The safety and efficacy of percutaneous coronary interventions (PCI) have been drastically improved by the introduction of bare metal stent (BMSs) in clinical practice.1 However, a new pathologic entity arose with the implantation of BMSs within coronary arteries: in-stent restenosis (ISR).2 ISR can be defined as the in-stent lumen narrowing that pathobiologically relates to the phenomenon of neointimal hyperplasia (NIH).3 Biologic, mechanical, procedural, and stent-related factors interplay in determining the incidence, morphology, and clinical implications of ISR. Introduction of drug-eluting stents (DESs) in 2001 in clinical practice represented a technologic breakthrough. DESs critically improved the efficacy of percutaneous revascularization procedures by reducing the need of target lesion revascularization (TLR) and target vessel revascularization (TVR) at follow-up.4 However, a low rate of ISR after DES implantation still exists. With more than 3 million DESs implanted worldwide each year, DES-ISR represents a major public health issue. In this chapter, we comprehensively review the incidence, mechanisms, diagnosis, and potential treatment strategies of ISR, with a particular focus in the contemporary DES era.
The goal of percutaneous revascularization procedures is the relief of significant coronary artery obstructions. Effectiveness of PCI with stent implantation is expressed in terms of clinically significant restenosis assessed objectively as a requirement for ischemia-driven repeat revascularization either of the stented lesion itself (TLR) or of the stented vessels and its collateral branches (TVR). ISR can be defined as a lumen narrowing within the site of stent implantation induced by local vascular barotrauma with reactive NIH. The clinical consequence of ISR is the development of a new flow-limiting obstructive lesion and subsequent reduction of downstream myocardial perfusion.
ISRs differ in their morphologic pattern, which in turn is associated with important clinical, prognostic, and therapeutic implications. The most accepted morphologic classification of ISR is the one proposed by Mehran et al.5 Before the introduction of this classification, patterns of ISR were broadly classified as focal (in-stent lesion <10 mm in length) or diffuse (in-stent lesion >10 mm in length). The Mehran classification introduced the description of the topographic relation between NIH and the coronary stent implanted. This classification is as follows (Fig. 33-1):
FIGURE 33-1
Descriptive diagram of in-stent restenosis (ISR) angiographic patterns. Pattern I (focal type) is <10 mm in length and contains 4 subtypes (types IA-ID). Patterns II to IV (diffuse type) are >10 mm in length and are distinguished according to the relation with the stent and the vessel lumen. (Reproduced with permission from Mehran R, Dangas G, Abizaid AS, et al. Angiographic patterns of in-stent restenosis: classification and implications for long-term outcome. Circulation. 1999;100:1872-1878.)

Class I, focal ISR group: Lesions are ≤10 mm in length and are placed at the unscaffolded segment (articulation or gap) (type IA), the body of the stent (type IB), the proximal or distal margin (type IC), or a combination of these sites (type ID; multifocal ISR).
Class II, diffuse ISR: Lesions are >10 mm in length and are confined to the stented segment, without extending outside the margin(s).
Class III, diffuse proliferative ISR: Lesions are >10 mm in length and extend beyond the margin(s) of the stented segment.
Class IV, ISR with total occlusion: Lesions with a Thrombolysis in Myocardial Infarction (TIMI) flow grade of 0.
In order to quantitatively evaluate the magnitude of ISR, 2 parameters have to be taken into account: (1) binary angiographic restenosis, defined as a luminal narrowing of 50% or more measured by follow-up coronary angiography6 and (2) late lumen loss (LLL), defined as the difference in millimeters between the diameter of a stented segment immediately after PCI and the diameter measured by a follow-up coronary angiography.6
Actually, the most widely used definition and classification of ISR is the one proposed by the Academic Research Consortium.6 This definition requires either a lumen narrowing of at least 50% of the vessel diameter associated with the evidence of functional significance (recurrent angina pectoris, objective signs of ischemia at rest or during exercise test [electrocardiographic (ECG) changes], abnormal fractional flow reserve [FFR <0.80] or intravascular ultrasound [IVUS]–assessed minimum cross-sectional area <4 mm2) or a luminal narrowing of at least 70% or greater in the absence of ischemic symptoms.
Morphologic classification of ISR is not exclusively descriptive; it also demonstrates a clinical prognostic value (Fig. 33-2). Diffuse intrastent, proliferative, and total occlusions represent a spectrum of increased ISR severity linked to an exaggerated NIH response. With BMS, a significant stepwise increase in TLR is associated with progressively greater levels of ISR in the Mehran classification (class I, 19%; class II, 35%; class III, 50%; class IV, 83%; P = .0001).
FIGURE 33-2
The morphologic pattern of in-stent restenosis (ISR) predicts the outcome. A stepwise increase in the need for revascularization is observed in the transition from focal patterns of ISR to occlusive patterns. 1°G, first generation; 2°G, second generation; TLR, target lesion revascularization. BMS, bare metal stent; DES, drug-eluting stent.

Also in DES, the morphologic pattern of ISR is a significant predictor of outcome.7,8 Cosgrave et al7 demonstrated in first-generation DES a significantly higher ISR rate after treatment (with repeat DES stenting or plain old balloon angioplasty [POBA]) in patients with nonfocal DES-ISR as compared to patients with focal DES-ISR (51.1% vs 17.8%; P = .001). In the same study, nonfocal pattern of ISR was a strong and independent predictor of TLR at follow-up, independently of the DES type.7 Latib et al8 observed in a large group of consecutive patients with DES-ISR treated with PCI (restenting or POBA) that recurrent ISR occurred in 29.1% of the focal type (class I), 45.8% (P = .007) of the diffuse type (class II and III), and 65.6% (P < .001) of the occlusive type (class IV) ISR. Accordingly, diffuse ISR was independently associated with a significant increase in TLR compared to focal ISR.8 In the same study, the investigators observed that the initial presentation pattern of ISR also predicted the pattern of recurrent ISR after the initial treatment. Occlusive ISR recurred as a reocclusion in 66.7%, diffuse ISR (class II and III) recurred as diffuse or occlusive in 57.9%, and focal ISR (class I) recurred as recurrent focal ISR in 67.2% of patients.
NIH is the pathobiologic substrate of ISR. Different types of mechanisms account for the temporal development, severity, and distribution of NIH and subsequent ISR (see Fig. 33-2; Fig. 33-3). Stent implantation with local arterial injury represents the first trigger of NIH with activation of complex local inflammatory pathways (see Fig. 33-3).9,10 Neointimal development consists in the activation, proliferation, and migration of smooth muscle cells within the intimal layer. These processes are followed by local extracellular matrix (ECM) deposition and remodeling with subsequent restenotic tissue accumulation within the stent. At least 4 types of factors can be identified in the pathogenesis of reactive NIH and subsequent ISR (Fig. 33-4): (1) biologic factors, (2) vascular factors, (3) procedural factors, and (4) stent factors.
FIGURE 33-4
Factors contributing to the development of in-stent restenosis (ISR). CAD, coronary artery disease; CTO, chronic total occlusion; DES, drug-eluting stent; IVUS, intravascular ultrasound; LAD, left anterior descending artery; PCI, percutaneous coronary intervention; SVG, saphenous vein graft.

Biologic factors for ISR include drug resistance, hypersensitivity, and genetic substrate. An accurate knowledge of the biologic factors underlying ISR might allow a customized coronary revascularization strategy. In fact, patients with an intrinsic high risk of developing ISR might benefit from additional local antiproliferative drug release, particular procedural tricks, or surgical revascularization.
Resistance to antiproliferative drugs shares the same molecular mechanisms observed in chemotherapy resistance in oncologic medicine.11,12 Drug resistance can be primary (in genetically predisposed individuals) or secondary, following exposure to the antiproliferative drug. The molecular pathways involved in drug resistance are complex, involving genes operating in drug absorption and metabolism, apoptosis regulation, DNA repair, and drug inactivation. Polymorphisms involving the genes encoding for mammalian target of rapamycin (mTOR) have been shown to be associated with paclitaxel or sirolimus resistance both in vitro and in vivo.13 Moreover, complex paracrine mechanisms involving cell survival signaling pathways and growth hormone response regulation influence the antiproliferative effect of the drug released by the stent platform.
Hypersensitivity reactions responsible for subsequent ISR may develop against 2 different targets: the DES polymer and the metallic stent platform.14-19 Implantation of a stent within a coronary artery induces a local barotrauma with endothelial denudation and subsequent activation of the inflammatory pathways with the distinctive foreign body reaction pattern with giant cell infiltration and granuloma formation. The inflammatory reaction occurring after arterial injury critically influences the burden of NIH. Persistence of local inflammation after 90 days is associated with a subsequent high risk of delayed endothelialization, ISR, and late or very late ST. First-generation DES polymers are strongly associated with an inflammatory response that persists beyond 180 days and for up to 2 years. This is in contrast to BMSs and second-generation DESs (which have a more biocompatible polymer), which are associated with an inflammatory reaction of 90 days and 12 months, respectively. Persistent inflammatory response represents a common pathways for the so-called “stent failure,” which include both ISR and ST.20 Hypersensitivity reactions to the metallic platform have also been described. Koster et al21 reported an association between nickel and molybdenum allergy and the risk of ISR with BMS. The evidence surrounding this issue is controversial and contradictory. With DES, no clear data exist. To date, a small study by Nakazawa et al22 found no association between the risk of ISR and the metallic platform allergy with sirolimus-eluting stents (SESs), suggesting that DESs prevent restenosis irrespective of metallic allergy.
The association between inflammatory and ECM remodeling biomarkers with ISR has been studied.23-25 The systemic inflammatory status assessed by means of C-reactive protein levels has not been consistently associated with ISR. Conversely, circulating matrix metalloproteinases (MMPs) have been shown to be useful in identifying patients at high risk of developing ISR. In particular, MMP-2 and MMP-9 elevations after PCI have been associated with the risk of ISR after DES implantation. MMPs are expressed and released by vascular smooth muscle cells (VSMCs), endothelial cells, and macrophages in response to local vessel trauma.
Arterial factors determining ISR include vessel size, lesion type, local shear stress, neoatherosclerosis, vessel remodeling, and edge vascular response.
Vessel size is a critical factor determining the effectiveness of PCI with both BMS and DES (Fig. 33-5). Agostoni et al26 demonstrated that BMSs are superior to POBA in a large meta-analysis of randomized controlled trials. Biondi-Zoccai et al,27 in another large meta-analysis of the use of DESs in small vessel disease, found that type of DES used has a substantial impact on PCI effectiveness. Pathologic mechanisms through which small vessel size influences PCI outcomes are small postprocedural minimal lumen area (MLA), higher degree of vessel injury and recoil, and higher metallic density.28 In particular, greater strut thickness has been associated with worse outcomes in small vessels.
FIGURE 33-5
Impact of vessel size on restenosis rates according to the use of balloon angioplasty, bare metal stents, or drug-eluting stents. PTCA, percutaneous transluminal coronary angioplasty. (Reproduced from Biondi-Zoccai G, Moretti C, Abbate A, Sheiban I. Percutaneous coronary intervention for small vessel coronary artery disease. Cardiovasc Revasc Med. 2010;11:189-198, Copyright © 2010, with permisison from Elsevier.)

Highly thrombotic lesions are associated with higher risk of stent failure with thrombosis or restenosis. High thrombus burden interferes with DES performance in several ways: (1) thrombus reduces the drug penetration and distribution by a magnitude of up to 10-fold29; (2) thrombus interferes with stent expansion, potentially leading to stent underexpansion; and (3) stent underexpansion and late thrombus resolution significantly increase the incidence of incomplete stent apposition and the risk of ST and restenosis. Despite this, a significant benefit with DESs, especially new-generation DESs, has been observed in ST-segment elevation myocardial infarction (STEMI) in several studies and meta-analyses.30 Although high thrombotic burden significantly increases the risk of stent failure and need for revascularization, the factors independently associated with TVR in STEMI do not seem to differ from those observed in other PCI settings.31 Finally, the use of thrombus aspiration does not seem to reduce the risk of thrombosis or restenosis after DES implantation.32
Blood hemodynamics in relation to vessel geometry and stent strut design play a role in determining endothelial dysfunction, vessel remodeling, and restenosis (Fig. 33-6). Within the arterial vessel, the fluid velocity is maximal in the middle of the lumen and minimal in proximity to the vessel wall due to viscosity forces. Particular lesion subsets, such as bifurcations or ostial lesions, are associated with a significantly lower fluid velocity and endothelial shear stress (ESS).33,34 Reduced blood flow combined with local vessel injury may contribute to accumulation of growth factors, cytokines, and blood cells promoting NIH development and subsequent restenosis. The relationship between everolimus-eluting stents (EESs) and ISR has been demonstrated in several studies. Wentzel et al35 showed an inverse relationship between EES magnitude and the extent of ISR with BMS, which has been demonstrated by later investigations. Importantly, although ISR did not develop in sites with low ESS, not all sites of ISR originated from low ESS regions, showing the multifactorial contribution to ISR development. The relationship between low ESS regions and ISR was also demonstrated with SESs and paclitaxel-eluting stents (PESs). Apart from vessel anatomy, stent design critically influences ESS and ISR risk (see later Stent Factors section).
FIGURE 33-6
Effect of nonstreamlined (A) and streamlined (B) strut design on endothelial shear stress and risk of thrombosis and restenosis. EES, everolimus-eluting stent; ESS, endothelial shear stress. (Adapted from Koskinas KC, Chatzizisis YS, Antoniadis AP, Giannoglou GD. Role of endothelial shear stress in stent restenosis and thrombosis: pathophysiologic mechanisms and implications for clinical translation. J Am Coll Cardiol. 2012;59:1337-1349.)

Neoatherosclerosis (NA) emerged recently as a potential cause of late DES failure responsible for both restenosis and thrombosis of the stent platform.36 NA can be defined as the presence of an atherosclerotic lesion within the neointima of a stented vascular segment. NA can assume a pathologic feature ranging from simple intimal thickening with lipid infiltration to ruptured thin-cap fibroatheroma with thrombosis and acute clinical presentation. Pooling the evidences from histopathologic and endovascular imaging studies, NA seems to occur with both BMS and DES in which it can be responsible for lumen occlusion with restenosis and subsequent need for revascularization and plaque rupture with thrombotic occlusion of the stent. NA is carefully reviewed and discussed in the Histopathology of In-Stent Restenosis and Imaging of In-Stent Restenosis sections.
Vascular remodeling influences NIH formation.37-39 Large plaque burden is associated with positive arterial remodeling (PR). Okura et al38 demonstrated that the presence of PR (defined as vessel area at the target lesion greater than that of average reference segments) is associated with adverse outcomes after IVUS-guided stent implantation. Following multivariable adjustment, remodeling index (defined as vessel area at the target lesion site divided by that of average reference segments) was the only independent predictor of TVR at 9 months. The association between PR and ISR may be explained by several mechanisms. First, PR is more frequent in unstable lesions; therefore, lesions with PR may be more biologically active and more prone to an exaggerated NIH response. Second, several studies demonstrated that the relative plaque burden outside the stent is proportional to the NIH that develops within the stent.
Edge vascular response (EVR) is defined as the pathologic changes occurring in the vascular tissue adjacent to the segment of stent implantation with subsequent focal lumen narrowing and need for revascularization of the target lesion.40 EVR can occur in the presence or absence of longitudinal geographic miss (LGM) with uncovered plaque, and thus, it is only partially dependent on the stent landing zone. The pathologic factors related to EVR include procedural factors (axial geographic miss or LGM, vascular injury, and plaque shift), stent factors (stent platform and eluted drug), and lesion factors (plaque burden). The TAXUS-II, -IV, -V, and -VI trials reported similar rates of LLL at both proximal and distal stent edges with BMS and PES. Conversely, the SIRIUS trial reported that ISR with SES had more commonly a focal pattern located at the proximal DES edge. In the ENDEAVOR-IV trial, the zotarolimus-eluting stent (ZES) was associated with comparable rates of LLL at both stent edges. Conversely, with bioresorbable vascular scaffolds (BVSs) in the ABSORB Cohort B1 study, LLL was significantly higher at the proximal edge. Compared to BMS, DES seems to be associated with lower distal LLL, most likely because of downstream drug effects related to drug release by the DES platform.
Optimal stent implantation is of paramount importance in reducing the risk of both restenosis and ST after PCI, as are directly modifiable factors. Procedural factors influencing the risk for ISR include stent underexpansion, LGM and axial geographic miss, unstented segment barotrauma with EVR, stent gap, and off-label DES implantation.
Stent underexpansion and smaller postprocedural minimal lumen diameter (MLD) are strongly associated with long-term DES patency and risk of ISR and ST.41-46 IVUS use significantly improved stent deployment and achievement of an optimal postprocedural MLD. IVUS analyses from the SIRIUS trial and other studies identified an optimal cutoff of postprocedure minimum stent area to reduce the risk of restenosis of 5.0 to 5.5 mm2. In a large meta-analysis comparing IVUS-guided with angiographic-guided BMS implantation, Casella et al47 demonstrated that IVUS use significantly reduces the risk of TVR, binary restenosis, and major adverse cardiac events (MACE) at 6 months of follow-up. Recently, a substudy from the ADAPT-DES (Assessment of Dual Antiplatelet Therapy With Drug-Eluting Stents) trial, the largest observational study of IVUS to date, reported a significant reduction in 1-year rates of ST, myocardial infarction (MI), MACE, and repeated revascularization with IVUS use.48 Moreover, a large meta-analysis including 26,503 patients demonstrated a significant benefit with IVUS use during PCI with DES implantation.49 Conversely, results from randomized clinical trials in the DES era are controversial.50,51 Some of them showed that the benefits of IVUS are observed in complex lesions, while others suggested that IVUS use per operator decision was associated with improved results (in the per-protocol analysis of the RESET [Real Safety and Efficacy of a 3-Month Dual Antiplatelet Therapy Following Zotarolimus-Eluting Stent Implantation] IVUS trial).52 Until large-scale trials demonstrate a clear advantage of IVUS-guided procedures, its use will be at the discretion of the clinical and anatomic evaluation of the operator.
LGM (Fig. 33-7) is defined as the failure to cover the target lesion with intracoronary stent implantation.53 Failure to cover the lesion with the implanted stent is associated with a significant portion of the lesion remaining out of the stent margins and barotrauma of nondiseased vessel segments. Conversely, axial geographic miss (AGM; see Fig. 33-7) is defined as a final balloon-artery size ratio mismatch <0.9 or >1.3.53 In the STLLR study, LGM was observed in two-thirds of the study cohort, with half of the patients having LGM and more than one-third having AGM.53 Approximately 15% had both LGM and AGM. Patients with geographic miss had higher rates of TVR and MI at 1 year of follow-up. Among patients with geographic miss, most of the cases were observed in patients with LGM. Moreover, an additional subanalysis from the STLLR study showed that diabetic patients with LGM had significantly higher rates of TLR compared with nondiabetics, underscoring the importance of optimal stent implantation in high-risk patient subsets such as those with diabetes.
Stent gap can be defined as a discontinuous coverage between 2 DESs throughout the coronary vessel extension. A gap between 2 DESs leaves a small zone of the coronary vessel not exposed to the antiproliferative effects of the released drug and the mechanical support of the metallic strut. Considering that overlapping DESs have been demonstrated to be safe and effective, a gap between 2 DESs should be avoided.54
Finally, off-label DES use has been consistently associated with worse clinical outcomes after PCI. The STENT (Strategic Transcatheter Evaluation of New Therapies) Group is the largest prospective registry evaluating outcomes in patients undergoing DES implantation in the United States. Brodie et al,55 in an analysis from this registry, compared on-label and off-label (ostial, left main, chronic total occlusion, saphenous vein graft, small vessels, multivessel disease, and ISR lesions) indications for DES implantation and reported a significantly higher TVR rate in the off-label group at 2 years (11.8% vs 6.5%; P < .01).
With DES, stent-related factors influencing ISR include strut thickness; drug release kinetics; drug type; stent fracture; polymer disruption, peeling, and cracking; and stent design.
The type of intracoronary stent influences the burden and temporal evolution of NIH.56 Angiographic and IVUS studies (Fig. 33-8) demonstrated a different pattern of NIH development with BMS and DES over time. IVUS studies with permanent polymer DES documented an increase in neointimal volume beyond the time point in which NIH is interrupted with BMS.57-59 Although with BMS the burden of NIH is significantly higher after the procedure and at long-term follow-up, it tends to reduce over time. Conversely, with early-generation DESs, neointimal volume tends to slowly increase years after the implantation. Finally, with bioresorbable polymer DESs, a stable behavior in neointimal volume has been observed. These differences might reflect the variable vascular response and inflammatory reaction to the DES polymer, which involves chronic inflammation associated with very-late restenosis in DES.
FIGURE 33-8
Differences in neointimal volume at follow-up between bare metal stents (BMSs), first-generation drug-eluting stents, and new-generation drug-eluting stents (DESs). BES, biolimus-eluting stent; EES, everolimus-eluting stent; IVUS, intravascular ultrasound; NIH, neointimal hyperplasia; MR, moderate release; PES, paclitaxel-eluting stent; SES, sirolimus-eluting stent; SR, slow release.

The fine balance between the drug type, drug release kinetics, and dosage is a critical factor in DES design. Drug release kinetics critically influence the suppression of NIH even more than the total dose of drug released. To ensure an adequate suppression of NIH, a certain dose of drug has to be delivered to the vessel wall during a sufficient time period, during which strut endothelization is ongoing. The in-human PISCES trial tested 6 different polymer-drug release formulations and observed that a greater magnitude in the inhibition of neointimal formation was achieved with long-term drug-release formulations than with drug-polymer combinations with shorter eluting times.60 Similar results have also been observed by Waseda et al61 comparing different polymer formulations with ZES.
Although drug release kinetics is of critical importance in suppressing NIH, the type of drug also plays an important role. The antiproliferative drugs associated with the greatest effects are paclitaxel and limus family drugs.62,63 These drugs differ in their mechanism of action and intracellular pharmacodynamics. Pclitaxel acts by stabilizing the microtubule polymer and blocking the progression of mitosis, triggering apoptosis or reverting to the G phase of the cell cycle without reaching the cell division phase. Sirolimus, everolimus, biolimus A9, and zotarolimus all bind to the FKBP12 protein, which subsequently binds and inhibits mTOR, blocking the transition from the G1 to the S phase. Conversely, tacrolimus and pimecrolimus act by binding FKBP506, which subsequently inhibits the calcineurin receptor, leading to a reduced intracellular growth factor signaling pathway. In the PAINT trial, Lemos et al64 compared 2 DESs with the same metallic platform and polymer but different released drugs (paclitaxel or sirolimus) and a BMS with the same metallic stent. At 9 months, the DES eluting sirolimus was associated with a significantly lower late loss compared with the DES eluting paclitaxel and the BMS.
Stent fracture (SF) can be defined as a complete or partical discontinuation in the strut structure at follow-up.65-68 SF is associated with 2 consequences: (1) loss of the local vascular support provided by the metallic strut, and (2) severe impairment of the local drug delivery. The most common consequence of SF is focal restenosis due to the decreased local drug delivery and impairment in NIH suppression. At IVUS assessment, partial SF is defined as the absence of at least one-third or 120° of stent struts for at least 1 frame; conversely, complete SF is defined as the total absence of stent struts for at least 1 frame. The most common risk factors for SF are right coronary artery lesions, vessel tortuosity, lesion length, moderate or severe calcifications, longer stents, SES use (due to the closed-cell design, as opposed to an open-cell design), myocardial bridges, and overlapping stents. The incidence of SF ranges from 1% to 8% and is associated with a significant increase in need for revascularization at follow-up. In particular, the LONG-DES-II study, which evaluated incidence and predictors of DES fracture in long coronary lesions, reported an incidence of SF of 1.7% and a subsequent restenosis rate at 6-month follow-up of 14%.69 Finally, stent cell design critically influences the risk of SF. In fact, in a recent meta-analysis, the incidence of SF was of 0.1% with PES (open-cell design) and approximately 2.3% with SES (closed-cell design).70
Polymer disruption has been demonstrated in bench studies with both old- and new-generation DES.71,72 Disruption and spreading of polymer components may be associated with reduced effectiveness of the drug release and increased local inflammation. Stent implantation failure was demonstrated to be associated with significant polymer damage. Bifurcation lesions have been associated with a high risk of polymer damage, nonuniform drug release, and focal restenosis. Guerin et al,73 in a bench study using scanning electron microscopy to evaluate the polymer integrity of 5 different types of DES, observed that a kissing balloon was associated with a significantly greater coating damage to the ostial portion of all the stents tested. In particular, the Endeavor stent (Medtronic, Dublin, Ireland) showed a subtotal disruption of the polymer on the luminal face, whereas the other DES showed a more localized pattern of polymer rupture.
The magnitude of stent-induced flow changes and vascular response is substantially dependent on strut design (see Fig. 33-6).34 Strut shape and thickness and the strut-strut intersection arrangement are all associated with ISR. Across stent types, stents with hemodynamically favorable designs are associated with homogeneous ESS across the inner platform surface and lower risk of ISR in clinical practice. Heterogeneous ESS is the result of flow disruption generating zones of high ESS and zones of low ESS (see Fig. 33-6A). A homogeneous ESS can be achieved by reducing strut thickness and a streamlined strut shape (see Fig. 33-6B). Several studies demonstrated that a nonstreamlined strut drastically increases low ESS to more than 80%. Strut connector length also influences in-stent hemodynamics. A computational comparison of 5 stents used in clinical practice demonstrated that minimal connector length in the cross-flow direction decreased the percentage of in-stent area with low ESS.
Underlying ISR histopathology relates to the angiographic appearance and clinical severity of ISR. ISR with BMS has been extensively studied in postmortem9,74 and endarterectomy75,76 specimens. Although the pathologic substrate of vessel restenosis with POBA was represented essentially by chronic vessel recoil with negative remodeling, VSMC proliferation is the dominant pathologic process leading to restenosis after BMS and DES implantation. The main identifiable cellular components of NIH are α-smooth muscle actin–positive cells embedded in a collagen-rich extracellular matrix (ECM). The composition of the hyperplastic restenotic tissue evolves over time. NIH evolution can be categorized into an early stage, characterized by high cellular density in active proliferation within a proteoglycan-rich ECM, and a late stage, characterized by a high burden of mature and dense ECM with lower cellular density and proliferation rate.76 Chung et al76 described the ECM changes in ISR tissue over time. Late ISR was composed mainly of large amounts of proteoglycan-rich and collagen-rich ECM alongside a low number of proliferative VSMCs. Interestingly, the investigators observed a significant correlation between ECM composition and ISR severity, instead of cellular proliferation.
Histopathology of ISR varies between BMS and DES. In an interesting study, van Beusekom et al77 compared the pathologic features of atherectomy specimens obtained from restenotic tissue in BMS, DES, and de novo atherosclerotic lesions. ECM in de novo atherosclerotic lesions was more commonly collagen-rich, whereas BMS- and DES-ISR tissue was more commonly proteoglycan-rich. With both BMS- and DES-ISR, the cellular component was mainly composed by proliferated VSMCs. However, DES-ISR tissue was richer in fibrinoid tissue, indicating a more persistent incomplete vascular healing response. Quantitatively, DES-ISR was significantly lower in terms of mass and volume compared with BMS-ISR. This difference is most likely related to the more prevalent focal nature of ISR with DES compared with BMS (see later section In-Stent Restenosis With Bare Metal Stents and Drug-Eluting Stents).
Chieffo et al78 compared the histopathology of ISR with BMS and a wide range of DES by means of light microscopy specimens obtained through directional coronary atherectomy. In both BMS- and DES-ISR, specimens were composed mainly of proteoglycan-rich smooth muscle cells and a fibrolipidic stroma. The authors found significant differences in VSMC phenotype across stent types. In particular, restenotic tissue was mainly composed of intermediate-synthetic VSMC phenotype in BMS and PES, of intermediate VSMC phenotype in ZES, of contractile-intermediate VSMC phenotype in SES, and of mostly contractile VSMC phenotype in tacrolimus-eluting stents. These differences in VSMC phenotype might reflect the differential responses to stent types and, in the case of DES, to the antiproliferative drug. Both BMSs and PESs were associated with an intermediate-synthetic phenotype, potentially partially explaining the high NIH burden observed with these stents.
Local vascular inflammation induced by vessel barotrauma following intracoronary stent implantation is a critical factor influencing the burden of subsequent neointimal hyperplastic response. Moreover, persistence of an inflammatory milieu is related to greater NIH thickening, remodeling, and subsequent ISR. Farb et al9 demonstrated that early after stent implantation, features of acute inflammation at the interface of the stent and arterial wall were almost always present. Moreover, the authors underscored the importance of vascular medial layer damage. In fact, NIH thickness was significantly greater when medial damage (arterial medial laceration or rupture) occurred.9 Wilson et al79 further clarified the role of chronic inflammation associated with first-generation DES implantation. Histopathologic analysis of stented porcine coronary arteries showed features of chronic inflammation with giant cells, granulomas, and eosinophilic infiltrations at long-term follow-up (between 90 and 180 days), whereas no evidence of inflammation was present with BMS. The main trigger for arterial chronic inflammation is represented by the presence of the durable polymer. Durable polymers induce and sustain chronic inflammation over time, which in turn is responsible for both delayed endothelialization and greater burden of NIH. Therefore, bioresorbable polymer DES design could be an attractive option in reducing the polymer-related inflammatory response and subsequent risk of thrombosis and restenosis. Bioresorbable polymer DESs seem to be associated with improved safety and efficacy profiles compared with first-generation DESs.80
Another potential mechanism influencing the features of ISR with BMS and DES is the delayed endothelialization phenomenon. From an autopsy registry, Joner et al81 compared the vascular histopathology of DES and BMS, observing that after 30 days DESs were associated with significantly delayed vascular healing manifested by incomplete endothelialization and significant fibrin persistence and accumulation. These pathologic differences may account for both DES failure events—ST and ISR. The higher prevalence of thrombotic components within DES-ISR led to the theory of the “thromborestenosis phenomenon.” Oikawa et al82 demonstrated, by means of IVUS, angioscopy, and histopathologic analyses, that thrombus and fibrin deposition are substantially more frequent in ISR lesions of SES compared with BMS. Therefore, chronic thrombus formation may play a role in the pathogenesis of ISR in DES, especially when delayed endothelialization occurs.
Finally, in-stent NA emerged as a potential mechanism of stent failure, being associated with both ISR and ST, and may account for the “late catch-up phenomenon” observed in DES trials at long-term follow-up.83,84 NA is defined as the presence within the neointima of atherosclerotic plaque elements like foamy macrophages in clusters with or without calcification, fibroatheromas, and thin-cap fibroatheromas. The pathobiology of NA can be described as a form of accelerated atherosclerosis secondary to delayed reendothelialization and severe endothelial dysfunction. Nakazawa et al36 described the incidence of NA in BMS, SES, and PES postmortem pathologic specimens (Fig. 33-9). Compared with BMS, the incidence of NA was significantly higher with DES (31% vs 16%; P < .01), whereas no differences were present between the 2 types of first-generation DESs. Importantly, NA occurred earlier with DES compared with BMS. In fact, the median stent duration with NA was shorter in DES than BMS (DES: 420 days; BMS: 2160 days; P < .001). Interestingly, SES was associated with a greater NA change compared with PES for implant durations of 2 years or less (SES 37% vs PES 21%; P = .021). The earlier development of NA in DES may be due to delayed stent endothelialization with reduced cell-to-cell contact and increased lipidic and macrophage intimal infiltration. NA and its role and evaluation in ISR will be further discussed in the Imaging of In-Stent Restenosis section.
FIGURE 33-9
A to C. Histologic section from a paclitaxel-eluting stent (PES) implanted in a left circumflex artery 14 months antemortem: (A) patent lumen with presence of moderate neointimal growth; (B) foamy macrophage infiltration plus cholesterol clefts within the neointima; and (C) presence of CD68-positive macrophages in the neointima surrounding the strut (asterisk). D to F. Pathologic specimens from a sirolimus-eluting stent (SES) with superimposed thrombosis: (E) evidence of thin-cap fibroatheroma with cap rupture and (F) presence of CD68-positive macrophages in the fibrous cap and in the necrotic core. G to I. Pathologic specimens from a bare metal stent (BMS) implanted 8 years before death with superimposed stent thrombosis: (G) presence of occlusive thrombus within the stent lumen and (H and I) presence of ruptured plaque with a large number of macrophages at the site of cap disruption. (Reproduced from Nakazawa G, Otsuka F, Nakano M, et al. The pathology of neoatherosclerosis in human coronary implants bare-metal and drug-eluting stents. J Am Coll Cardiol. 2011;57:1314-1322, Copyright © 2011, with permission from Elsevier.)

Predictors of ISR with BMS and DES are illustrated in Table 33-1. Among other factors, diabetes mellitus (DM) has been consistently identified as a strong clinical predictor of ISR with both BMS and DES.85 Moreover, DM patients present more frequently with a worse morphologic pattern with a prevalent proliferative (type III) and occlusive ISR (type IV).5,86 Kornowski et al87 observed that ISR in DM patients, compared with ISR in non-DM patients, is associated with higher burden of NIH, underlying the influences of impaired glucose homeostasis and insulin resistance in promoting cellular proliferation after vascular injury. The accentuated neointimal hyperplastic response in diabetics has been documented in several studies. An IVUS-ISR study in patients treated with BMS showed substantial differences in the degree of neointimal hyperplasia among diabetics (5.1 ± 2.8 mm2) compared to nondiabetics (2.1 ± 2.0 mm2).87 Moreover higher prevalences of heterogeneous patterns have been observed among diabetics.88
Predictors with BMS | |
Clinical | Diabetes mellitus Female sex |
Anatomic | Long lesions Small vessels Baseline MLD Plaque burden Complex lesions (B2/C) Multivessel disease ISR lesions Plaque burden |
Procedural | Stent length Postprocedural MLD Stent length/lesion length ratio Coil stents |
Predictors with DES | |
Clinical | Diabetes mellitus Female sex |
Anatomic | Long lesions Small vessels Baseline MLD Plaque burden Complex lesions (B2/C) Multivessel disease ISR lesions Saphenous vein grafts |
Procedural | Postprocedural MLD Stent gap; stent fracture Use of PES or E-ZES Stent length |
Several factors are associated with specific morphologic patterns of ISR. Goldberg et al89 identified predictors of diffuse (≥10 mm) ISR and aggressive ISR (defined as the development of a longer or tighter lesion at follow-up compared with baseline) with BMS. Predictors of diffuse ISR were longer baseline lesion length, smaller final result achieved, and the use of coil stents. Conversely, predictors of aggressive ISR were female sex, MLD at baseline, lesion length, and stent length/lesion length ratio. Among these, MLD at baseline was the strongest predictor. The role of MLD as a predictor of TVR for ISR was confirmed in the CRUISE (Can Routine Ultrasound Influence Stent Expansion) trial.90 In this study, final in-stent MLD was also demonstrated to be a significant predictor of TVR.
Lesion-related characteristics impact the risk of ISR and specific ISR morphology. Elezi et al91 demonstrated in a study of 2602 patients the important influence of vessel size on ISR rate. In this study, patients with small-size vessels (<2.8 mm) had a significantly higher restenosis rate compared with patients with mid-size (2.8-3.2 mm) and large-size (>3.2 mm) vessels (38.6% vs 28.4% vs 20.4%, respectively; P < .001). The association persisted following multivariable adjustment. Within small vessels, a subsequent study performed by Hausleiter et al92 demonstrated that the main predictors in this lesion subset are diabetes, complex lesions (type B2/C), vessel stenosis diameter before intervention, stented segment length, balloon/vessel ratio, and stent design.
Finally, Hoffmann et al93 explored angiographic and IVUS predictors of ISR demonstrating 3 imaging variables consistently associated with ISR: ostial lesion location (odds ratio [OR], 4.01; P = .015), lesion site plaque burden (plaque/total arterial area) assessed by IVUS (OR, 1.25; P = .008), and final lumen area or MLD assessed by IVUS (OR, 0.24; P = .013). These predictors underscore the importance of IVUS-guided procedures to optimize PCI outcomes.
Predictors of ISR with DES are similar to those with BMS (see Table 33-1),94 in which clinical, procedural, and anatomic factors have been consistently identified as independent predictors of restenosis and revascularization. However, with DES, procedural factors seem to have relatively more impact on the risk of restenosis compared with BMS. Kastrati et al,95 who analyzed predictors of ISR with SES and PES in a cohort of 1845 and 2093 target lesions, respectively, found that baseline clinical characteristics were not as strong predictors of ISR compared with angiographic factors such as vessel size, complex lesions, and final angiographic results. Moreover, differences in ISR rate among DESs might be more evident in small coronary arteries.96 Other potential risk factors for restenosis with SES were evaluated by Lemos et al97 in the RESEARCH (Rapamycin-Eluting Stent Evaluated at Rotterdam Cardiology Hospital) trial. The investigators identified treatment of ISR, DM, and total stented length as independent predictors of ISR ostial lesions.
Compared with BMS, DESs are more commonly associated with a focal pattern of ISR. Investigators studied the predictors of nonfocal ISR in DES. The investigators identified female sex, overlapping stent implantation, and PES use as independent predictors of nonfocal ISR.7,98 Lesion complexity is strongly associated with ISR with DES. In particular, PCI of chronic total occlusive lesions also carries a high risk of restenosis after successful stent implantation. In a prospective cohort of successfully revascularized chronic total occlusive lesions using DES, Lee et al99 reported an ISR incidence of 24% at 6 to 9 months, with a prevalence of diffuse ISR of 61%. Finally, in the new-generation DES era, the RESOLUTE-All Comers randomized trial reported insulin-treated diabetes, treatment of saphenous vein graphs, ostial lesions, ISR treatment, and right coronary artery as a target vessel as independent major predictors of TLR.100
DES implantation appears to be effective in attenuating the burden of NIH compared with BMS. However, DM is still a strong independent predictor of TLR even in the DES era.56 Moreover, several reports have shown that the presence of DM may attenuate the benefits associated with newer versus first-generation DESs.101,102
Stent type critically influences the risk of ISR, its morphologic pattern, and its associated clinical outcomes. Introduction of DESs drastically reduced the incidence of ISR and subsequent need of TLR.4 However, even in the DES era, the risk of ISR still persists. SES and EES were demonstrated to be the 2 types of DES associated with the lowest incidence of TVR. However, over time, EES demonstrated a significant improvement in the safety profile compared with first-generation DESs.103 A summary of the incidence of ISR and relative prevalence of morphologic patterns with different types of DESs from randomized controlled trials is reported in Table 33-2, Figure 33-10, and Figure 33-11.
No. of DES-Treated Patients | Follow-Up Period | Binary ISR | In-Segment Restenosis | Longest Clinical Follow-Up (mo) | TLR at Longest Clinical Follow-Up | |
---|---|---|---|---|---|---|
SES | ||||||
RAVEL195 | 120 | 6 months | 0% | 0% | 60 | 10.3% |
SIRIUS196 | 533 | 8 months | 3.2% | 8.9% | 60 | 9.4% |
E-SIRIUS197 | 175 | 8 months | 3.9% | 5.9% | – | – |
C-SIRIUS198 | 50 | 8 months | 0% | 2.3% | – | – |
SES SMART199 | 129 | 8 months | 4.9% | 9.8% | 24 | 7.9% |
DIABETES200 | 80 | 9 months | 3.9% | 7.8% | 48 | 8.1% |
PES | ||||||
TAXUS II SR201 | 131 | 6 months | 2.3% | 5.5% | 60 | 10.3% |
TAXUS II MR201 | 135 | 6 months | 4.7% | 8.6% | 60 | 4.5% |
TAXUS IV202 | 662 | 9 months | 5.5% | 7.9% | 60 | 9.1% |
TAXUS V203 | 577 | 9 months | 13.7% | 18.9% | 60 | 17.0% |
TAXUS V ISR204 | 195 | 9 months | 7.0% | 14.5% | 24 | 10.1% |
TAXUS VI205 | 219 | 9 months | 9.1% | 12.4% | 60 | 14.6% |
ZES | ||||||
ENDEAVOR II206 | 598 | 8 months | 9.4% | 13.2% | 60 | 7.5% |
SES versus PES | ||||||
REALITY207 SES | 648 | 8 months | 7.0% | 9.6% | – | – |
PES | 669 | 8 months | 8.3% | 11.1% | – | – |
SIRTAX208 SES | 503 | 8 months | 3.2% | 6.6% | 14.9% | |
PES | 569 | 8 months | 7.5% | 11.7% | 60 | 17.9% |
ISAR-DIABETIC209 SES | 180 | 6-8 months | 8.0% | 11.4% | – | – |
PES | 180 | 6-8 months | 14.9% | 19.0% | – | – |
ISAR-SMART 3210 SES | 100 | 6-8 months | 11.0% | 14.3% | – | – |
PES | 100 | 6-8 months | 18.5% | 21.7% | – | – |
ISAR-DESIRE211 SES | 125 | 6-8 months | 4.9% | 6.9% | – | – |
PES | 125 | 6-8 months | 13.6% | 16.5% | – | – |
ZES versus SES | ||||||
ENDEAVOR III212 ZES | 323 | 8 months | 9.2% | 11.7% | 60 | 8.1% |
SES | 113 | 8 months | 2.1 | 4.3% | 6.5% | |
ZES versus PES | ||||||
ENDEAVOR IV213 ZES | 770 | 8 months | 13.3% | 15.3% | 36 | 6.5% |
PES | 772 | 8 months | 6.7% | 10.4% | 6.0% | |
EES versus PES | ||||||
SPIRIT II214 EES | 223 | 6 months | 1.3% | 3.4% | 48 | 5.9% |
PES | 77 | 6 months | 3.5% | 5.8% | 12.7% | |
EES versus PES | ||||||
SPIRIT III215 EES | 669 | 8 months | 2.3% | 4.7% | 36 | 5.4% |
PES | 332 | 8 months | 5.7% | 8.9% | 8.9% | |
BES versus SES | ||||||
LEADERS145 BES | 857 | 9 months | 17.5% | 16.4% | 48 | 10% |
SES | 850 | 9 months | 19.6% | 18.5% | 13% |
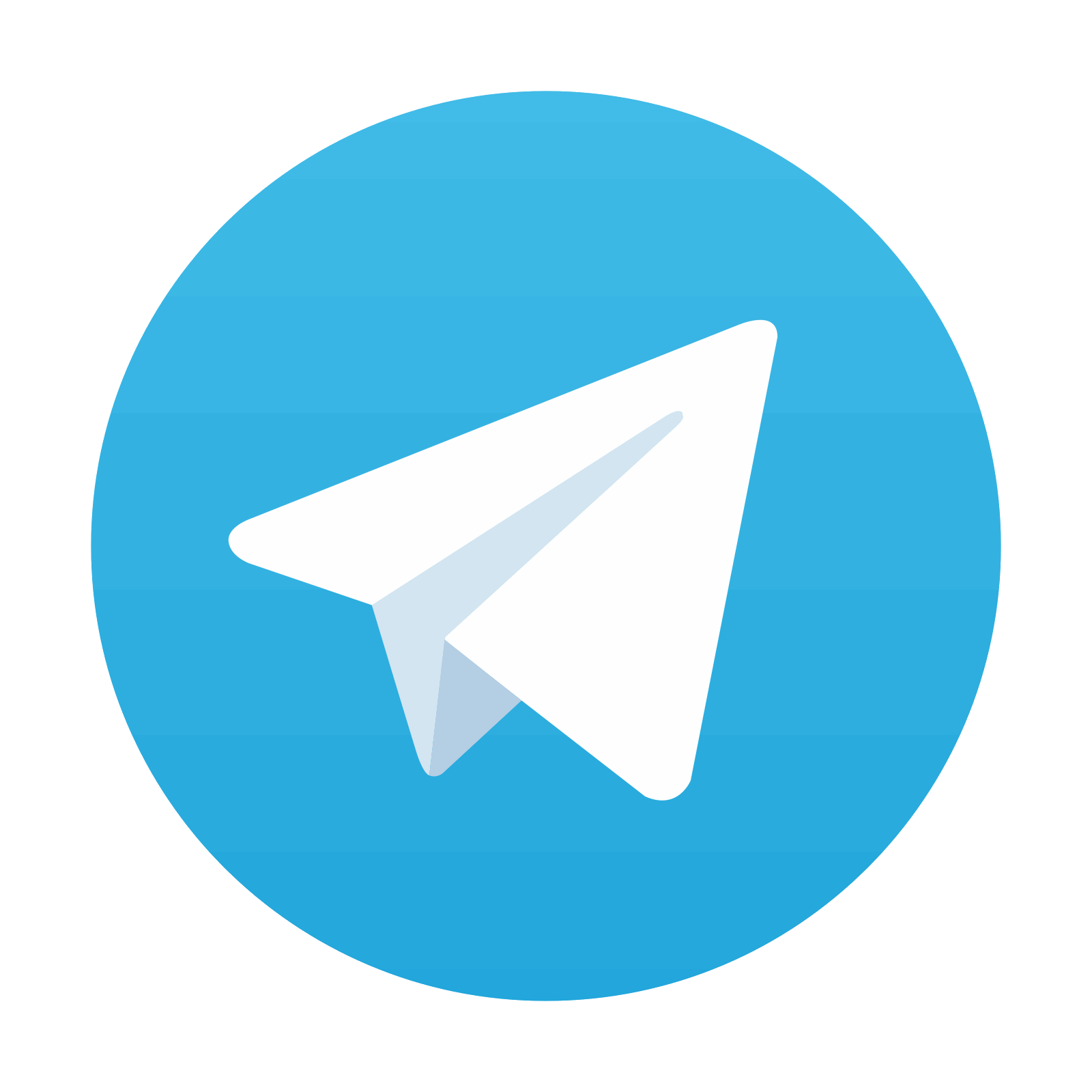
Stay updated, free articles. Join our Telegram channel

Full access? Get Clinical Tree
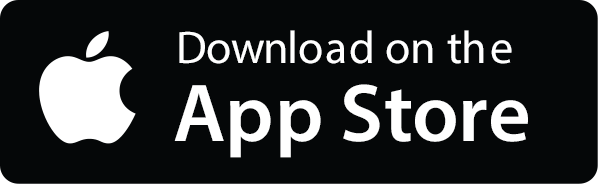
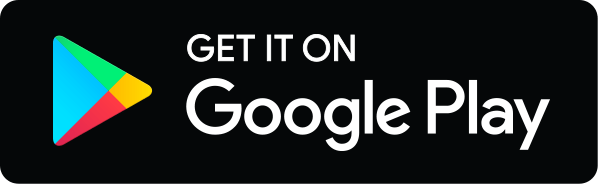
