Background
Chronic kidney disease (CKD) is associated with increased cardiovascular mortality and morbidity, particularly ischemic heart disease and cardiomyopathy. Newer echocardiographic techniques such as myocardial strain analysis provides the opportunity to detect early myocardial dysfunction. The aim of this study was to examine echocardiographic parameters, in particular left atrial (LA) function and volume, in patients with CKD. A further aim was to determine echocardiographic parameters that are sensitive to detect cardiovascular involvement in early CKD.
Methods
Seventy-six patients with stage 3 CKD (estimated glomerular filtration rate, 30–59 mL/min/1.73 m 2 ) with hypertension and/or diabetes mellitus, without any previous cardiac illness, were prospectively recruited. These patients were compared with subjects matched for age, sex, and risk factors (hypertension and/or diabetes mellitus) with normal renal function and 76 healthy age-matched control subjects. Two-dimensional strain analyses of the left atrium and left ventricle were performed. Comprehensive echocardiographic examinations were performed in all participants, and traditional echocardiographic parameters including indexed LA volume (LAVI) and two-dimensional strain analysis of the left ventricle and left atrium were performed in all participants. Differences among the three groups on demographic, clinical, and echocardiographic parameters were examined.
Results
LA systolic strain (20.9 ± 6.3% vs 27.4 ± 7.9%, P < .0001) and systolic and late diastolic strain rates were altered in the CKD group, while early diastolic strain rate was similar to that in the risk factor–matched group. LAVI was significantly larger in the CKD group compared with the risk factor–matched group and healthy control subjects (38.5 ± 10 vs 31.2 ± 9 vs 22.3 ± 5 mL/m 2 , P < .0001). LV strain as well as LV systolic and early diastolic strain rates were similar in the CKD and risk factor–matched groups. LV late diastolic strain rate, a surrogate measure of LA contractile function, was, however, reduced in the CKD group. Forward logistic regression analysis showed LA global strain to be the most sensitive predictor for the presence of CKD, followed by LAVI; though LV late diastolic strain rate was reduced in the CKD group, it was not an independent predictor. Furthermore, the addition of LA strain to traditional echocardiographic parameters significantly increased the predictive power to detect cardiovascular involvement (C statistic = 0.65 vs C statistic = 0.84, P < .0001). Increased LAVI, reduced left ventricular global strain, and the presence of CKD were independent predictors of LA strain, while left ventricular mass index, E/e′ ratio, and the presence of CKD were predictors of LAVI.
Conclusion
LA strain and LAVI are more sensitive parameters than traditional echocardiographic parameters as well as left ventricular strain in patients with early CKD. LA strain and LAVI may be useful to detect myocardial involvement in stage 3 CKD, and LA alterations may be consequent to increased activation of the renin-angiotensin-aldosterone pathway, causing myocardial fibrosis in CKD.
Highlights
- •
Patients with stage 3 CKD with hypertension and diabetes were compared with a risk-matched group.
- •
LA global strain and systolic and late diastolic strain rates were reduced in the CKD group.
- •
LAVI was larger in the CKD group.
- •
LA strain is a sensitive tool to detect myocardial involvement in CKD.
- •
LA strain has incremental predictive value compared with other echocardiographic parameters in CKD.
Chronic kidney disease (CKD), an important emerging global health issue, is associated with increased cardiovascular mortality and morbidity, particularly related to ischemic heart disease and cardiomyopathy.
Cardiovascular risk in patients with CKD is multifactorial. Both CKD and cardiovascular disease (CVD) share the common traditional risk factors, such as diabetes mellitus (DM) and hypertension (HT). Importantly, traditional risk factors, such the Framingham risk score, often underestimate cardiovascular risk in patients with CKD. Underestimation of true cardiovascular risk in patients with CKD can be explained by nontraditional risk factors; structural and biochemical abnormalities occur more commonly in patients with CKD and increase the risk for CVD.
We used the modified National Kidney Foundation classification of CKD, based on estimated glomerular filtration rate (eGFR) ( Table 1 ). All stages of kidney disease have a high risk for the development of adverse CVD ; however, >11% risk for adverse cardiovascular has been reported in stage 3 CKD.
Stage | Description | eGFR(mL/min/1.73 m 2 ) |
---|---|---|
1 | Kidney damage with normal or increased GFR | ≥90 |
2 | Kidney damage with mild reduced GFR | 60–89 |
3 | Moderately decreased GFR | 30–59 |
4 | Severely decreased GFR | 15–29 |
5 | Kidney failure | <15 |
Conventional echocardiography evaluates cardiac structure and function and remains a common examination performed in almost all patients with CKD. However, conventional echocardiographic parameters have limited sensitivity, with changes evident only once overt cardiac involvement has occurred. It is well documented that left ventricular (LV) hypertrophy and coronary artery disease are common in patients with end-stage renal disease. LV strain was also shown to be reduced in patients with CKD, mainly in end-stage renal failure (ESRF). Tripepi et al . demonstrated that changes in left atrial (LA) volume predict cardiovascular events in dialysis patients, independent of baseline LA volume or LV mass. They concluded monitoring LA size is useful for monitoring cardiovascular risk in patients with ESRF. However, there are limited data regarding LA function assessed by strain parameters and LA volume in early CKD.
Activation of the renin-angiotensin-aldosterone system (RAAS) in CKD caused myocardial fibrosis in an animal model. Saito et al . demonstrated that myocardial fibrosis can be detected by two-dimensional (2D) strain. Given that the atrium has thinner walls than the ventricle, it is possible that alterations in myocardial deformation may be detected earlier in the atrium using strain analysis.
Two-dimensional strain is an angle-independent, semiautomated technique that evaluates LA function. The aim of our study was to investigate the value of LA volume and 2D strain analysis in the detection of myocardial involvement in early CKD in patients with coexistent DM and/or HT. We hypothesized that atrial dysfunction in patients with CKD can be detected at an early stage and that CKD may result in myocardial dysfunction, independent and additive to the effects of coexistent DM and HT. We also hypothesized that LA strain analysis may be more sensitive than LA volume indexed to body surface area (LAVI) or LV strain parameters in patients with CKD to detect myocardial involvement.
Methods
Seventy-six patients with stage 3 CKD (eGFR, 30–59 mL/min/1.73 m 2 by the Modification of Diet in Renal Disease formula) with HT or DM (or both) and without any previous cardiac illness were prospectively recruited and compared with subjects matched for age, sex, and risk factors (HT and/or DM) with normal renal function and 76 healthy age-matched control subjects.
Study Population
The final study group comprised 228 subjects. Patients with CKD with HT and/or DM ( n = 76) were recruited prospectively from the renal outpatient clinic at Liverpool Hospital (Sydney, Australia). CKD staging was done according to standard criteria ; the etiology for CKD was HT ( n = 29), diabetic nephropathy ( n = 31), drug related, renal calculus, chronic inflammatory nephropathy, glomerulonephropathy, ischemic nephropathy, chronic granulomatous nephropathy, and single kidney ( n = 2 for each) and hepatitis and polycystic kidney disease ( n = 1 each). We selected patients with stage 3 CKD because patients with eGFRs < 60 mL/min/1.73 m 2 have previously been shown to have an increased prevalence of CVD. Two patients with CKD were excluded from the final analysis because they had positive results on stress electrocardiography for exercise-induced ischemia, and 74 patients were used in the final analysis.
All patients with CKD were screened for coexistent HT and/or DM. None of these patients had previous cardiovascular, peripheral vascular, or cerebrovascular disease. All study subjects were in sinus rhythm, and none had any histories of atrial fibrillation. The patients with CKD were regularly followed by their renal physicians and were optimally treated for their blood pressure and DM. All patients with HT were on one or more antihypertensive medications, with systolic pressure < 140 mm Hg and diastolic pressure < 90 mm Hg. All diabetic patients were either on oral hypoglycemic agents and/or insulin, and their glycated hemoglobin levels were <7%. All patients with CKD underwent baseline transthoracic echocardiography to assess LV function, LV mass, and valvular abnormalities in addition to stress echocardiography to rule out latent ischemia.
Because patients with CKD commonly have a high prevalence of HT and/or DM, a risk factor–matched control group was identified from a clinical database at Canberra Hospital. These patients were subjects with HT and/or DM with normal renal function, who were also age and gender matched to the CKD group. These patients were on antihypertensive agents and oral hypoglycemic agents or insulin. None of these patients had histories of CVD, including specifically atrial arrhythmias or ischemic heart disease. These subjects were treated for their HT and DM by their treating physicians, and recruitment into this study did not alter their management.
The healthy control subjects were selected from a population-based cohort study, who were carefully screened for histories of cardiovascular, peripheral vascular, or cerebrovascular disease and for the presence of cardiovascular risk factors including HT and DM. They were normotensive and had normal results on electrocardiography and echocardiography, and they were not receiving any cardioactive medications. Using a computer-based program, suitable subjects from the database were randomly selected to match the CKD study group.
This study was approved by Human Research and Ethics Committee South Western Area Health Service (HREC/09/LPOOL/53). All participants provided written informed consent.
Echocardiography
Comprehensive transthoracic echocardiographic examinations were performed using a Vivid 9 (GE Healthcare, Little Chalfont, United Kingdom) system with subjects in the left lateral position. Images obtained included 2D, color, pulsed- and continuous-wave Doppler, and Doppler tissue imaging (DTI) according to standard practice. Zoomed left atrium and left ventricle were obtained in four- and two-chamber views at high frame rates (>70 frames/sec), and three consecutive cardiac cycles were stored. LV ejection fraction (LVEF) was calculated using Simpson’s biplane method. LV mass was calculated per American Society of Echocardiography criteria and indexed to body surface area (LVMI). Patients with greater than mild mitral or aortic valve disease were excluded. None of the patients had more than mild mitral annular calcification.
All transthoracic echocardiograms were analyzed at Liverpool Hospital, and all measurements were performed by two readers (K.K.K. and L.T.), blinded to patient groups. All echocardiographic examinations were performed on a similar ultrasound platform (Vivid 9), and similar comprehensive protocols were used. Similar echocardiographic exclusion criteria were applied to all three groups.
Traditional Measurements
Pulsed Doppler transmitral flow was obtained with the sample volume placed at the mitral leaflet tips in the apical four-chamber view. Peak E and A velocity and the E-wave deceleration time were measured, and the E/A ratio was calculated. DTI velocities s′ (systolic), e′ (early diastole), and a′ (late diastole) were measured with the sample volume placed at the septal and lateral mitral annulus, and averages were calculated. Patients were classified into different groups on the basis of diastolic function using standard echocardiographic parameters, including peak E velocity, peak e′ velocity, the E/A ratio, and deceleration time. Patients were divided into four groups on the basis of diastolic function, (1) normal, (2) impaired relaxation, (3) pseudonormal, and (4) restrictive, per standard criteria.
Apical four- and two-chamber zoomed LA images were used to measure maximum LA volume using Simpson’s biplane method at end-systole. LA volume was indexed to body surface area (LAVI). Averages of three measurements were used for all parameters.
Two-Dimensional Speckle-Tracking LA and LV Strain Imaging
Two-dimensional LA strain analysis was performed using customized computer software (EchoPAC; GE Vingmed Ultrasound AS, Horten, Norway) from four- and two-chamber LA images acquired at high frame rates (>70 frames/sec), as reported previously. The endocardial border was manually traced in end-systole, and the software automatically tracked the myocardial region of interest, with QRS gating as has been previously described. The width of the region of interest was manually adjusted (by decreasing the region of interest for the thin-walled atrium) to ensure proper tracking of the myocardial wall. Peak systolic strain measurement was obtained ( Figure 1 A). Systolic strain rate (SRs), early diastolic strain rate (SRe), and late diastolic strain rate (SRa) were measured ( Figure 1 B).

LV myocardial strain was similarly measured from apical four- and two-chamber images (six segments in each view), obtained at high frame rates (>70 frames/sec) ( Figure 2 ). An average of segmental strain from 12 LV segments was calculated as a measure of LV strain.

Statistical Analysis
All continuous variables are expressed as mean ± SD, while categorical variables are expressed as percentages. An independent-samples t test was performed to evaluate differences between the CKD group and risk factor–matched control subjects. Differences among the three groups (patients with CKD, risk factor–matched control subjects, and healthy subjects) were examined using one-way analysis of variance with post hoc Bonferroni analysis. Significant univariate predictors for the effect of group (i.e., presence of CKD) were entered into a logistic regression model to determine independent predictors in CKD. Logistic regression analysis was performed using SAS version 9.2 (SAS Institute Inc, Cary, North Carolina) to determine the significant echocardiographic predictors for patients with CKD. To further compare the incremental predictive value of LA strain over traditional echocardiographic parameters, a baseline model containing significant echocardiographic covariates was constructed, and a logistic regression model was used to obtain the C statistic. Analysis was performed separately for strain and strain rate with univariate correlates, to eliminate the effect of colinearity between LA strain and strain rate. Inter- and intraobserver variability was assessed using intraclass correlation coefficients (ICCs) with SPSS for Windows version 22 (SPSS, Inc, Chicago, Illinois).
Results
Baseline characteristics of the three groups are presented in Table 2 . Two thirds of patients (67%) in the CKD group ( n = 74) and risk factor–matched control group ( n = 76) were men, compared with 27 (36%) in the healthy control group ( n = 76). The mean age of the patients with CKD was 65 ± 16 years, similar to the two comparator groups. As expected, the CKD group and risk factor–matched group had higher body surface areas and body mass indexes compared with healthy control subjects, with no significant difference between the CKD and risk factor–matched control groups ( Table 2 ). The risk factor–matched control group had higher blood pressure compared with both the CKD and normal groups. More patients in the CKD group were on β–blockers (18 vs 11, P = .002) as well as angiotensin-converting enzyme (ACE) inhibitors (25 vs 17, P = .007) compared with the risk factor–matched group. Six patients with CKD were on spironolactone; however, none in the risk factor–matched control group were on spironolactone.
Parameter | CKD group | Risk factor–matched group | Healthy control subjects | P |
---|---|---|---|---|
Age (y) | 65.5 ± 16 | 65.3 ± 10 | 61.7 ± 8 | .08 |
Men | 48 (65%) | 50 (65%) | 26 (34%) | <.001 ∗ |
Systemic HT | 69 (93%) | 70 (92%) | 0 | .59 |
DM | 35 (47%) | 36 (47%) | 0 | .98 |
Body surface area (m 2 ) | 1.94 ± 0.24 | 1.97 ± 0.24 | 1.82 ± 0.22 | <.0001 ∗ |
Body mass index (kg/m 2 ) | 29.9 ± 6.7 | 30.1 ± 5.3 | 26 ± 4.5 | <.0001 ∗ |
Systolic blood pressure (mm Hg) | 127 ± 18 | 150 ± 18 | 123 ± 12 | <.0001 † |
Diastolic blood pressure (mm Hg) | 72 ± 8 | 85 ± 9 | 75 ± 9 | <.0001 † |
Peak A (m/sec) | 0.78 ± 0.17 | 0.75 ± 0.2 | .68 ± 0.17 | .003 ∗ |
A VTI (cm) | 8.8 ± 2.3 | 8.9 ± 2.3 | 9.3 ± 2.9 | .5 |
LVEF (%) | 65.8 ± 6.5 | 65.8 ± 6.5 | 60 ± 5 | <.0001 ∗ |
LVMI (g/m 2 ) | 97.1 ± 31.7 | 104.7 ± 30.9 | 85.1 ± 27.6 | <.0001 ∗ |
LAVI (mL/m 2 ) | 38.5 ± 10.3 | 31.2 ± 8.6 | 22.3 ± 4.5 | <.0001 ∗ † |
s′ (cm/sec) | 7 ± 1.5 | 7.3 ± 1.8 | 7.5 ± 1.5 | .16 |
e′ (cm/sec) | 6.3 ± 1.6 | 6.9 ± 2.6 | 8 ± 2 | <.0001 ∗ |
a′ (cm/sec) | 9.4 ± 2.1 | 9.5 ± 2 | 10.3 ± 1.9 | .009 ∗ |
E/e′ | 12 ± 3.8 | 11.1 ± 4.9 | 8.9 ± 2.5 | <.0001 ∗ |
E/A ratio | 0.94 ± 0.24 | 0.99 ± 0.39 | 1.03 ± 0.24 | .19 |
∗ P < .05 versus healthy control subjects.
Traditional Echocardiographic Parameters
LVEFs were normal in all three groups; LVMI was 97 ± 32 g/m 2 in the CKD group, 104 ± 31 g/m 2 in the risk factor–matched control group, and 85 ± 28 g/m 2 in healthy control subjects, which was also within the normal range (43–95 g/m 2 in women and 49–115 g/m 2 in men). Even though LVMI was similar between patients with CKD and risk factor–matched control subjects, LVMI was higher than in healthy control subjects ( Table 2 ). Only four subjects in CKD group had normal diastolic function; 35 had impaired relaxation, 33 had pseudonormal relaxation, and two had restrictive patterns. In contrast, 18% of patients in the risk factor–matched control group had normal diastolic function, 45% had impaired relaxation, 37% had pseudonormal relaxation, and none had a restrictive pattern. The healthy control subjects had either normal diastolic function or impaired relaxation patterns. DTI s′ velocity was similar in the three groups, but both early (e′) and late (a′) diastolic velocities on DTI were significantly different between healthy control subjects and patients with CKD and risk factor–matched control subjects. E/e′ ratios were higher in the CKD group than that observed in risk factor–matched control or healthy control subjects ( Table 2 ). However, none of these parameters could differentiate patients with CKD from the risk factor–matched control subjects.
LA Parameters
LA strain, SRs (reservoir function), and SRa (contractile function) were reduced in the CKD group ( Tables 3 and 4 ), while LA conduit function (as measured by SRe) was similar to that in the comparator group. LAVI was significantly larger in the CKD group, compared with both risk factor–matched and healthy control subjects. We used current reported values of increased LAVI (>34 mL/m 2 ) and the lower limit for LA strain (<34%) reported in the literature. When applying these normal cutoffs to the CKD group, 29 patients (38%) with CKD had normal LA volumes (<34 mL/m 2 ), whereas only two patients (3%) had normal LA global strain of >34%.
LA strain parameter | CKD group | Risk factor–matched group | Healthy control subjects | P |
---|---|---|---|---|
LA GS (%) | 20.9 ± 6.3 | 27.4 ± 7.9 | 36.8 ± 9 | <.0001 ∗ † |
LA SRs (sec −1 ) | 1.1 ± 0.3 | 1.2 ± 0.3 | 1.9 ± 0.5 | <.0001 ∗ † |
LA SRe (sec −1 ) | 1 ± 0.3 | 1.1 ± 0.4 | 1.6 ± 0.5 | <.0001 ∗ |
LA SRa (sec −1 ) | 1.5 ± 0.4 | 1.8 ± 0.6 | 2.3 ± 0.8 | <.0001 ∗ † |
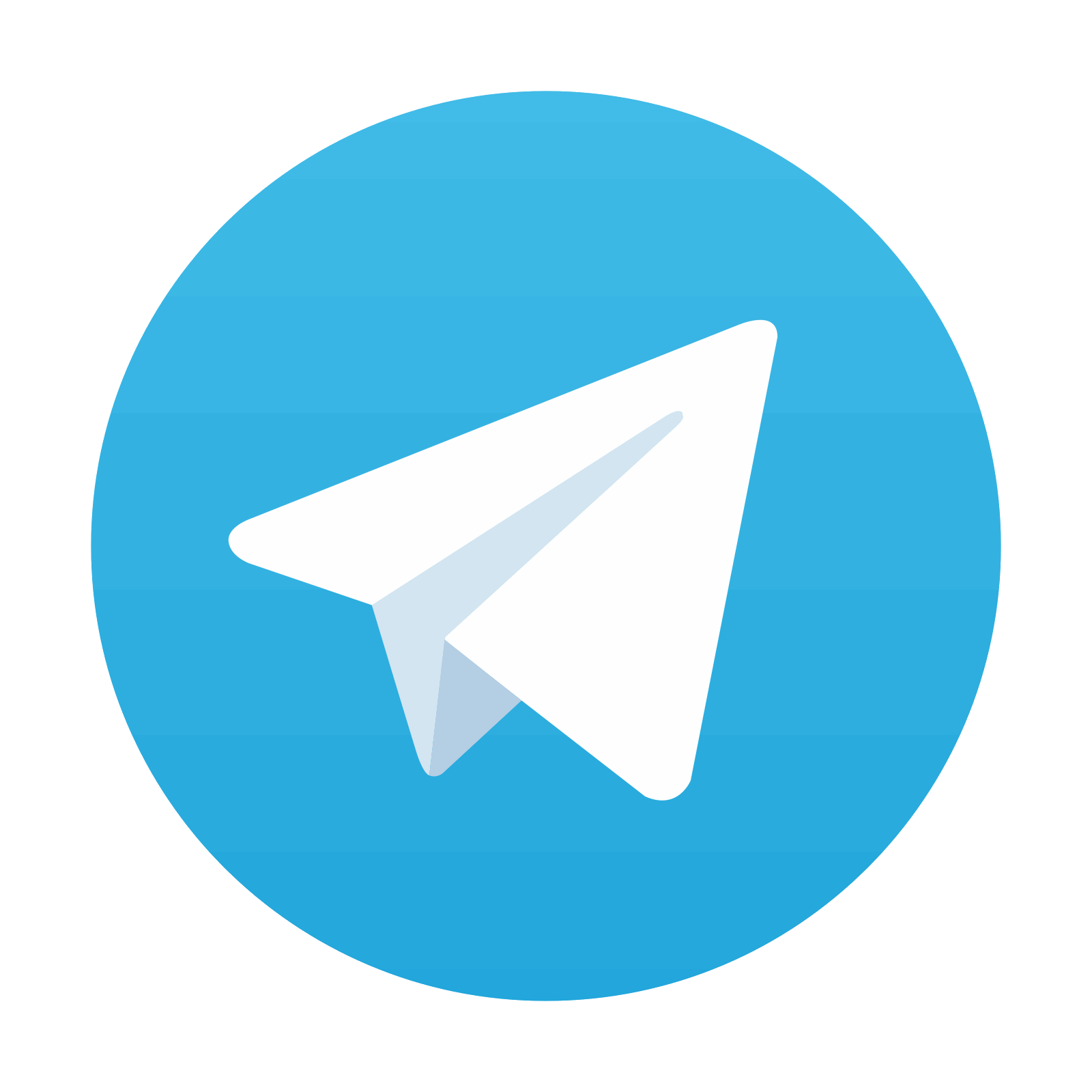
Stay updated, free articles. Join our Telegram channel

Full access? Get Clinical Tree
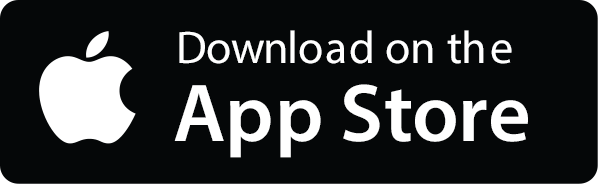
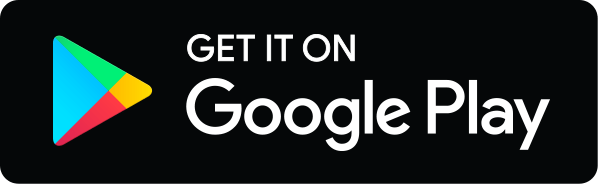
