Background
Accurate assessment of left ventricular (LV) function in patients with atrial fibrillation (AF) remains difficult, mainly because of the beat-to-beat variability of many echocardiographic parameters. The aim of this study was to assess the hypothesis that LV function can be estimated from an index-beat echocardiographic assessment in patients with AF using myocardial strain and strain rate.
Methods
A prospective study was conducted to assess 25 patients with AF (mean age, 66 ± 10 years). Peak systolic longitudinal strain (LS) and peak diastolic longitudinal strain rate (dSR) were measured using two different methods: (1) mean LS and dSR, the averages of instantaneous LS and dSR over 10 sec, and (2) index-beat LS and dSR, calculated when the ratio of the preceding (RR1) to the pre-preceding (RR2) interval was 1 (range, 0.96–1.04). These variables were compared with simultaneously measured LV pressure parameters using Millar catheters.
Results
There was a positive linear relationship between mean LS and index-beat LS at RR1/RR2 = 1 ( r = 0.94, P < .001) and a positive linear relationship between mean dSR and index-beat dSR ( r = 0.69, P < .001). Index-beat LS was correlated with the maximal positive derivative of LV pressure (peak +dP/dt) ( r = −0.73, P < .001). Index-beat dSR was correlated with the time constant of isovolumic LV pressure decay (τ) ( r = −0.63, P < .001). To investigate the independent predictors of τ, a stepwise multilinear regression analysis showed that index-beat dSR was the best predictor of τ.
Conclusions
Index-beat parameters accurately reflect the mean values of parameters in patients with AF. These noninvasively obtained index-beat parameters are useful to assess surrogate LV function even in patients with AF.
Atrial fibrillation (AF) is the most common arrhythmia, and the number of patients with AF has increased with the rapid aging of society. Furthermore, chronic AF is a major contributor to long-term disability and mortality. Clinical assessment of left ventricular (LV) contractility during AF is difficult and unreliable because myocardial contractility changes constantly from beat to beat. Previous studies using animal models have shown that beat-to-beat changes in contractility in AF may be estimated using the ratio of the preceding (RR1) to the pre-preceding (RR2) interval, which determines mechanical restitution and potentiation. It was reported that LV systolic function showed a strong positive linear correlation with the RR1/RR2 ratio and that the value of end-systolic maximum elastance at an RR1/RR2 ratio of 1 could accurately estimate average LV contractility during AF. There have been several recent studies showing the relationship between the RR interval and various systolic parameters obtained by echocardiography.
In addition to systolic function, the evaluation of LV diastolic function during AF is also extremely challenging. The ratio of early diastolic transmitral flow velocity (E) to early diastolic mitral annular velocity (e′), E/e′, has been widely used to estimate LV filling pressure in patients with AF. In a previous study, we showed that the single-beat E/e′ ratio at an RR1/RR2 ratio of 1 reflects the average value. Single-beat assessment may be applicable to the clinical setting for the estimation of LV diastolic function in patients with AF. However, in our previous study, subjects were limited to patients with preserved LV systolic function, and diagnostic accuracy was not high.
Two-dimensional (2D) echocardiographic speckle tracking and tissue Doppler can accurately measure myocardial strain and strain rate correctly. Using such a system, the goal of the present study was to measure peak systolic longi-tudinal strain (LS) and peak diastolic longitudinal strain rate (dSR) in patients undergoing left-sided cardiac catheterization to determine whether such measures can predict invasively measured LV relaxation and filling pressure. In addition, we wanted to determine if index-beat strain and strain rate values at an RR1/RR2 ratio of 1 (range, 0.96–1.04) reflect the average strain and strain rate in patients with AF.
Methods
Study Population
We designed a prospective study to assess patients with AF. Consecutive patients who had dyspnea or angina or LV asynergy and were referred to the cardiac catheterization laboratory were approached for recruitment. Twenty-five patients (17 man, eight women; mean age, 66 ± 10 years) with chronic AF underwent simultaneous LV pressure measurements and Doppler examinations. Patients with valvular stenosis, significant valvular regurgitation, severe heart failure (New York Heart Association functional class IV), and unstable angina were excluded. Conventional medical therapies such as angiotensin II receptor blockers (32%), β-blockers (48%), and calcium antagonists (36%) were continued throughout the study. The institutional review board of the University of Tokushima approved the study protocol, and written informed consent was obtained from all subjects.
Echocardiography
A total of 25 echocardiographic examinations were performed simultaneously with the cardiac catheterization procedures, with patients lying in the supine position. Two-dimensional transthoracic echocardiographic examinations were performed using a commercial ultrasound machine (iE33; Philips Medical Systems, Bothell, WA). All images were stored digitally for offline playback and analysis. LV end-diastolic and end-systolic dimensions and fractional shortening were measured on M-mode echocardiography. The LV ejection fraction and left atrial (LA) volume were calculated using Simpson’s method with 2D images. LA volume was indexed to body surface area. Transmitral flow and mitral annular motion velocities were recorded in the apical four-chamber view. Transmitral flow velocity was recorded at the tip of the mitral leaflet. Mitral annular velocities were recorded at the mitral annulus of the LV lateral and septal wall using pulsed-wave Doppler tissue imaging. The following parameters were measured: E wave, e′, systolic mitral annular velocity (s′), and the deceleration time of the E wave. Echocardiographic parameters were measured for five consecutive beats and then averaged.
Strain Imaging
Two-dimensional color tissue Doppler recordings with second-harmonic imaging were collected at high frame rates (100–170 frames/sec). Two-dimensional images were recorded in the apical four-chamber view at a frame rate of 60 to 85 frames/sec during a brief breath hold. All measurements were performed using commercially available equipment and software (QLAB version 7.0; Philips Medical Systems, Andover, MA). Measurement of e′, peak systolic longitudinal strain rate, and dSR using 2D color Doppler tissue imaging was performed offline. We measured dSR at the time of mitral valve opening on 2D echocardiographic images. Strain signals were obtained from the basal, mid, and apical LV segments using oval regions of interest (10 × 6 mm) placed in the midwall position and included manual tracking to optimize the segmental strain signal. Peak systolic longitudinal strain rate and dSR were the average diastolic strain rate in all regions. Measurement of LS was calculated as the percentage of lengthening or shortening using 2D speckle tracking. When a cardiac cycle with a high-quality image was selected, a region of interest for speckle tracking was defined first at end-diastole using a semiautomated border detection method in the four-chamber view. The data were then processed automatically by the system, and myocardial strain curves were generated ( Figure 1 ). LS was the average systolic strain in all regions. LS and dSR were calculated using the following two methods: (1) mean LS and dSR were the averages of LS and dSR over 10 sec, and (2) index-beat LS and dSR were calculated at an RR1/RR2 ratio of 1 (range, 0.96–1.04). About 10 to 20 beats were included over 10 sec. These numbers were needed for assessing the mean value with well rate controlled or poor rate controlled AF. All measurements and calculations were performed without knowledge of the invasive hemodynamic measurements.

Hemodynamic Measurements
Left-heart catheterization was performed through a radial or brachial approach. The study was performed before left ventriculography and coronary angiography. A 5-Fr Millar transducer (Millar Instruments, Inc., Houston, TX) with a single lumen was introduced into the left ventricle. The maximum and minimum value of the first derivative of LV pressure (peak +dP/dt and peak −dP/dt), the time constant of isovolumic LV pressure decay (τ), and LV end-diastolic pressure (LVEDP) were determined offline after digitizing the LV pressure curve. Although τ is often calculated on the basis of the assumption of a zero asymptote by nonlinear least squares parameter estimation, we calculated τ from the logarithm of LV pressure using the method of Weiss et al.
Statistical Analysis
Values are expressed as mean ± SD. Comparisons of values within the same individuals were performed using paired t tests. Correlations were determined using linear regression analysis. Potential determinants of peak +dP/dt, peak −dP/dt, τ, and LVEDP were identified using univariate linear regression analysis, and all identified predictors were then entered in a stepwise manner into a multivariate linear regression model. We found no evidence for collinearity problems in our model (variance inflation factor values < 2). Bland-Altman analysis was used as a means of assessing systematic differences between two measurements. P values < .05 were considered statistically significant. All statistical analyses, except for the Bland-Altman analysis, were performed using SPSS version 18.0 (SPSS, Inc., Chicago, IL).
Reproducibility
Interobserver and intraobserver variability for LS and dSR were examined. Measurements were performed in a group of 10 randomly selected subjects by one observer, then repeated on 2 separate days by two investigators who were unaware of the other’s measurements and study time point. Reproducibility was expressed as the mean percentage error (absolute difference divided by the average of the two observations).
Results
Clinical Characteristics
The mean age of the patients was 66 ± 10 years. Sixty-six percent of the patients had hypertension. The mean heart rate of the patients was 74 ± 15 beats/min. Heart rate was controlled in most patients using β-adrenergic blockers or calcium channel antagonists. Forty-eight percent of the patients had hypertensive heart disease; 32% had ischemic heart disease. The mean LA volume of the patients was large (LA volume index, 47 ± 22 mL/m 2 ; Table 1 ).
Variable | Value |
---|---|
Age (y) | 66 ± 10 |
Men/women | 17/8 |
Body surface area (m 2 ) | 1.67 ± 0.17 |
Heart rate (beats/min) | 74 ± 15 |
Systolic blood pressure (mm Hg) | 131 ± 16 |
Diastolic blood pressure (mm Hg) | 76 ± 12 |
New York Heart Association functional class | |
I | 10 |
II | 12 |
III | 3 |
Echocardiographic data | |
End-diastolic LV dimension (mm) | 52 ± 8 |
End-systolic LV dimension (mm) | 36 ± 9 |
Interventricular septal thickness (mm) | 10 ± 3 |
LV posterior wall thickness (mm) | 10 ± 2 |
LV fractional shortening (%) | 29 ± 6 |
LV mass index (g/m 2 ) | 127 ± 49 |
LA volume index (mL/m 2 ) | 47 ± 22 |
Pulmonary artery systolic pressure (mm Hg) | 31 ± 9 |
Inferior vena cava dimension (mm) | 13 ± 4 |
LV end-diastolic volume (mL) | 80 ± 31 |
LV end-systolic volume (mL) | 38 ± 27 |
LV ejection fraction (%) | 53 ± 14 |
E (cm/sec) | 87 ± 16 |
E-wave deceleration time (msec) | 125 ± 25 |
s′ (cm/sec) | 6.5 ± 1.5 |
e′ (cm/sec) | 7.3 ± 2.2 |
E/e′ ratio | 12.9 ± 4.0 |
Peak systolic longitudinal strain rate (1/sec) | −0.66 ± 0.15 |
Mean LS (%) | −16.8 ± 2.4 |
Index-beat LS (%) | −16.9 ± 2.3 |
Mean dSR (1/sec) | 0.65 ± 0.15 |
Index-beat dSR (1/sec) | 0.68 ± 0.12 |
Invasive data | |
τ (msec) | 45 ± 8 |
LVEDP (mmHg) | 12 ± 4 |
Peak +dP/dt (mm Hg/sec) | 1,191 ± 296 |
Peak −dP/dt (mm Hg/sec) | −1,316 ± 304 |
Beat-to-Beat Variability and Index-Beat Measurements in dSR and LS
There was a strong positive linear relationship between mean LS and index-beat LS at an RR1/RR2 ratio of 1 ( r = 0.94, P < .001; Figure 2 ). There was a moderate positive linear correlation between mean dSR and index-beat dSR at an RR1/RR2 ratio of 1 ( r = 0.69, P < .001; Figure 3 ). The Bland-Altman analysis is shown in Figures 2 and 3 . In addition, we evaluated the relationships between each LS and dSR and RR1, RR2, and the RR1/RR2 ratio for every individual cardiac cycle occurring over the 20-sec recording interval in each patient. There was a positive linear relationship between LS and RR1 or RR1/RR2 and a negative relationship with RR2. In all patients, LS was correlated significantly with RR1/RR2 ( r = 0.32–0.70, P < .05); it was also correlated significantly with RR1 ( r = 0.25–0.65) and with RR2 ( r = 0.21–0.53). Although there was also a positive linear relationship between dSR and RR1 or RR1/RR2 and a negative relationship with RR2, these relationships were weak. The dSR was correlated significantly with RR1/RR2 ( r = 0.20–0.51) and with RR1 ( r = 0.14–0.41); there was a trend toward a correlation between dSR and RR2 ( r = 0.15–0.34).


Relationship between dP/dt and Echocardiographic Parameters
Peak +dP/dt was correlated with ejection fraction, s′, peak systolic longitudinal strain rate, and index-beat LS. In each patient, LS was correlated well with peak +dP/dt in each heartbeat ( r = 0.64–0.85, all P values < .01). To investigate the independent predictors of peak +dP/dt, a stepwise multilinear regression analysis was performed with several echocardiographic variables. This analysis showed that index-beat LS was the best predictor of peak +dP/dt ( Table 2 ). The intraobserver variability for the measurement of mean LS was 6.6 ± 8.8%, and the interobserver variability was 7.2 ± 9.1%. Peak −dP/dt correlated with index-beat dSR. To investigate the independent predictors of peak −dP/dt, a stepwise multilinear regression analysis was performed with several echocardiographic variables. This analysis showed that index-beat dSR was the best independent predictor of peak −dP/dt ( Table 3 ).
Variable | Univariate regression analysis | Multivariate regression analysis | ||
---|---|---|---|---|
r | P | t | P | |
Fractional shortening | 0.29 | .16 | — | NS |
Ejection fraction | 0.49 | .013 | — | NS |
s′ | 0.56 | .003 | — | NS |
Peak systolic longitudinal strain rate | −0.61 | .001 | — | NS |
Index-beat LS | −0.73 | <.001 | −5.2 | <.001 |
Variable | Univariate regression analysis | Multivariate regression analysis | ||
---|---|---|---|---|
r | P | t | P | |
LV mass index | 0.38 | .079 | — | NS |
LA volume index | 0.19 | .484 | — | NS |
E wave | −0.03 | .927 | — | NS |
E-wave deceleration time | −0.08 | .686 | — | NS |
e′ | −0.34 | .105 | — | NS |
Index-beat dSR | −0.67 | .002 | −4.4 | <.001 |
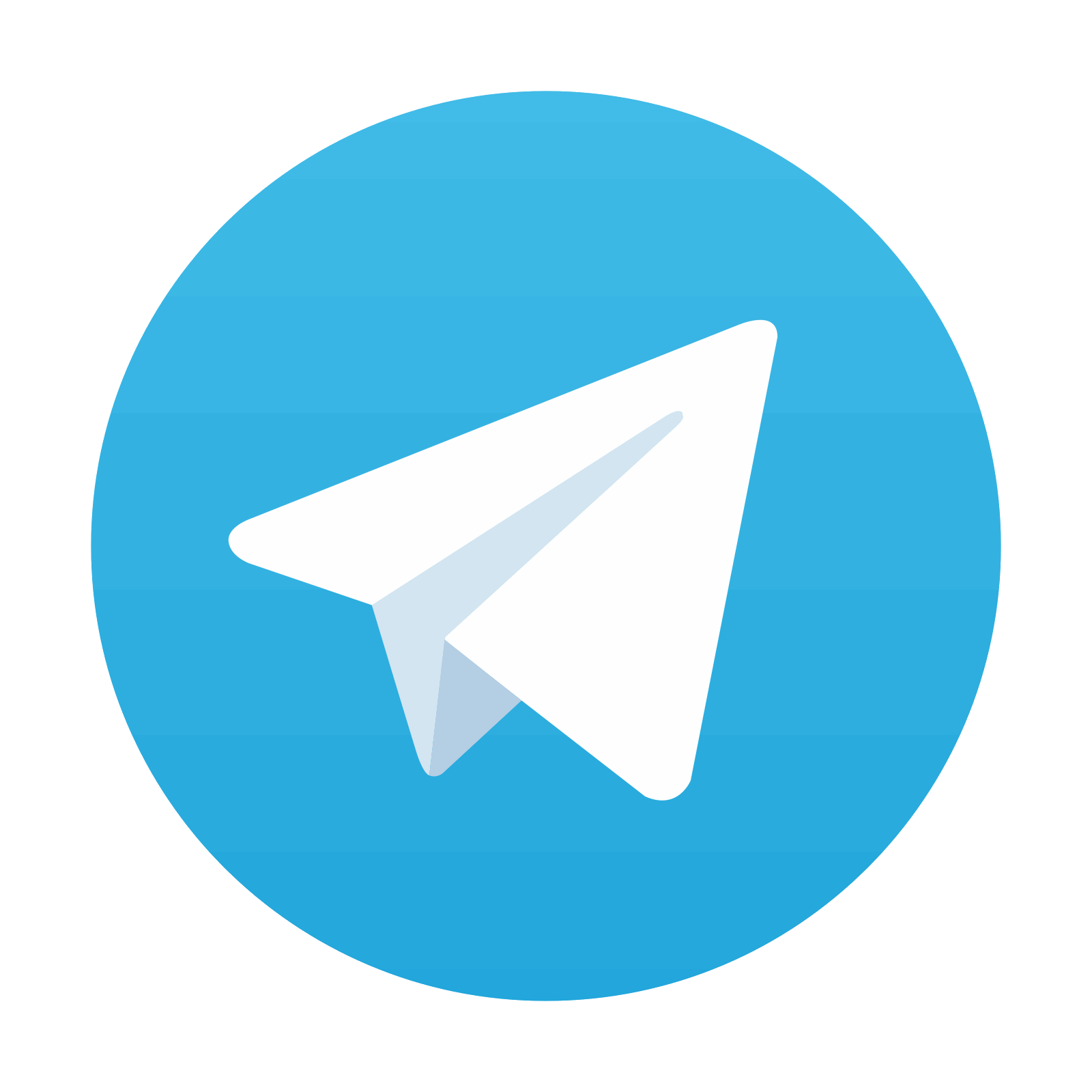
Stay updated, free articles. Join our Telegram channel

Full access? Get Clinical Tree
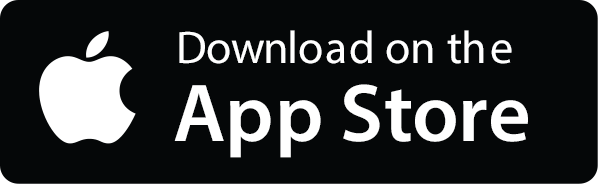
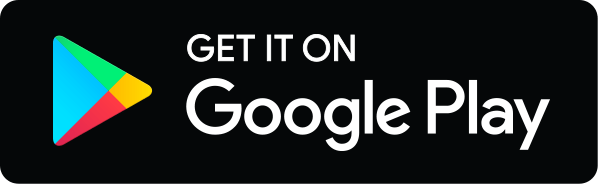
