11
Indoor sports
This chapter reviews hazardous respiratory exposures encountered during participation in, or proximity to, indoor sports and the diagnosis, management and prevention of the adverse health effects attributable to them. Respiratory risks vary across sports and across indoor venues (Table 11.1). Exposures and associated health risks are dependent upon multiple factors, including the activities and processes occurring within a venue, proximity to a toxicant point source and, in some circumstances, the ventilation characteristics of the venue. In contrast with exposed populations in most other hazardous environments, indoor sports participants are commonly engaged in vigorous athletic activity, significantly amplifying exposure dose and the associated risk of illness compared with sedentary individuals of comparable health status.
Table 11.1 Indoor sports, pulmonary exposures, and health effects
Sports | Pulmonary exposure | Health effects |
Water sports Swimming Diving Water polo Synchronized swimming Hot tubs Jet pools Saunas | Disinfectants and by-products Organic and inorganic compounds Infectious agents Fluid shifts in body Blunt trauma | Asthma Sensitizer-induced asthma (new onset) Acute irritant induced asthma (new onset) Exacerbation of pre-existing asthma Extrinsic allergic alveolitis Respiratory tract infections Pulmonary edema Pneumothorax Pulmonary contusion |
Ice sports Hockey Figure skating Speed skating Curling | Carbon monoxide Nitrogen dioxide Cold air | Central nervous system and cardiovascular system dysfunction Pneumonitis Asthma exacerbation |
Equestrian | Sandy soils Clay Wood Crumb rubber Chip rubber | Chronic bronchitis Asthma Cough Allergic rhinitis |
Gymnastics Weightlifting Rock climbing Athletics (track and field) | Chalk dust Magnesium carbonate Calcium carbonate | Cough Respiratory tract irritant effects |
Unsurprisingly, exposure assessment and diagnosis of illness attributable to activity in, or proximity to, an indoor sport can be challenging. Diagnostic and management strategies will necessarily differ across sports and across venues. Nevertheless, there are some general tenets and observations that apply to the indoor sports reviewed in this chapter and the respiratory health issues associated with them:
- Indoor sports participants, support staff and spectators experience ambient conditions and respiratory exposures they may not encounter elsewhere.
- Physical activity increases aerobic activity and, by extension, minute ventilation, which amplifies airborne exposures and generally increases the risk of a toxicantrelated health effect.
- The atmospheric microenvironment at the level of play in an indoor sports venue can be uniquely hazardous compared with other areas inside the venue, subjecting sport participants and ground-level support staff to heightened respiratory risk compared with that of individuals (e.g. spectators) who are not in close proximity to the area of play.
- Harmful exposures encountered during participation in indoor sports may go unrecognized because most health effects are nonspecific and because specific biomarkers of exposure and disease are generally not available in clinical practice (with the important exception of carbon monoxide intoxication).
- Athletic impairment resulting from a harmful respiratory exposure encountered during participation in an indoor sport may not be easily appreciated because participants are generally otherwise healthy and have significant cardiopulmonary functional reserve. Accordingly, while low to moderate intensity exposures may adversely affect peak athletic performance, health effects may otherwise be ‘sub-clinical’, thereby eluding detection and prevention.
- The use of masks or respirators is not a practical option under most circumstances for indoor sports participants, in contrast with occupational settings where the use of personal respiratory protection may be a prudent strategy to reduce exposure to airborne pollutants.
- Air contamination in indoor sports venues should be anticipated under standard operations. Appropriate engineering controls, ventilation and monitoring can reduce exposure and the associated risk of illness.
- Very limited information is available about the long-term health effects of chronic, low-intensity exposures resulting from participation in indoor sports.
Over the past several decades, a spectrum of adverse health effects have been attributed to poor indoor air quality encountered in indoor ice arenas. Numerous contaminants and toxicants have been detected, the most important of which, carbon monoxide (CO) and nitrogen dioxide (NO2), are produced during ice resurfacing. Other compounds produced during ice resurfacing, including other oxides of nitrogen, aldehydes, volatile organic compounds and respirable-range particulate matter, may also contribute to disease. The total burden of illness and impairment (transient or chronic) attributable to poor indoor air quality in arenas is unknown, but may be quite significant. One analysis carried out in the late 1990s of 332 rinks in nine countries reported excess concentrations of NO2 in 40% of the rinks.
Ice arenas are found throughout many communities worldwide, especially across Europe and North America. Multiple sports utilize indoor ice arenas, including ice hockey, figure skating, recreational ice skating, short track speed skating and curling. Ice hockey is among the world’s most popular sports with tens of millions of participants in youth and adult leagues worldwide. Since air quality monitoring in ice arenas varies across venues, if present at all, details about the scope of the problem and the public health impact of poor indoor air quality in indoor ice arenas cannot be precisely established. Moreover, since exposures to indoor ice arena air pollutants such as CO and NO2 typically produce nonspecific signs and symptoms and, because many of their health effects are typically short-lived and self-limited after the exposure abates, it is likely that a significant burden of disease attributable to poor ice arena air quality is not recognized.
11.3 Ice arena air pollution: exposures and practical hints when taking a history
Poor indoor air quality in ice arenas may result from a variety of environmental and engineering factors including ventilation system deficiencies, tobacco smoke, faulty heating systems, cleaning chemicals, refrigerants and mold contamination. A discussion of all possible point sources for poor indoor air quality is beyond the purview of this chapter. The principle focus of this section is ice resurfacing equipment exhaust, a major point source for pollutants in most arenas, and the health effects attributable to this exposure.
Most ice resurfacers, including ‘Zamboni machines’, utilize combustion engines that operate with a fossil fuel (e.g. gasoline and propane) and produce exhaust with a mixture of hazardous compounds, including CO and oxides of nitrogen, especially NO2, as well as volatile organic compounds and respirable-range particulate matter. While there is nothing remarkable about the exhaust produced from ice resurfacing equipment, the machines present a special hazard in indoor ice arenas because they operate repeatedly, starting and stopping, in what is essentially a confined space. Just as running a motor vehicle inside of a closed garage can produce a hazardous exposure setting, the operation of ice resurfacing equipment inside a poorly ventilated arena can result in significant indoor air pollution.
The concentration of compounds present in the exhaust of ice resurfacing equipment is partially a function of the temperature of the engine. CO production is greatest when an engine has been newly started and is running at a relatively low temperature. As the machine warms, CO production decreases while the production of oxides of nitrogen, including NO2, increases. Accordingly, concentrations of exhaust pollutants from ice resurfacing equipment inside an arena will, in part, depend upon on the frequency of equipment start-stop cycles and the duration that the engine runs during each cycle, as well as venue characteristics such as size and air exchange rate.
The clinical syndromes attributable to ice arena air pollution are generally abrupt in onset and unfold over a relatively short time frame: minutes to hours. Health effects resulting from long-term exposure to low-level concentrations of airborne pollutants encountered in indoor ice arenas are not well established. While the relationships between chronic exposure to exhaust pollutants and cardiopulmonary and neurocognitive outcomes are of epidemiologic interest, it unlikely that a causal link between low-level indoor ice arena air pollution and a chronic health effect can be established with confidence at the level of the single individual.
Operators of ice resurfacing equipment are at obvious risk of exposure to equipment exhaust as a consequence of their proximity to the point source and their repeated exposure to exhaust throughout their work shift. Athletes and sports participants are also at special risk because of the duration of exposure (i.e. time spent on the ice) and especially because of their increased ventilation and metabolic demands resulting from vigorous aerobic exercise.
Confirmation of exposure to CO can be established through testing of peripheral blood specimens for carboxyhemoglobin in a clinical laboratory. In contrast, detection of exposure to most other toxic gases, including NO2, requires air sampling in the field by an industrial hygienist.
11.4 Indoor ice arena toxicant syndromes
Diagnosis of an adverse health effect attributable to airborne pollutants in an indoor ice arena begins with the history. First, the clinician must suspect that an illness or symptom may be attributable to a toxic environmental exposure. Second, the clinician must act on the suspicion and gain data that supports or refutes the hypothesis. As is the case with other exposure settings and environmental illnesses, the patient may be the first person to consider a possible relationship between either a specific toxicant or exposure setting and the illness. In such instances, the process of establishing a diagnosis will be facilitated by the patient’s presenting complaint (e.g. ‘I am always the first person on the ice after the Zamboni machine finishes resurfacing because I like to take advantage of the excellent skating conditions, but I have noticed, lately, I develop headaches and dizziness when I start skating which tend to get better on their own.’). Patient recognition of causation commonly occurs when there is an extreme exposure with a manifestly clear cause and effect relationship (e.g. a major mishap, accident or evident equipment malfunction), or a cluster of cases (e.g. multiple affected teammates) that point to etiology. In cases where causation is not obvious, the clinician will need to make the link between the exposure setting and possible etiologic toxicants and the disorder.
Workers, athletes and spectators may be exposed to ice arena toxicants. Accordingly, the occupational history may elucidate causation for some affected individuals (i.e. employees who work in ice arenas, such as drivers of ice resurfacing machines) and, in turn, facilitate diagnosis of the illness. However, in others, an environmental history that considers hobbies, pastimes and athletic activities (i.e. participation in an ice sport) will be necessary to identify the exposure-disorder relationship.
The major disorders attributable to ice arena air pollution are CO intoxication and NO2 pneumonitis. Strictly defined, carbon monoxide intoxication is not a disorder of the lungs. While the respiratory tract is the portal of entry for carbon monoxide, adverse health effects caused by carbon monoxide largely cause dysfunction of other organ systems. In contrast, the respiratory tract is both the route of exposure and the target organ in NO2 related illness.
Carbon monoxide is the gas most often reported as a cause of intoxication in indoor ice arenas. CO is a nonirritating, tasteless, odorless and colorless gas formed by hydrocarbon combustion and an important component of the exhaust of ice resurfacing equipment. CO diffuses readily across the alveolar-capillary interface and binds with avidity to hemoglobin, forming carboxyhemoglobin (COHb), which results in compromised oxygen transport and utilization. In addition to competing with oxygen for the heme moiety of hemoglobin, CO also compromises the ability of the other heme moieties with bound oxygen to offload oxygen to peripheral tissues. CO also interferes with peripheral oxygen utilization. These CO effects are independent of those on hemoglobin and oxygen delivery.
The uptake of CO increases with exercise. Studies of CO concentrations in hockey players have found a linear relationship between COHb levels and exposure time and concentrations of ambient CO. Investigators found a 1.0% rise in COHb for each 10 parts per million (ppm) of ambient CO in an indoor arena during a 90 minute hockey game. Other investigators found a CO concentration of approximately 22 ppm raised COHb levels in hockey players to greater than 3% from a baseline of 1%, on average. Concentrations of CO exceeding 20 ppm in indoor ice arenas have been described in numerous reports. The United States Environmental Protection Agency National Ambient Air Quality Standard for CO is 9 ppm time weighted average over 8 h.
The clinical findings of CO poisoning are nonspecific and vary based on severity of intoxication. COHb levels less than 2.5% are typically inconsequential. COHb concentrations between 2.5 and 10% may affect vision, manual dexterity and exercise tolerance. Concentrations greater than 20% can cause headaches, dizziness, malaise and nausea. Severe CO intoxication may result in seizures, coma and myocardial ischemia, and dysrhythmias. Severe intoxication in an indoor ice arena would only result from an unusual mishap such as the running (e.g. idling) of an ice resurfacing engine inside of a small confined space within the larger enclosed ice arena.
Acute CO poisoning is first suspected on the basis of a suggestive history. Confirmation of intoxication requires a COHb level measured by co-oximetry of a blood gas sample. Moderate- to high-intensity exposures, especially in patients with underlying coronary artery disease, warrant further evaluation to assess for myocardial ischemia, including an electrocardiogram and measurement of cardiac enzymes. The most important interventions in the management of CO intoxication are removal of the affected individual from the CO source and institution of high-flow oxygen by face mask. The role of hyperbaric oxygen therapy remains controversial and is unlikely to be required to manage the low-level intoxication typically encountered in indoor ice arenas. Indeed, because symptoms of exposure (e.g. headaches and diminished exercise capacity) may be interpreted as a nuisance by the affected individual rather than a major health problem, an important consideration is the possibility of repeated low-level intoxication that goes undetected. Moreover, an affected individual (e.g. enthusiastic athlete) may not be receptive to counseling to avoid the potentially toxic environment. Suspicion of ice arena intoxication should prompt assessments of engineering controls at the venue.
Nitrogen dioxide is another gas that may be encountered in hazardous concentrations in indoor ice arenas, although disorders attributable to NO2 exposure have not been reported as commonly as CO intoxication. NO2 is a reddish-brown gas with a characteristic sharp, biting odor. The principle point source for NO2 is the internal combustion engine of an ice resurfacing machine. NO2 is heavier than most of the constituent gases in air and tends to remain close to the surface of the ice. NO2 causes respiratory tract injury that may range from mild irritation with minimal short-term clinical consequences to acute lung injury with significant symptoms and respiratory impairment. Chest tightness, wheezing, asthma flares and cough may be caused by NO2 inhalation. NO2 is relatively water-insoluble and therefore does not typically produce classic symptoms of irritation upon initial exposure. The lack of ‘warning symptoms’ can result in significant exposure over hours before any symptoms of injury develop. NO2 is a powerful oxidant and also slowly combines with water in the respiratory tract to produce nitric and nitrous acid which may contribute to lung injury.
In one of the best described exposure incidents, an outbreak of NO2-induced respiratory illness occurred among players and spectators at two high school hockey games played at an indoor ice arena in Minnesota, USA. The source of the NO2 was the malfunctioning engine of the ice resurfacer. Affected individuals experienced acute onset of cough, hemoptysis and/or dyspnea during attendance or within 48 hours of attending a hockey game. In all, 116 cases were identified among hockey players, cheerleaders and band members who attended the two games. Members of two hockey teams had spirometry performed at 10 days and 2 months after exposure; no significant compromise in lung function was documented.
Swedish investigators reported two cases of toxic pneumonitis with delayed onset in hockey players due to NO2 exposure during an ice hockey game in an indoor arena. Signs and symptoms included cough, hemoptysis, dyspnea and reduced peak expiratory flow. The chest radiographs showed reticulo-nodular opacities in one player and patchy consolidation in the second player. Repeat chest radiographs obtained within one week of exposure were normal in both cases.
It is unknown to what extent athletic performance may be adversely affected by ‘subclinical’ exposures to NO2 in indoor ice arenas.
There is no specific therapy for NO2 pneumonitis. Bronchodilators are appropriate to treat airflow obstruction and inhaled corticosteroids may reduce airway inflammation. The role of systemic corticosteroids is not established, but should be considered with high-intensity exposures.
Mixed exhaust exposures have also been linked with respiratory illness. Chronic cough and dyspnea were reported in ice hockey players after an acute exposure to a faulty ice resurfacer. In one report of 16 previously healthy hockey players, more than half complained of cough and dyspnea on exertion 6 months post-exposure. All had normal pulmonary function tests, exercise studies and chest computed tomographs. Additional testing using impulse oscillometry showed evidence of increased airway resistance and small airway disease.
For concerns about ice arena toxicant exposure, emergent consultation with a medical toxicologist can be helpful. The United States Poison Control Network is available at (+ 1) 800-222-1222, 24 hours a day, 7 days a week. This is the telephone number for every poison center in the United States. Calls to this number are automatically connected to one of 65 poison centers across the country. The World Health Organization’s list of international poison centers is available at www.who.int/ipcs/poisons/centre/directory/en.
11.5 Standards, guidelines and public health considerations
Standards addressing air quality in indoor ice arenas have been developed and published. A Canadian agency has recommended average concentrations for CO and NO2 to be lower than those in work environments. The United States National Ambient Air Quality Standard for carbon monoxide is 9 ppm time-weighted average over 8 hours and 35 ppm over 1 hour, and for NO2 0.053 ppm (53 parts per billion, ppb) annual average. Additional Canadian guidelines for the operation of ice resurfacers with internal combustion engines include: (1) regular testing of ice resurfacer equipment emissions; (2) installation of monitors to measure the concentration of toxic gases at the opening of the exhaust pipe; (3) use of a catalytic purifier; (4) operation of resurfacers by an experienced operator to minimize time of equipment operation; (5) 90 minute intervals between resurfacing; (6) open rink doors during and after resurfacing; (6) air quality standards of 1 hour maximal allowable exposure to CO at 20 ppm and NO2 at 200 ppb; (7) regular monitoring of ambient air in indoor ice rinks; (7) installation of warning signs that describe the potential symptoms and hazards of CO and NO2 poisoning; (8) educational seminars for rink staff, coaches, parents and patrons of ice rinks; and (9) development of an emergency plan in the case of an intoxication incident.
A potentially transformative engineering control has been the development of the electric ice resurfacing machine which does not emit exhaust fumes. As they are more costly than internal combustion models, replacement of internal combustion models with electric-powered ones may not be possible in many ice arenas, but if carried out would result in improved indoor air quality.
11.6 Cold air-exacerbated asthma and dyspnea
Cold air can produce bronchoconstriction and provoke asthma exacerbations. Indeed, cold air challenges are used in some clinical pulmonary function laboratories to test for the presence of bronchial hyperresponsiveness, a hallmark of asthma. The combination of exposure to cold air and intense exercise contributes to dyspnea and provokes asthma in a significant minority of endurance athletes. The prevalence of asthma in elite athletes has been reported to be as high as 20%. Some individuals intolerant of intense exercise in cold air may avoid cold weather sports, resulting in a ‘healthy athlete effect’ and an underestimation of the total population affected by the co-exposures. Diagnosis of bronchoconstriction provoked by exercise in cold air may be facilitated by serial peak expiratory flow monitoring before, during and following exposure. The use of inhaled beta agonists before participation in cold weather sports is advisable for individuals with evidence of exercise-induced bronchoconstriction, and should be considered for athletes who experience dyspnea out of proportion to their exertion, or dyspnea that does not resolve with cessation of exercise. A trial of beta-2 agonist therapy may help to confirm the diagnosis of asthma, as well as offer relief to the sports participant.
Importantly, not all dyspnea associated with exercise in cold air is due to broncho-constriction. Reflex mechanisms in some individuals that reduce minute ventilation in response to cold air inhalation may contribute to dyspnea. Bronchodilators are not likely to be beneficial in these settings because bronchoconstriction is not a hallmark of the condition.
Swimming is a widely popular sport among people of all ages. In 2005 it was estimated that the number of Americans swimming for fitness was about 16 million. Learning how to swim is mandatory at the elementary school level in the UK and swimming is among the most popular forms of exercise among adults. Other competitive and recreational indoor water sports include water polo, springboard diving and synchronized swimming. Spas and fitness centers with hot water tubs, jet pools and saunas are additional indoor settings where the hazards of indoor water sports may be encountered.
While the respiratory disorders associated with water sports are seen in both the indoor and outdoor setting, the indoor setting presents special risks. This is attributable to multiple factors including reduced ventilation, differences in pool maintenance requirements and micro-climates around indoor swimming arenas with higher temperature and humidity. The population at highest risk includes those who use such facilities with regularity, or workers such as lifeguards, trainers and cleaning and maintenance staff.
The water in swimming pools, hot water tubs and spas has many organic and inorganic chemicals and contaminants. These may be present in water from the source, brought to the pool by swimmers and bathers, or introduced into the water intentionally by the staff for disinfection purposes (Table 11.2). The source water is usually drinking-quality water from the local municipality and contains organic materials like humic acid, disinfection by-products, lime and alkalis, phosphates and monochloramines. Swimmers and bathers introduce sweat, urine, lotions, cosmetics and soap residues into pool water which includes a variety of nitrogen-based compounds in the form of ammonia, urea, amino acids and creatinine (Table 11.3). The concentration of natural body products in the water, such as sweat or urine, is dependent on factors such as the amount of time and type of exertion while in water (for sweat and urine) and the demographic of bathers (with children passing more urine while in the water). Even though most of the nitrogen is excreted (or secreted) in the form of urea, swimming pools have a higher concentration of ammonia-based nitrates due to the reaction of various nitrogen compounds with chlorine-based products. A study using a model pool to simulate the fate of various natural compounds found that organic carbon, chloramines and trihalomethanes reached a steady state after 200-500 hours of operation and only nitrate-based compounds accumulated.
Table 11.2 Primary disinfectants used in swimming pools
Professional or large pools | Smaller pools, hot tubs, saunas | Domestic pools |
Chlorinated compounds Chlorine gas Calcium and sodium hypochlorite Chlorinated isocyanurates Chlorine dioxide Bromochlorodimethylhydantoin Ozone gas Ultra-violet radiation | Bromine Liquid bromine Sodium bromide Sodium hypochlorite Lithium hypochlorite | Various combinations of bromide and hypochlorite Ultra-violet radiation Ozone gas Iodine |
Table 11.3 By-products of disinfection compounds commonly found in swimming pools
Disinfectant | Disinfectant by-product |
Chlorine | Trihalomethanes Haloacetic acids Haloacetonitriles Chlorate Chloramines |
Bromine | Bromoform |
Chlorine dioxide | Chlorite Chlorate |
Ozone | Bromate Aldehydes Ketones Bromoform Brominated acetic acids |
Bromochlorodimethylhydantoin | Bromal hydrate Bromate Bromamines |
The water in the pools requires continuous treatment, a process divided into filtration, coagulation, pH correction and disinfection. The choice of disinfectant is determined by factors like effectiveness, ease of handling and use, and ability to monitor levels of the chemicals employed. Chlorine-based products are the most widely used disinfectants since they have a residual effect after treatment. This is not the case with ozone or ultra-violet radiation, which kill microorganisms immediately, but need to be combined with chlorine- or bromine-based products for continual effect. Chlorinebased products include chlorine gas, sodium hypochlorite, calcium hypochlorite, lithium hypochlorite and chlorinated isocyanurates. Guidelines for acceptable ‘free’ (residual) chlorine levels vary by pool size and region, and it is generally recommended that levels for public pools be between 1 and 3 mg/l and those for public hot tubs may be slightly higher, but less than 5 mg/l. Concentrations higher than 5 mg/l may lead to higher incidence of mucous membrane irritation and should therefore be avoided. Higher chlorine levels (up to 20 mg/l) may be required infrequently to prevent biofilm buildup or to treat gross contamination. Chlorinated isocyanurates dissociate into free chlorine and cyanuric acid products and it is recommended that their level be kept below 117 mg/l.
Chlorine dioxide disintegrates into chlorite and chlorate (and not into free chlorine, therefore it is not considered chlorine-based). Bromochlorodimethylhydantoin and a combination of sodium bromide and hypochlorite are both bromine based disinfectants used in indoor pool settings and their recommended levels are usually less than 2.0-2.5 mg/l. Ozone and ultraviolet radiation purify water as it passes through a treatment room without any residual disinfectant effect. The leakage of ozone (from the generators or tanks) is a serious concern given the highly irritating properties of the gas, and it is recommended that a separate de-ozonation process be included. The recommended concentration of ozone in air is less than 0.12 mg/m3.
Disinfectants react with organic compounds excreted by bathers and form various by-products. Of all the chlorine-derived disinfection by-products found in the pools, trihalomethanes (THMs) such as chloroform and haloacetic acids like di-chloroacetic acid and trichloroacetic acid are present in the greatest concentrations. Inorganic bromine leads to the formation of brominated THMs. Both chlorine and bromine react with ammonia (from human source) to form haloamines like chloramines (monochloramine, dichloramine and nitrogen trichloride) and bromamines. Ozone reacts with organic matter to produce aldehydes and carboxylic acids. Studies have found a linear correlation between the number of people using the pool and the concentration of THMs.
THMs are volatile in nature and are also found in the air above indoor pools. The concentration of THMs in the air is a function of their concentration in the water, the water temperature and the amount of splashing and surface disturbance. The concentration at different levels in the air depends on ventilation, building size and air circulation. Swimmers inhale these substances from just above the water’s surface, and the volume of air inhaled is a function of the intensity of effort and time spent in the pool. Inhalation is probably the major route of uptake of volatile components such as THMs One analysis of swimmers found that chlorinated swimming pool water lead to increased levels of THMs in both plasma and alveolar air, but the concentration in alveolar air rapidly fell after exiting the pool environment. This exposure becomes especially significant among lifeguards, coaches and pool maintenance staff.
Exposure to volatile chloramines and bromamines (especially nitrogen trichloride and tribromide) can result in ocular and respiratory symptoms, particularly those related to irritation of mucous membranes (lacrimation, injection, cough, rhinorrhea, wheezing). The pulmonary symptoms are worse in patients with underlying bronchial asthma or increased bronchial hyperreactivity. Nitrogen chloride has an intense and unpleasant odor even at lower concentrations. The effect of exposure to chlorinated compounds is dependent upon the intensity (concentration) and duration of exposure. While high-intensity exposure can cause chemical burns, asthma, airway edema and chemical pneumonitis, lower-intensity exposures lead to lacrimation and nasopharyngeal irritation.
11.9 Diseases and health effects
The more common pulmonary disorders attributable to indoor water sports include: (1) asthma – exacerbations of pre-existing asthma and irritant-induced asthma; (2) extrinsic allergic alveolitis – also known as hypersensitivity pneumonitis; (3) nontuberculous mycobacterial infection (especially Mycobacterium avium and Mycobacterium intracellulare); (4) swimming-induced pulmonary edema; and (5) pulmonary contusion and pneumothorax.
In an unscientific survey of 220 participants at the interactive occupational grand round of the 1998 annual European Respiratory Society meeting in Geneva, about half the audience had either definitely or probably seen cases of swimming pool asthma. Most of these participants spent <10% of their practice time treating occupational lung disorders. Swimming pool cleaning staff are at special risk of respiratory tract injury and disease resulting from mishaps involving disinfectants, including mixing incompatibilities (e.g. bleach with ammonia or acids).
A Dutch study collected samples from 38 swimming pool facilities and questionnaires from 624 workers at these facilities. They found a higher incidence of pulmonary atopy-related complaints among the workers compared with the general population. High levels of chloramine exposure correlated with greater frequency of pulmonary complaints. However, there are conflicting data that have resulted in children with asthma being encouraged to take up swimming because of the perceived benefit of breathing moist air.
Elite swimmers have a higher incidence of bronchial hyperresponsiveness compared with the general population. Certain investigators have hypothesized that swimming in chlorinated pools potentiates lung damage and may be partly responsible for the increasing incidence of asthma in Western societies. This conclusion is based on the observation of elevated levels of serum pneumoproteins in children who frequently attend chlorinated swimming pools compared with controls. The elevated levels of serum pneumoproteins are believed to reflect increased alveolar-capillary permeability and early lung injury.
The United States Centers for Disease Control and Prevention recently reported an analysis of a large number of guests at two separate hotels who were exposed to high levels of chloramine-like compounds near the indoor swimming pool area and experienced significant ocular and respiratory symptoms. Events such as these underscore the importance of staff training, proper ventilation and appropriate handling of compounds used for disinfection. Public health departments are encouraged to adhere to guidelines for cleaning and maintenance of swimming pools, to ensure ventilation and minimize exposure to volatile compounds, and to educate the public about proper pool hygiene.
Exposure to certain chlorine-derived compounds or ozone can cause or exacerbate asthma. Asthma symptoms including cough, wheezing, chest tightness, dyspnea at rest or with exercise and reduced exercise capacity are usually temporally linked to either routine use of the pool or to exposures to high concentrations of chemicals (either unregulated or during shock treatment). Patients often feel better during days when they are not in the pool environment. The physical and laboratory findings in these patients may include wheezing, a prolonged expiratory phase, hypocapnea and hypoxemia on arterial blood gas measurement, especially during an exacerbation. Serial assessments of peak expiratory flow rate and pulmonary function testing are commonly part of the evaluation. Radiographs are often unremarkable, though hyperinflation may be seen in severe cases. If patients are symptomatic, but have nondiagnostic pulmonary function test results, bronchoprovocation testing with methacholine (methacholine challenge test) can provide important diagnostic information.
Management consists of avoiding the pool and the surrounding environment if the symptoms are severe and debilitating. If it appears that a large number of users are developing similar symptoms, then the pool maintenance staff must test the water quality, ensure proper treatment of pools by using recommended practices, and also test air quality around the pool area with the objective that proper ventilation is maintained. Pharmacologic treatment is no different than that for other cases of asthma and includes corticosteroids and bronchodilators. Systemic corticosteroids may be used for severe exacerbations.
11.9.2 Acute irritant induced asthma
The indoor pool environment presents the risk of an accidental high intensity exposure to water treatment chemicals. Such an exposure may cause severe respiratory tract irritation resulting in acute irritant-induced asthma. The hallmark features of irritantinduced asthma include a high-intensity exposure resulting in the abrupt onset of symptoms of increased airway reactivity, often within minutes of exposure. An important clinical reminder for physicians is that some patients manifest worsening symptoms after initial improvement or after a latency period of 6-12 hours after exposure. Therefore it is important to monitor individuals involved in major chemical spills and mishaps in a controlled setting for at least 24 hours. Acute irritant-induced asthma may improve over time or persist. It is necessary to prevent recurrent high intensity exposure to the offending agent. However, low-level exposure to the irritant agent is typically tolerated, so exposed individuals can typically return to the pool environment. Pharmacological management is similar to that for other presentations of asthma.
11.10 Extrinsic allergic alveolitis (hypersensitivity pneumonitis)
Extrinsic allergic alveolitis (EAA), also known as hypersensitivity pneumonitis, is an immunologic non-IgE mediated inflammatory lung disease that results from prior sensitization and subsequent exposure to specific organic and inorganic antigens in the atmosphere. It has been studied and investigated in both outdoor and indoor swimming pools, hot water tubs, spas and saunas. Factors associated with EAA include:
(1) inadequate filtration and treatment of water harboring microorganisms either in a biofilm or as bacterial fragments;
(2) higher temperature and humidity in such facilities, facilitating the growth of microorganisms;
(3) intermittent use of the pool, tub or spa that promotes formation of biofilm;
(4) spas generating water sprays and air bubbles that dissipate widely;
(5) spray particle size in the respirable range (less than 10 μm in diameter);
(6) prolonged and repeated exposures.
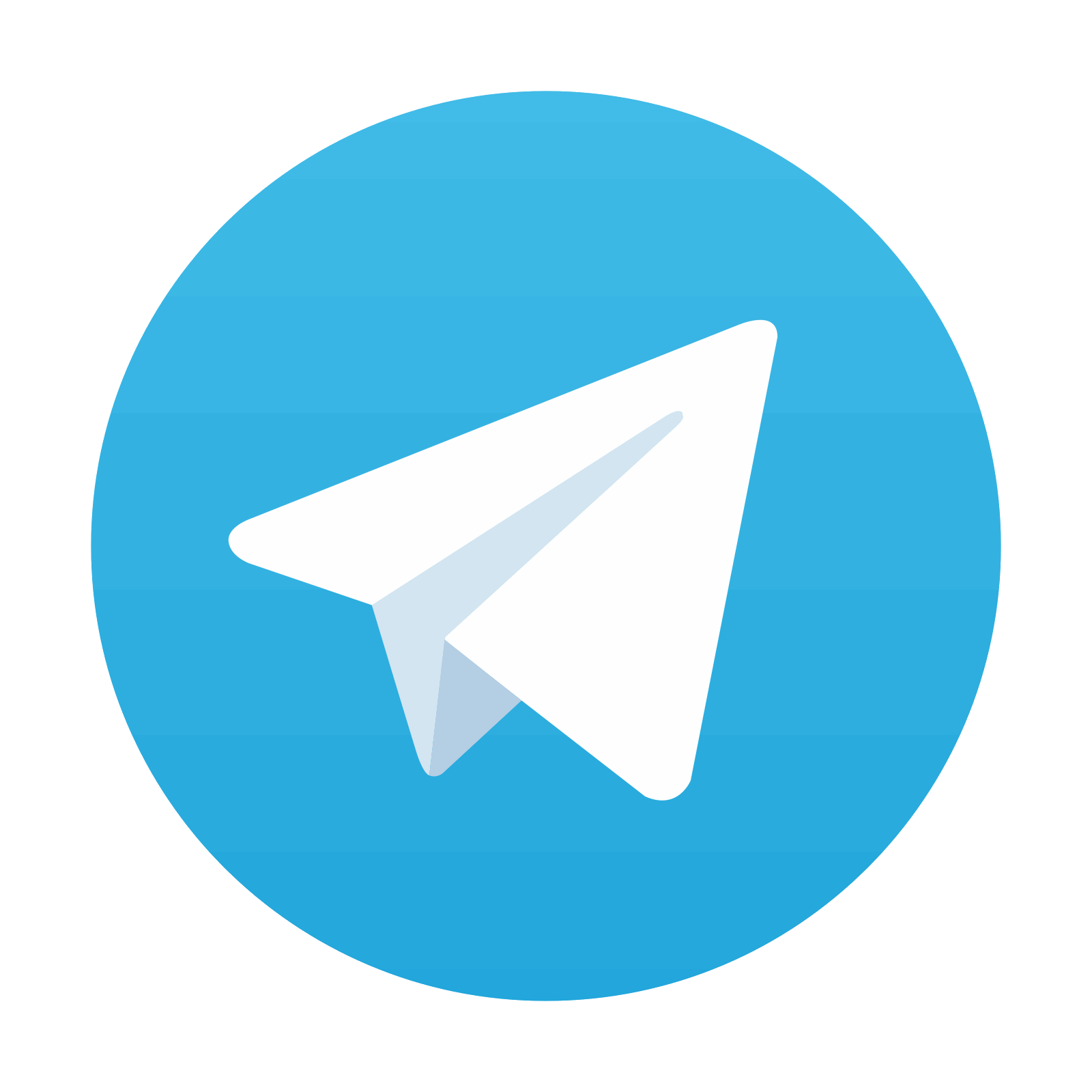
Stay updated, free articles. Join our Telegram channel

Full access? Get Clinical Tree
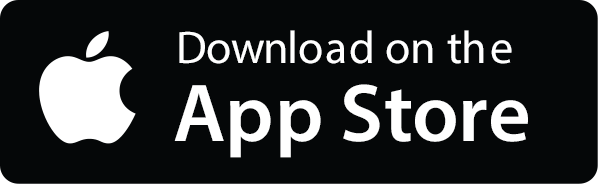
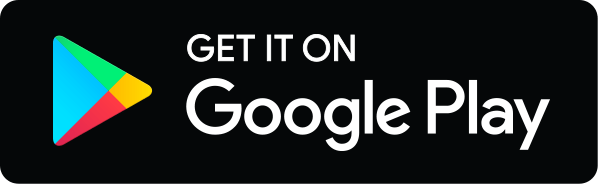