathogens, but a variety of other organisms were seen including Gram-negative bacilli (Proteus, and extended-spectrum-beta-lactamase producing E. coli); and yeast (Candida albicans and C. glabrata) [35]. Patients with pre-existing VAD-related infections are at risk for mediastinitis with their previous infecting organisms unless a lengthy course (4–6 weeks) of pathogen-directed post-transplant therapy is administered.
Other commonly seen infections during the first month include candidiasis (especially oropharyngeal thrush) and herpes simplex virus (HSV) reactivation. Prophylaxis of oropharyngeal candidiasis with either nystatin oral suspension (swish and swallow) or clotrimazole troches is almost universal. Oropharyngeal and occasionally esophageal HSV infection can result from reactivation of HSV-1, while genital and perianal HSV can result from reactivation of HSV-2. Thus most patients who are not receiving ganciclovir or valganciclovir prophylaxis for CMV should be receiving acyclovir or valacyclovir prophylaxis for HSV (and VZV). Uncommonly, opportunistic infections such as cytomegalovirus or aspergillosis may be seen during the first month, in the setting of pre-transplant immunosuppression or excessive environmental exposures.
Donor-Transmitted Infections
A wide variety of organisms (bacterial, fungal, viral) have been reported to be transmitted via solid organ transplantation [36]. Some, but not all, are preventable through pre-transplant screening [13]. Like prospective transplant recipients, prospective deceased donors undergo a rigorous screening process consisting of a serologic panel, review of medical records, and detailed medical and social history (in this case from the donor’s family members.) In addition, blood cultures, urine culture, and chest radiography are generally performed although the results of cultures may not be available until after the transplant has been performed. Details of standard deceased donor testing can be found in Table 23.2 and on the OPTN/HRSA website http://optn.transplant.hrsa.gov/PoliciesandBylaws2/policies/pdfs/policy_2.pdf [37].
OPTN/HRSA minimum requirements for deceased donor testing (United States) for infection |
---|
U.S. FDA-licensed Anti-HIV-1 and Anti-HIV-2 serologic screening test |
Hepatitis screen serological testing, including HBsAg, HBcAb, and anti-HCV |
VDRL or RPR |
Anti-CMV |
EBV serological testing |
Blood and urine cultures; urinalysis within 24 hours prior to cross-clamp |
Additional donor testing (under specific circumstances) |
NAT (Nucleic Acid Amplification Testing) for HCV, HBV, HIV (see text) |
Recipient pre-transplant testing |
Serology for HIV, HBV, HCV (anti-HCV and HCV RNA), syphilis (RPR or syphilis IgG), CMV (CMV IgG), EBV (VCA IgG) Toxoplasma IgG, as above |
Serology for VZV, hepatitis A, ± HSV |
Testing for latent TB infection (interferon-gamma release assay, or tuberculin skin test) |
For those with possible exposures: serologies for Strongyloides, Chagas disease, schistosomiasis |
Blood, urine, sputum, stool microbiologic diagnostic testing if clinically indicated |
Chest Xray; CT scans and other imaging if clinically indicated |
The serologic panel performed on deceased donors is similar to that for recipients, and serves three main functions . First, certain serologies may disqualify the donor completely – in the US, in the past, this has included a positive test for HIV or a positive HBsAg indicative of active HBV infection, although in other parts of the world these criteria might not disqualify all donors. Most recently, the possible use of HIV-positive donors is being explored through the HOPE Act [38]. Second, serology results might suggest limiting the donor to a particular subgroup of recipients (e.g. an HCV-positive donor to an HCV-positive recipient, see below.) Finally, serologic screening may help to determine risk stratification and prophylaxis protocols post-transplant, as with CMV and EBV for which the highest risk group is seronegative recipients with seropositive donors (D+/R−). A positive donor serology for syphilis is not a contraindication to transplantation, but the recipient should be treated. Donor blood testing for latent TB infection, in the form of the interferon-gamma release assay (IGRA), is not yet universally available due to the requirement that the test be performed in a specialized laboratory using living cells.
Until recently, most of the elements of the serology panel were antibody serologies (IgG) indicating exposure at some time in the past. This landscape is changing, after transmissions of viral infections such as HIV and HCV were reported during the “window period” (prior to antibody seroconversion) of the donor [39, 40]. The deceased-donor time frame was traditionally not long enough to conduct assays that directly detect the presence of viral genomes, which would detect infection earlier than antibody seroconversion and thus shorten the “window period”. More recently, with development of rapid molecular testing known as NAT (“nucleic acid amplification”) testing, it has become possible for the majority of organ procurement organizations (OPO’s) to perform NAT testing in the deceased donor time frame [41]. A national discussion ensued regarding whether or not NAT testing should be applied to all prospective donors, or whether it should be restricted to those with CDC-defined high risk behaviors (including injection drug use, sexual promiscuity, and incarceration) [40]. Recent guidelines from the US Public Health Service have recommended NAT testing for HCV for all donors, and NAT testing for HIV in high-risk donors [41]. For further discussion on the risk of HCV and HBV in the cardiac transplant recipient, see section on “Other Viruses” below.
Other infections which would generally disqualify prospective donors include bacteremias with virulent organisms such as MRSA, VRE, or multidrug-resistant Gram-negative bacteria; active invasive fungal infection, or active tuberculosis. Occasionally, a bacterially-contaminated organ, where the infection was not previously suspected, may result in transmission of infection to the recipient [42]. However, bacterial meningitis with community-acquired organisms such as Pneumococcus is not considered a contraindication to transplantation if appropriate antibiotics are administered to the recipient post-transplant [43, 44]. Recently a study from UCLA extended this concept by reporting safe transplantation of hearts from donors with bacterial sepsis [45], although it should be noted that this referred to sepsis with community–acquired organisms and not with nosocomial, multidrug-resistant organisms. Also, caution should be exercised in prospective donors with abnormal CSF findings without positive bacterial cultures, since such fatal transmissions as West Nile virus, rabies, lymphocytic choriomeningitis virus, or even lymphoma could result [36]. If a bacterial meningitis donor is to be utilized, proof of bacterial infection with a positive culture of donor CSF is important.
The formation of the Disease Transmission Advisory Committee (DTAC) by OPTN/UNOS was a significant advance towards a more evidence-based understanding of the nature, risks, and outcomes of donor-transmitted infections [46]. Since 2005, this group has reviewed all reported possible transmissions of infection or malignancy, and have scored these as proven, probable, or possible according to uniform criteria [46]. Transplant centers and clinicians are strongly encouraged to report any suspected donor-derived infections via this mechanism, which contributes to the knowledge base of the transplant community as a whole, in addition to facilitating notifications and communications to other transplant centers who have transplanted organs from the same donor.
Cytomegalovirus
Cytomegalovirus (CMV) remains one of the most important post-transplant infections, although prophylactic and pre-emptive therapy strategies have reduced its incidence and severity [1, 5, 6]. Prior to the prophylaxis era, between 40–80 % of transplant recipients developed symptomatic CMV disease. The highest risk group is the donor-seropositive, recipient-seronegative (CMV D+/R−) group in which the recipient has no antecedent CMV-specific immunity but acquires a CMV viral load from the donor. Those recipients who were already seropositive for CMV (R+) can develop CMV reactivation under the influence of immunosuppression, particularly after treatment for rejection, and those who are D+/R+ can either reactivate their own CMV strain from the past, or can develop superinfection with the donor’s strain of CMV. Classically, symptomatic CMV appeared most frequently between 1 and 4 months post-transplant, but with prophylaxis, a first episode of CMV might occur in the second half of the first post-transplant year or even later [1] (Fig. 23.1).


Fig. 23.1
Cytomegalovirus inclusion in type 2 pneumocyte from immunocompromised patient with CMV pneumonia. The cell contains an eosinophilic nuclear inclusion that obscures most of the cell nuclear and multiple smaller basophilic cytoplasmic inclusions. 1000× magnification (Image courtesy of Dr. Carol Farver, Pathology Department, Cleveland Clinic)
Clinical manifestations of CMV infection fall into three categories: asymptomatic viremia, “CMV syndrome,” and tissue-invasive CMV. All 3 categories are referred to as “CMV infection,” and the latter 2 categories (CMV syndrome and tissue-invasive CMV) are referred to as “symptomatic CMV” or “CMV disease.” Asymptomatic viremia is usually associated with a low blood viral load (often <10,000 copies/ml) and is usually discovered as part of a pre-emptive monitoring program. “CMV syndrome”, associated with moderate elevation of the viral load (e.g. 10,000–100,000 copies/ml), is a flulike illness with fevers, chills, malaise, myalgias, and often leukopenia, thrombocytopenia, and mild elevations of the liver function tests. Tissue-invasive CMV (usually associated with high viral loads, 100,000 copies/ml and above) is the most clinically severe manifestation, and refers to the situation where CMV can be detected in tissue by histopathology and/or tissue immunostaining. The organs most commonly involved are the lung (CMV pneumonitis), liver (CMV hepatitis), GI tract (CMV esophagitis, gastritis, enteritis, colitis) and less commonly the eye (CMV retinitis) and central nervous system (CMV meningoencephalitis.) For lung and liver transplant recipients, the allograft is the most common localization for tissue-invasive CMV, but in heart recipients, CMV myocarditis is uncommon, and GI tract manifestations appear more frequently. Tissue-invasive CMV is often associated with debilitation, multiorgan dysfunction, and a prolonged recovery phase. Higher peak viral loads in the initial CMV episode are associated with risk for recurrences, tissue-invasive disease, and development of ganciclovir resistance.
Detection of CMV was originally performed by tissue culture of peripheral blood, but this was time-consuming and labor-intensive. Shell-vial centrifugation culture reduced the turnaround time to 48 hours but was less sensitive at low viral loads. The pp65 antigenemia test provided a semi-quantitative measure of CMV load, but was labor-intensive and lost sensitivity if samples were mailed in from distant sites. Most centers now use some form of molecular diagnostic test, most commonly the quantitative CMV PCR, which expresses the viral load in copies/ml. However, inter-center comparisons have been complicated by inter-laboratory variation and a multiplicity of locally-designed assays. The recent development of a WHO standard (in IU/ml) and the advent of the first FDA-approved quantitative PCR test should help to standardize these disparate results [47].
Given the severity of CMV in the early years of transplantation, considerable effort has been devoted to developing systems of prevention. Two main strategies, termed “prophylaxis” and “pre-emptive therapy”, have been shown to reduce CMV incidence and severity.
“Prophylaxis” refers to administration of an antiviral agent to an entire group. In cardiac transplantation, this approach was pioneered by Merigan et al. in 1992, with a randomized trial of a 4-week intravenous ganciclovir regimen compared with placebo [48]. This regimen significantly reduced the incidence of symptomatic CMV from 46 % to 9 % in the recipient-seropositive (R+) subgroup, but did not significantly reduce symptomatic CMV in the high-risk D+/R− subgroup [48]. Today, most centers use prophylaxis with valganciclovir, which is an oral analogue of IV ganciclovir but which has greater bioavailability than the older formulation of oral ganciclovir. The PV16000 study compared 100 days of prophylaxis with valganciclovir to oral ganciclovir in kidney, heart, pancreas, and liver transplant recipients, and found that oral valganciclovir prophylaxis was associated with less breakthrough viremia while on prophylaxis and less ganciclovir resistance, but the incidence of viremia and symptomatic CMV disease by the end of 1 year were comparable between the two groups [49]. In D+/R− kidney [50] and in all lung recipients [51], extended courses of prophylaxis have recently been reported in randomized trials to be beneficial with regards to reduction in CMV events (6 months and 12 months respectively) but whether this is the case for D+/R− heart recipients remains to be demonstrated. The addition of CMV hyperimmune globulin (CMVIg) to prophylaxis regimens for D+/R− recipients, a once-popular strategy [52], is now less frequently used due to the high cost of this therapy and the perceived efficacy of valganciclovir. However, recent studies using large databases suggest that the use of CMVIg as well as use of antiviral prophylaxis may improve long-term outcomes in cardiac transplantation [53, 54].
The other main strategy for CMV prevention is “pre-emptive therapy,” which restricts anti-CMV antiviral usage only to those who develop evidence of CMV infection on a sensitive early detection test (usually either quantitative CMV PCR or pp65 antigenemia.) This requires surveillance monitoring of all patients at risk. Advocates of pre-emptive therapy cite reductions in cost, toxicity, and possibly antiviral resistance associated with use of less antiviral drug [55]. They also point out that “late CMV” can occur after discontinuation of prophylaxis, and can be highly symptomatic [56]. However, the overall efficacy of prophylaxis has been shown in many studies over time [57]. With pre-emptive therapy, logistics can be daunting, and missing even one sample can lead to development of high viral loads and overt CMV disease prior to detection. Prophylaxis may also provide other benefits, such as prevention of other herpesviruses such as Epstein-Barr virus [58] and human herpesvirus-6 (HHV-6). Most centers now use valganciclovir prophylaxis, at least for D+/R− recipients (for at least 3 months), but may choose either prophylaxis or pre-emptive therapy for lower-risk (R+) recipients. Some centers have chosen to use a combined strategy of prophylaxis and pre-emptive therapy in order to detect “late CMV” occurring after prophylaxis while still at a low level of viral load, although this strategy has yet to be subjected to a randomized trial. It should be noted that valganciclovir causes both neutropenia and thrombocytopenia, and the CBC with differential should be carefully monitored (at least every 1–2 weeks, preferably weekly) while on extended courses of valganciclovir. CMV D−/R− recipients do not require anti-CMV prophylaxis, but generally receive prophylaxis for herpes simplex virus (HSV) and varicella-zoster virus (VZV) in the form of acyclovir or valacyclovir, for at least the first 1–3 months post-transplant.
The effects of CMV on the function of the allograft, particularly on cardiac allograft vasculopathy, have been the subject of intense research interest [12, 59, 60]. Early studies suggested that development of symptomatic CMV, or being in the high-risk D+/R− subgroup, were associated with greater risk for allograft dysfunction and development of CAV [61]. However, not all studies have uniformly shown this [62, 63]. Valantine et al. examined late outcomes of the groups from the original 1992 heart transplant IV ganciclovir prophylaxis study [48], who had been randomized to ganciclovir or no ganciclovir prophylaxis [64]. She found that ganciclovir prophylaxis (especially in patients who did not receive calcium channel blockers) was associated with significantly less risk for CAV [64]. There is also a suggestion that longer-term, low-level CMV viremia may be more deleterious to the allograft than short-term, high-level viremia [65, 66]. The impact of subclinical CMV was reported by Tu et al., in a study showing that CMV-specific CD4 cell activity was associated with better control of CMV viremia and a decreased risk for allograft vasculopathy and rejection [67]. In another study from the same group, aggressive prophylaxis (for the high-risk D+/R− group) was associated with better CMV outcomes than less aggressive prophylaxis (for the lower-risk R+ group), showing that the expected findings were reversed by the intensity of prophylaxis [11]. A non-randomized but intriguing study by Potena et al. recently reported that prophylaxis was associated with less symptomatic CMV disease and smaller changes in maximal intimal thickness than pre-emptive therapy [68]. However this study compared two different eras of CMV prevention at one center, and so these findings await larger and preferably randomized studies for confirmation.
Different immunosuppressive agents can have differential effects on CMV risk. It has long been known that administration of antilymphocyte therapy for rejection markedly raises risk for symptomatic CMV in the weeks following such treatment [69], and that that increased risk can be counterbalanced by administration of antiviral prophylaxis with ganciclovir derivatives during and after this anti-rejection treatment [70]. In addition, the mTOR inhibitor everolimus was associated with significantly lower risk for CMV in a large randomized trial comparing regimens containing two doses of everolimus with a non-everolimus regimen [71], as well as a lower risk for CAV in the everolimus groups [71]. Future studies of novel immunosuppressive agents should ideally include an assessment of the impact of these agents on current rates of CMV infection and disease.
Epstein-Barr Virus (EBV) and Post-transplant Lymphoproliferative Disorder (PTLD)
Epstein-Barr virus is a lymphotropic and oncogenic virus which remains latent in lymphocytes over the lifetime of an infected individual, and can reactivate under the influence of immunosuppression [72]. Transplant immunosuppression reduces EBV-specific immune function, allowing replication of EBV in infected lymphocytes to proceed unchecked, resulting first in a polyclonal lymphoproliferative syndrome, and progressing in some cases to a full-blown monoclonal B-cell lymphoma. Over 90 % of adults have had EBV at some time in the past, reflected in a positive EBV VCA IgG serology at the time of transplant. Consequently, almost all adult donors are also EBV-seropositive. The uncommon EBV D+/R− group is at high risk for primary EBV acquired from the donor, and for transformation to PTLD/lymphoma [72]. Pediatric transplant recipients are more likely than adults to be EBV D+/R− (since they may not yet have had time to acquire EBV infection), and as such, are at high risk for PTLD [73]. In addition, EBV R+ recipients may develop symptomatic EBV/PTLD particularly after intensification of immunosuppression for rejection, such as treatment with antilymphocyte therapy [74]. EBV infection may take the form of asymptomatic viremia, an undifferentiated febrile illness, a mononucleosis-like syndrome, or a monoclonal lymphoma (PTLD) that can affect any organ including the allograft, but is particularly common in the lung, GI tract, central nervous system, and liver. Peripheral lymphadenopathy may not be present. For GI tract lesions, sudden gastrointestinal hemorrhage or perforation may be the presenting symptom. Although overt lymphoma from PTLD is usually associated with high blood viral loads of EBV, there are exceptions where PTLD occurs with low or even undetectable blood viral load [72]. Such cases may be EBV-negative PTLD, or may be EBV-positive when detected in tissue by in situ hybridization (EBER).
Treatment of PTLD involves reduction of immunosuppression, to the extent possible. For heart recipients, this option is limited by the risk of rejection, in contrast to kidney recipients who can go back on dialysis if they lose the allograft. Reduction of immunosuppression generally works best in the setting of EBV viremia before frank lymphoma has developed, but is still worth doing even after biopsy-proven lymphoma has been diagnosed. If reduction of immunosuppression does not reverse the process, treatment of full-blown PTLD generally consists of rituximab-based regimens (rituximab alone, or in combination with chemotherapy regimens such as R-CHOP.) For localized disease, surgery or radiation therapy can be an option. Prior to the rituximab era, the prognosis of PTLD was poor, but has been improved by the addition of rituximab therapy [72].
Prevention of PTLD involves careful use of immunosuppression in high-risk patients, and monitoring of EBV D+/R− patients with quantitative EBV PCR over time [73, 75]. As with pre-emptive therapy for CMV, this approach offers the opportunity to intervene early with reduction of immunosuppression when the EBV PCR turns positive, in hopes of avoiding progression to high viral loads and PTLD [73, 75]. The utility of antiviral therapy in preventing PTLD has been debated, but a body of indirect evidence suggests a potential role for ganciclovir derivatives in this regard [58, 73, 76].
Other Viral Infections
Other viruses in the herpesvirus family share the characteristic of lifelong latency with CMV and EBV. Herpes simplex virus (HSV) is common in the general population, particularly HSV-1 (the oropharyngeal strain of HSV). Reactivation occurs early post-transplant in the form of oral ulcers and occasionally esophagitis, if prophylaxis is not administered. Patients who are seropositive for HSV-2 can experience reactivation in the genital/perianal area. Rarely, HSV can cause other more serious infections such as hepatitis, pneumonitis, and meningoencephalitis. These are uncommonly seen now due to the widespread use of antiviral prophylaxis (ganciclovir derivatives as well as acyclovir and valacyclovir prevent HSV and VZV).
Varicella-zoster virus (VZV) reactivation is common as well, and >90 % of adults are VZV-seropositive. Most zoster reactivations are in the form of zoster (shingles) extending over one to two or occasionally several dermatomes. In severely immunosuppressed patients, disseminated zoster can occur, including both cutaneous and visceral dissemination (involving lungs, liver, central nervous system, and sometimes other organs.) In occasional cases, “rashless” zoster can occur, either with cutaneous symptoms in the absence of a rash, or purely visceral involvement. Diagnosis of either HSV or VZV in the central nervous system rests upon the CSF PCR for those viruses.
In addition to HSV, VZV, CMV, and EBV, the herpesvirus family also includes human herpesviruses 6, 7, and 8. HHV-6 and 7 are the agents of roseola in infants, and seropositivity is almost universal in adults. Reactivation of HHV-6 is common post-transplant and may occur earlier than CMV [77]. It may take the form of asymptomatic viremia, a febrile illness, pancytopenia, or tissue localization in lung, liver, or the central nervous system (meningoencephalitis). HHV-7 produces a similar array of infections though it is less commonly detected post-transplant than HHV-6. HHV-8 is the agent of Kaposi’s sarcoma and may also reactivate post-transplant, although this appears to be uncommon in the US and more frequently seen in certain transplant centers in the Middle East and Europe [78].
Community respiratory viruses are important pathogens post-transplant, as hypoxemia and severe and protracted respiratory illness can result, sometimes requiring mechanical ventilation [79, 80]. Given that RSV infection can be particularly severe in pediatric cardiac patients and transplant recipients, nearly half of pediatric transplant centers in a survey in the U.S. utilize RSV prophylaxis with palivizumab, mostly below age 24 months [81]. In addition, in lung transplant recipients, profound allograft dysfunction can result 3–6 months after resolution of a respiratory viral infection [82]. Multiplex viral panels for diagnosis of respiratory viral infections are available to be performed on either nasopharyngeal viral swabs or bronchoalveolar lavage specimens [83]. These panels often consist of PCR’s for influenza, parainfluenza virus (1, 2, 3), adenovirus, respiratory syncytial virus (RSV), human metapneumovirus (h-MP), and sometimes other viruses. Early diagnosis of influenza and institution of therapy within 48 hours can reduce the risk of ICU admission and severe complications [79]. Antiviral resistance in influenza may vary from year to year, and each year’s updated recommendations from the Centers for Disease Control and Prevention (CDC) should be consulted [84]. Patients with symptomatic RSV, parainfluenza, or hMP infection may be candidates for inhaled or oral ribavirin therapy to prevent worsening lower-tract disease (and allograft dysfunction in lung transplant recipients) [85, 86]. Adenovirus deserves special mention because it was the most common viral genome detected in a pediatric heart transplant study of myocardial biopsies by Shirali et al., in which detection of myocardial viral genomes was associated with allograft dysfunction and adverse events [87]. In this study it was far more commonly detected in myocardial biopsies than was CMV [87]. Adenovirus infection, however, is likely less common in adults.
Parvovirus B19 may reactivate in immunocompromised patients and most frequently presents with severe anemia in the absence of blood loss [88]. The classic presentation with a slapped-cheek rash is usually not seen. Diagnosis is by blood parvovirus PCR and/or a bone marrow biopsy showing characteristic changes. Treatment is with intravenous immunoglobulin as there are currently no available antivirals with parvovirus-specific activity [88].
The gastrointestinal viruses (including norovirus and rotavirus) are very common in the general population, and are acquired through fecal-oral spread or foodborne illness. Although generally short and self-limited infections in healthy people, these viruses can cause protracted syndromes of chronic diarrhea in transplant recipients [89] and should be specifically sought in patients with unexplained diarrhea in whom C. difficile, bacterial enteric pathogens, and stool examination for ova and parasites has been negative.
Hepatitis B (HBV) and hepatitis C (HCV) may have a variety of effects on the heart transplant recipient, depending on the circumstances. Donors positive for hepatitis B surface antigen (HBsAg) are not generally used in the US although a literature from Taiwan demonstrates safe transplantation from such donors with intensive prophylaxis in an endemic region [90]. On the other hand, the “core-positive” donor (HBsAg negative, anti-HBc positive) conveys a much lower risk of HBV transmission (1 in 30 or 1 in 60) which may be further reduced by effective pre-transplant immunization of the recipient and by prophylaxis of HBV-seronegative recipients [91, 92].
Hepatitis C is transmitted highly efficiently from seropositive donors to seropositive recipients (up to 75 % of the time in different studies), and carried a risk of increased cardiac allograft vasculopathy in one study [93] whereas another study showed poorer overall outcomes [94]. Although hepatitis C positive donors are sometimes targeted for HCV+ recipients and/or elderly recipients, the latter study by Gasink et al. demonstrated less successful outcomes with these groups as well [94]. HCV-positive donors should be used with great caution and only with stringent informed consent, preferably in life-threatening situations in which it would be difficult for another donor to be found.
Bacterial Infections Including MRSA, VRE, and Multidrug-Resistant Gram-Negative Bacteria
In the current era, some strains of both Gram-positive and Gram-negative organisms have increasingly developed resistance to standard antibiotics. Methicillin-resistant Staphylococcus aureus (MRSA) has become common in the general community as well as in healthcare-associated infections [7, 25]. Vancomycin-resistant Enterococcus (VRE) has also become a common colonizer of the GI tract particularly in patients with protracted hospitalizations and extensive antibiotic use [9]. More recently, Gram-negative bacilli such as E. coli and Klebsiella pneumoniae that produce extended-spectrum beta-lactamases (ESBL) have become increasingly common, and these strains are resistant to all beta-lactam-related antibiotics except for carbapenems (imipenem, meropenem, ertapenem) [8]. Resistance to quinolones is also on the rise, due to widespread use of quinolones for respiratory and urinary tract infections in the general population. Susceptibility to quinolones can no longer be assumed, as with a patient with fever and pyuria. Most concerning is the rise of carbapenem-resistant organisms such as KPC, which may be resistant to all antibiotics except for amikacin, colistin, and tigecycline [8]. The first two of these agents are nephrotoxic, and tigecycline is not highly active for bacteremic infections. Development of more effective and less toxic antibiotics for these multiresistant Gram-negative organisms would be a welcome development. Awareness of past colonization or infection with these any antimicrobial-resistant organisms in the recipient can inform decisions about empiric therapy for febrile illnesses post-transplant while awaiting culture results. Whether infection or colonization with such organisms should disqualify recipients from transplantation is debated. If past MRSA or VRE or ESBL-Gram negative infection has been treated and is no longer active, most clinicians would not consider these to be contraindications to transplantation, but past infection with more highly-resistant organisms such as KPC may be, given the difficulty of complete eradication of these types of organisms and the limitations of available antibiotic therapy. Studies are currently underway to provide more evidence-based risk assessment in this regard.
Bacterial Infections: Clostridium difficile
C. difficile infection, though known for decades, rose to prominence in 2005 with the advent of a new and more virulent strain which produced an upsurge in infections and an increase in morbidity and mortality [10]. It may be hospital-acquired, or may be triggered by antibiotic usage which alters the balance of normal organisms in the intestine, allowing C. difficile to multiply. The organism produces a toxin which causes inflammation and pseudomembranes of the colonic mucosa, and presents clinically as severe diarrhea which can be accompanied by fever, leukocytosis, and abdominal pain. When C. difficile occurs in the setting of ileus, with increasing abdominal distention and pain rather than diarrhea, the complication of colonic dilatation and perforation is a significant risk. Fulminant infection may require colectomy for control. Infection in transplant recipients is common, partly due to their frequent and lengthy hospitalizations and partly as a result of extensive antibiotic use both pre- and post-transplant in this population [10, 95]. Detection of C. difficile by PCR is more sensitive than the previous enzyme immunoassays for C. difficile toxin. Oral metronidazole remains the initial treatment of choice except in severe infection, in which oral vancomycin is generally used. Oral vancomycin may be substituted if oral metronidazole has not produced improvement. When ileus is present, intravenous metronidazole is used, sometimes in combination with rectal vancomycin enemas or instillation of enteral vancomycin via nasogastric tube. The new drug fidaxomicin appears to be associated with decreased risk of C. difficile recurrences [96], but is expensive. Its use in transplant recipients is currently under study.
Recurrences of C. difficile-associated diarrhea may occur in transplant recipients due to the persistence of C. difficile spores that are unaffected by anti-C. difficile antibiotic therapy and that subsequently germinate and cause recrudescence of the infection, as well as frequent use of antibiotics in this population. Another area of controversy is how long to wait after a C. difficile episode in a recipient on the waiting list, before reactivating their candidacy for transplantation. Further studies of optimal therapy, duration, and prophylaxis of C. difficile would be helpful in the transplant population.
Bacterial Infections: Nocardia, Legionella, Listeria, Salmonella, Tuberculosis, Nontuberculous Mycobacteria
Some bacterial infections are seen principally in immunocompromised patients (e.g. nocardiosis) and some are more severe in their manifestations in immunocompromised patients (e.g. salmonellosis, mycobacterial infection.) Nocardiosis, like fungal infections, likely relates to environmental exposures in the setting of immunosuppression [97]. Most common presentations include pulmonary nodules and nodular infiltrates, with occasional CNS involvement (including space-occupying brain abscesses) and skin and soft-tissue infections. Trimethoprim-sulfamethoxazole prophylaxis (administered for Pneumocystis prevention) provides some but not complete protection.
Legionella pneumonia occurs in the community particularly in older individuals or those with chronic lung disease, but can be particularly severe, multilobar, and rapidly progressive in immunocompromised individuals [98, 99]. It is associated with water sources including water leaks in patient homes, and also hospital water supplies in some centers. Hallmarks of legionellosis include rapid radiographic progression and presence of polymorphonuclear leukocytes but absence of conventional organisms on Gram staining of sputum. It is likely an under-recognized infection in transplant recipients [98, 99]. Culture or PCR for Legionella can be performed as part of an immunocompromised bronchoalveolar lavage panel, and inclusion of a macrolide (e.g. azithromycin) as part of empiric therapy for pneumonia provides Legionella coverage. The urine antigen test detects only Legionella pneumophila type 1, but other species (e. g L. micdadei, L. longbeachae) may cause pneumonia in immunocompromised hosts, so the urine antigen test should not be considered to rule out Legionella [100]. Along with Legionella, other “atypical” organisms such as Mycoplasma pneumoniae and Chlamydophila (formerly Chlamydia) pneumoniae should be considered in the differential diagnosis of severe pneumonia. PCR testing for these organisms is also available, and this group responds to azithromycin, doxycycline, or respiratory quinolones such as moxifloxacin and levofloxacin.
Listeria monocytogenes causes meningitis, bacteremia, and occasionally other infections in infants, the elderly, pregnant women, and immunocompromised patients [101, 102]. Myocarditis in cardiac transplant recipients has been occasionally reported [103]. It is a foodborne illness associated with unpasteurized dairy foods and soft cheeses, deli meats, frankfurters including turkey franks, and other foods as well. A large outbreak in 2012 was traced to contaminated cantaloupe. Other foodborne pathogens may have protracted or unusually severe presentations in transplant recipients, including Salmonella, which is more likely to cause bacteremia and metastatic seeding in this group. Eggs and poultry should always be cooked thoroughly, and utensils and cutting boards thoroughly cleaned.
Tuberculosis is a major problem for transplant programs in endemic areas [104]. In addition to classic pulmonary TB presentations, extrapulmonary, military, and atypical presentations are common in transplant recipients [104–106]. Most post-transplant TB occurs by reactivation of latent TB infection in the recipient, but approximately 4 % is donor-derived [105]. In the past, detection of TB infection relied on the PPD skin test, but anergy in chronically ill or immunocompromised individuals has led to false negative tests in some transplant candidates and recipients. In the last several years, interferon-gamma release assay (IGRA) testing has become available, which detects the patient’s lymphocyte reactivity to TB antigens [30]. The advantages of this test are that it differentiates between Mycobacterium tuberculosis infection (positive) and BCG vaccination (negative) and that it is performed as a single step rather than requiring reassessment in 48 hours as with the skin test. However, the IGRA assay may still be falsely negative in immunocompromised individuals as it depends on cellular immune function. In addition, it must be transported promptly to the laboratory and processed immediately, or an “indeterminate” result may be received.
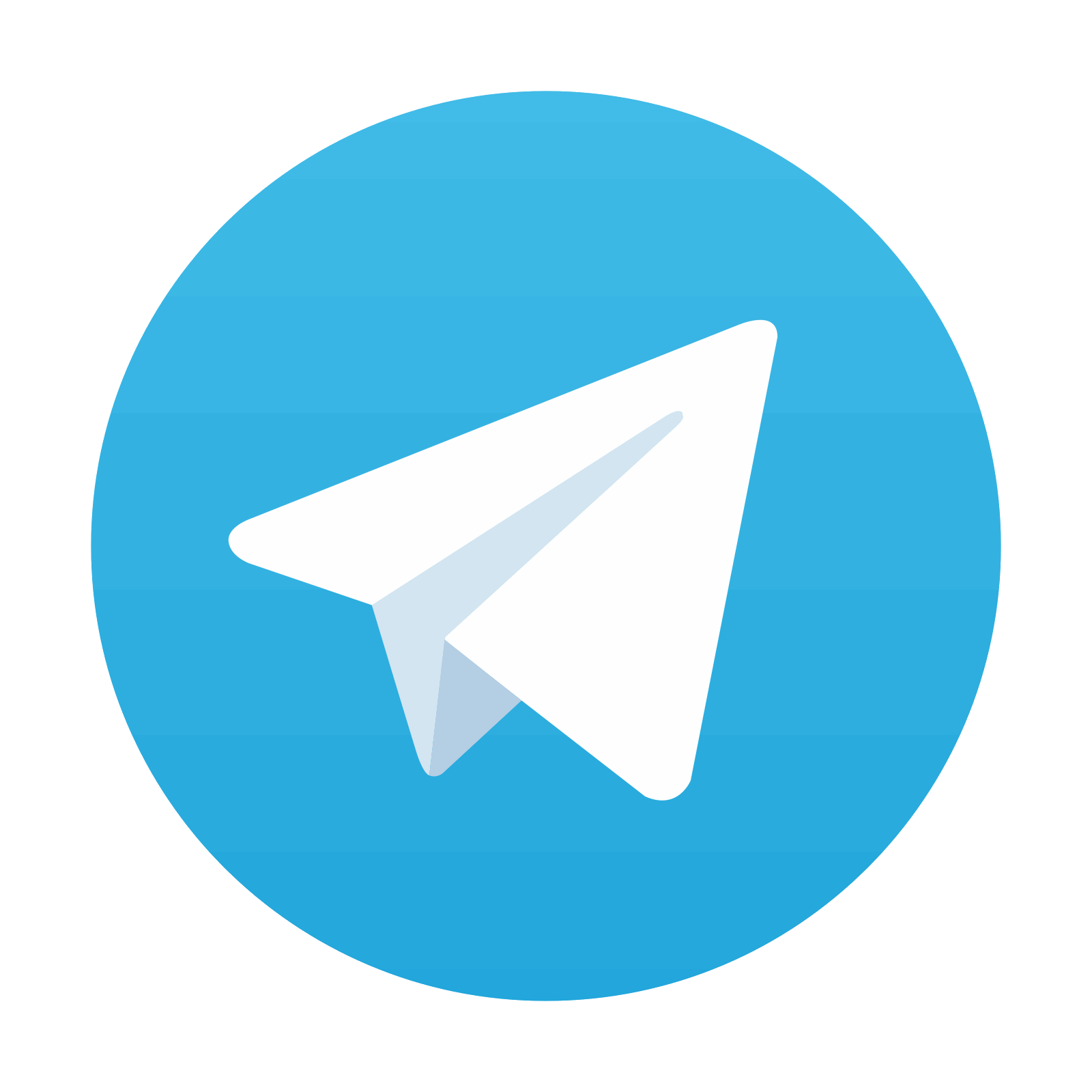
Stay updated, free articles. Join our Telegram channel

Full access? Get Clinical Tree
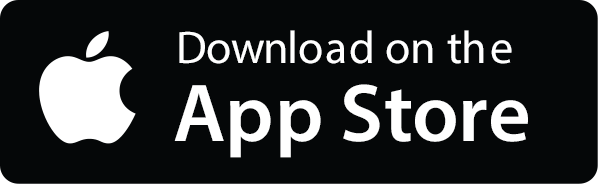
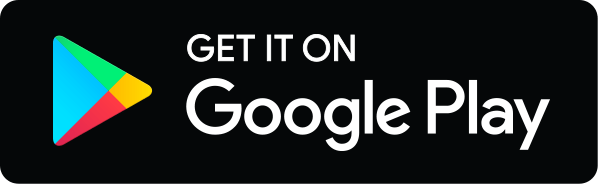