In-Stent Restenosis
Wells J. Askew
John P. Sweeney
The advent of coronary stents over a decade ago transformed the practice of interventional cardiology by increasing procedural success (1), decreasing procedural complications, and improving long-term outcomes (2). The early trials of coronary stenting in de novo coronary lesions, Stent Restenosis Study (STRESS) and Belgium Netherlands Stent (BENESTENT) studies, definitively demonstrated that stents reduced restenosis rates in discrete de novo lesions of large caliber vessels (1,3). With technical improvements in the stent implantation procedure, the restenosis rate initially declined in selected patient populations (4). As stent design and deliverability improved and operator experience increased, more complex lesions were treated, dramatically increasing stent usage in clinical practice. However, registries of “real world” practice, which have included these complex lesions, have reported substantially higher restenosis rates when compared with earlier, more selected populations (5,6). In addition, once in-stent restenosis occurs, treatment can be a challenge, because standard methods of revascularization have resulted in suboptimal outcomes. With more than 80% of coronary interventions now resulting in stent implantation, the challenge of in-stent restenosis promises to be a major clinical issue for years to come.
Patterns, pathogenesis, mechanisms, predictors, treatment, and prevention of in-stent restenosis will be addressed in this chapter.
INCIDENCE
The incidence of in-stent restenosis in the current era of drug-eluting stents (DES) is unknown. Prior to the advent of DES, Di Mario et al. estimated that more than 150,000 patients would require treatment for in-stent restenosis annually (7). However, recent trials of sirolimus- and paclitaxel-eluting stents have demonstrated a substantial reduction in target-vessel failure (TVF) and restenosis rates in selected patient populations (8,9). In addition, sirolimuseluting stents have demonstrated low rates of restenosis in “real world” populations of unselected patients with complex lesions, including long lesions in small vessels (10, 11, 12). Based on this data, one can safely assume that there will be a substantial reduction in the number of in-stent restenosis cases; however, the magnitude of this reduction is yet to be determined.
DEFINITION
Restenosis that results from “the arterial healing response after injury incurred during revascularization” (13) has been defined both angiographically and clinically. Numerous descriptions of the restenotic process using continuous outcomes (i.e., cumulative frequency curves) or comparative measurements (i.e., late loss index) have allowed more effective comparisons between different interventional approaches. However, the most commonly applied angiographic definition for in-stent restenosis is the dichotomous endpoint of a diameter stenosis of ≥50% at the previously treated vessel site. Clinical restenosis is defined as the need for ischemia-driven target-vessel revascularization (TVR) in follow-up and has emerged as a useful surrogate marker for angiographic restenosis.
PATTERNS
The description of in-stent restenosis has evolved from the initial categorization of lesions into either focal (<10 mm in length) or diffuse (>10 mm in length) patterns (14). Mehran et al. (15) expanded and refined the classification of in-stent restenosis and, in doing so, applied these new definitions to obtain prognostic information regarding long-term outcomes following repeat revascularization. This classification included four patterns of in-stent
restenosis (Fig. 35.1): Pattern I or “focal” was defined as lesions of ≤10 mm in length. Pattern II or “diffuse intrastent” restenosis was defined as lesions of >10 mm in length that are confined to the stent. Pattern III or “diffuse proliferative” restenosis describes lesions of >10 mm in length that extend beyond the stent margin. Pattern IV is “total occlusion” restenosis. Of the 288 lesions classified by Mehran et al., pattern I was most common after bare metal stenting (BMS) and represented 42% of patients. This focal pattern has a more favorable response to treatment, with target lesion revascularization (TLR) rates of 19% following reintervention. Escalating TLR rates following reintervention were observed with other patterns of in-stent restenosis: 35% for Pattern II, 50% for Pattern III, and 83% for Pattern IV.
restenosis (Fig. 35.1): Pattern I or “focal” was defined as lesions of ≤10 mm in length. Pattern II or “diffuse intrastent” restenosis was defined as lesions of >10 mm in length that are confined to the stent. Pattern III or “diffuse proliferative” restenosis describes lesions of >10 mm in length that extend beyond the stent margin. Pattern IV is “total occlusion” restenosis. Of the 288 lesions classified by Mehran et al., pattern I was most common after bare metal stenting (BMS) and represented 42% of patients. This focal pattern has a more favorable response to treatment, with target lesion revascularization (TLR) rates of 19% following reintervention. Escalating TLR rates following reintervention were observed with other patterns of in-stent restenosis: 35% for Pattern II, 50% for Pattern III, and 83% for Pattern IV.
TIME COURSE OF IN-STENT RESTENOSIS
The time course of in-stent restenosis is similar to that of percutaneous transluminal coronary angioplasty (PTCA), because restenosis is extremely uncommon in the first month. The risk of restenosis plateaus by 3 to 6 months, correlating with in-stent tissue accumulation peaking around 6 months (16,17). The course of restenosis after 6 months was defined by Kimura et al. (17), who performed serial angiograms on patients treated with Palmaz-Schatz stents and demonstrated a gradual increase in MLD over the ensuing 2.5 years, likely secondary to neointimal shrinkage or organization. This late regression should be considered in asymptomatic patients with moderate degrees of in-stent restenosis.
PREDICTORS OF RESTENOSIS
A variety of factors have been found to correlate with an increasing risk of restenosis. Predictors of restenosis include patient and clinical variables, procedural factors, and lesion or vessel characteristics.
Patient and Clinical Variables
The most consistently described predictor of restenosis is the presence of diabetes mellitus. Patients with diabetes are known to have greater risks of restenosis following PTCA (18) and similarly, diabetes has consistently been shown to increase the risk of restenosis following coronary stenting (19, 20, 21). Intravascular ultrasound (IVUS) studies have revealed that patients with diabetes manifest an increased proliferative response, resulting in more pronounced neointimal formation following coronary interventions (19,22). Thus, diabetes was not surprisingly found to be the only independent predictor of diffuse-type in-stent restenosis using stepwise multiple regression analysis in a study of 114 diffuse-type lesions (23).
Whereas other clinical variables such as unstable angina had been shown to be predictive of restenosis following angioplasty (24), it does not appear to be a predictor of in-stent restenosis (25). Female gender has variably been associated with an increased risk of restenosis; however, Mehilli et al. found that women experience a lower risk of restenosis following coronary stent implantation despite having smaller vessel size and increased rates of diabetes mellitus (26). Additionally, contact allergy to nickel and molybdenum has been associated with an increased rate of restenosis (27).
The relationship between genetic factors and the risk of in-stent restenosis also has been examined (28,29). Initial observations supporting the association of the deletion allele of the insertion/deletion (I/D) polymorphism of the angiotensin I-converting enzyme (ACE) gene as an independent predictor of late loss following restenosis following stenting (30,31) have not been confirmed (32, 33, 34). However, studies have shown that the elevated ACE plasma levels are associated with in-stent restenosis (31,34). The role of polymorphisms of platelet glycoprotein IIIa (PlA) in restenosis also has been examined. Kastrati et al. found a significant association between the PlA polymorphism of glycoprotein IIIa (GP IIIa) and in-stent restenosis in 1,150 consecutive patients following successful stent implantation. The risk of restenosis was especially strong in women who were homozygous for the PlA2 allele of the GP IIIa gene (35). Mutant genotypes of the methylenetetrahydrofolate reductase gene also have been associated with an increased risk of restenosis (36). The role of these and other genetic factors in interventional cardiology continues to evolve and be defined.
Procedural Factors
Despite improvements in catheter-based techniques and equipment, procedural factors continue to be important,
because the vascular integrity and cellular homeostasis are disrupted during coronary intervention.
because the vascular integrity and cellular homeostasis are disrupted during coronary intervention.
Stent design not only influences flexibility, deliverability, and radial strength, but also affects in-stent restenosis. Escaned et al. found that the restenosis rate in 523 successfully stented lesions ranged from 10% for multicellular stents and 20% for slotted-tube stents to 46% for coil stents and 49% for self-expanding stents (37). A large, unselected population of 4,510 consecutive patients treated with a variety of stent designs supported stent type as a strong predictor of restenosis, with the incidence of restenosis ranging from 20% to 50.3%, depending on the stent type employed (38).
The Strut Thickness Effect on Restenosis Outcome (ISAR-STEREO) trial examined the role of stent strut thickness and found thicker struts to be a significant predictor of in-stent restenosis in stents with a similar design (39). The angiographic restenosis rate was 15% in the thin-strut group compared to 25.8% in the thick-strut group (p = 0.003).
The importance of stent length in predicting restenosis was evaluated by Kereiakes et al., who showed that increases in stent length at any given postprocedure MLD increased the risk of restenosis (40). In contrast, Kornowski et al. evaluated 117 patients treated with multiple (≥3) stents using IVUS guidance, and compared 1 year outcomes to patients receiving one or two stents (41). The placement of multiple stents was associated with a higher rate of non-Q-wave MI; however, overall major cardiac events and TLR rates did not significantly differ at 1 year between the two groups.
Studies using IVUS in patients undergoing stent implantation supported previous PTCA findings that the postprocedure cross-sectional area (CSA) is a important predictor of restenosis (42). Moussa et al. studied 921 lesions in 628 patients who had undergone successful IVUS-guided stent implantation (43). An in-stent minimum lumen cross-sectional area (ISMLCSA) of ≥9 mm2 was associated with an 8% restenosis rate, compared to 29% when the ISMLCSA was ≤9 mm2 (p <0.0001). However, this cross-sectional area may be difficult if not impossible to achieve in smaller vessels.
The relationship between in-stent restenosis and high-pressure balloon inflation is controversial. Although high-pressure deployment optimizes stent expansion and postprocedure CSA, this approach may lead to more vascular injury and increased neointimal proliferation. Although early studies indicated that late loss increased with higher-pressure inflations (44,45), larger randomized trials did not support an increased restenosis rate using routine high-pressure inflations (46,47).
Lesion and Vessel Characteristics
Certain lesion and vessel characteristics, such as lesion length and location, reference vessel size, and the extent of plaque, can impact restenosis rates.
The length of the treated lesion is an important predictor of restenosis. Kobayashi et al. reviewed 1,090 lesions of varying length in 725 patients undergoing stent implantation (48). Lesion length was determined to be an independent predictor of restenosis. Kastrati et al. studied 2,736 consecutive patients who underwent coronary stent placement and noted higher restenosis rates in lesions of ≥15 mm, compared with lesions of <15 mm (49). Treatment of long lesions can require the use of longer stents (40), multiple stents (25,40), and overlapping stents, all of which may increase the restenosis rate. Other lesions, such as ostial lesions (14,50,51), bifurcating lesions (52,53), and chronic total occlusions (54), also are at increased risk for restenosis.
Small reference vessel size has consistently been demonstrated to be a risk factor for in-stent restenosis. The intimal hyperplastic thickness appears to be independent of stent size and thus impacts small vessels to a greater extent (55). A study of 2,602 patients determined that patients undergoing stent implantation in small vessels were at higher risk for restenosis (56). The angiographic restenosis rate was 38.6% in vessels of <2.8 mm, 28.4% in vessels of 2.8 to 3.2 mm, and 20.4% in vessels of >3.2 mm (p <0.001). A recent meta-analysis of 11 studies (3,541 patients) indicated that BMS is a better treatment than PTCA in vessels of <3 mm, as demonstrated by lower restenosis and TLR rates (57).
A large preinterventional plaque burden (plaque/total arterial area), as assessed with IVUS, has been shown to increase restenosis rates (50). Plaque burden that was associated with increased accumulation of in-stent neointimal hyperplasia on serial angiographic and IVUS studies following implantation of Palmaz-Schatz stents (58) prompted interest in debulking strategies prior to stent implantation in an effort to reduce restenosis. Unfortunately, outcomes with directional and rotational atherectomy prior to stent implantation failed to improve restenosis rates (59,60).
A prior history of in-stent restenosis contributes to the risk for future episodes of restenosis (54,61,62). Lesions with diffuse-type in-stent restenosis (length >10 mm) include both the proliferative and total-occlusion subtypes and commonly require repeat revascularization due to recurrent in-stent restenosis (15,51). The temporal relationship between intervention and the initial episode of in-stent restenosis can predict risk for additional restenotic events. Early in-stent restenosis (<90 days after stent implantation) was significantly associated with recurrent episodes compared to in-stent restenosis occurring after 90 days (51).
MECHANICAL TREATMENT OF IN-STENT RESTENOSIS
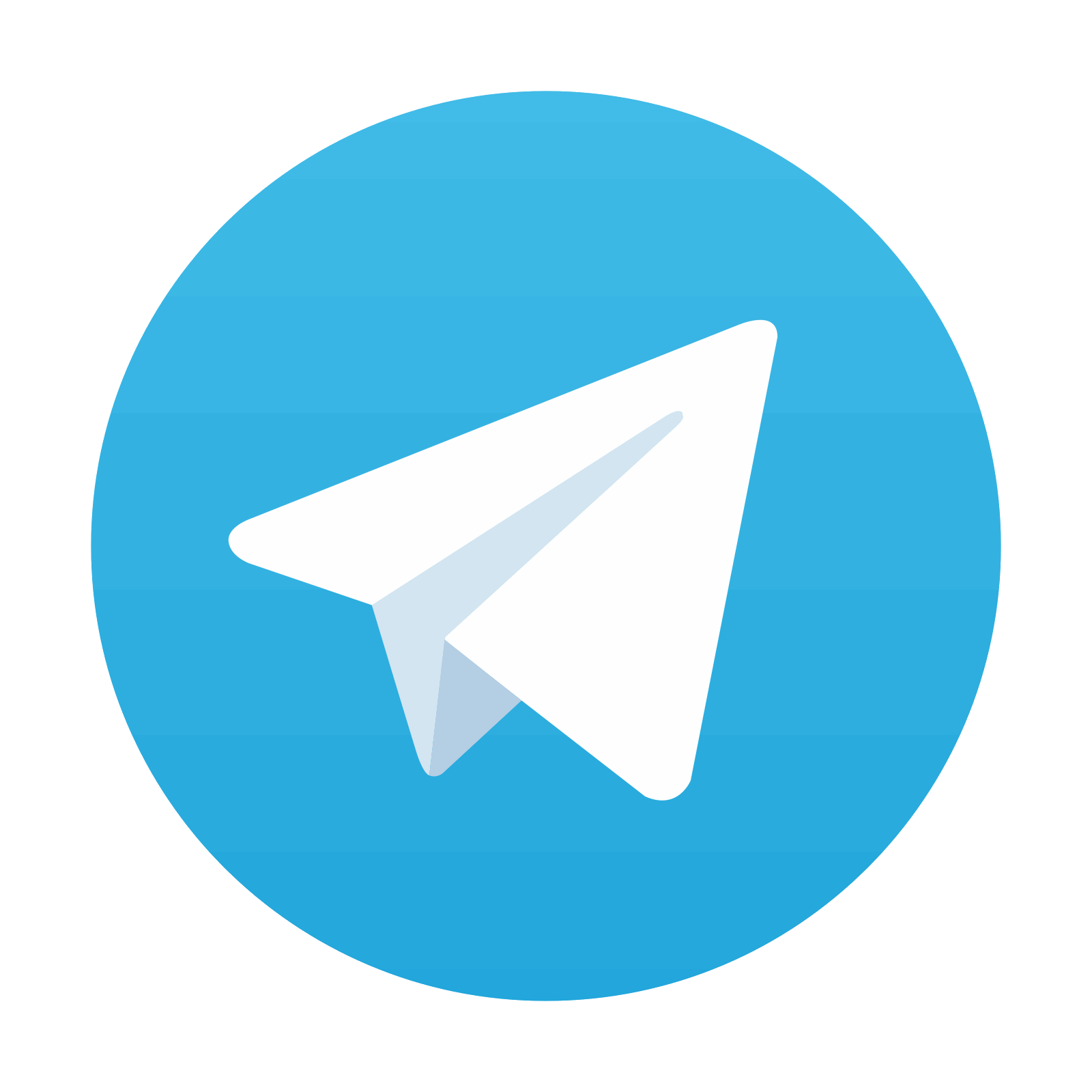
Stay updated, free articles. Join our Telegram channel

Full access? Get Clinical Tree
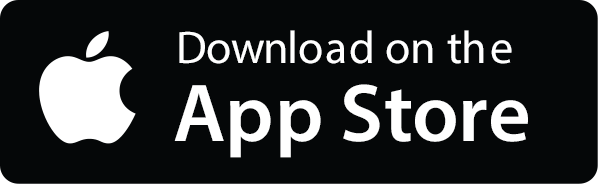
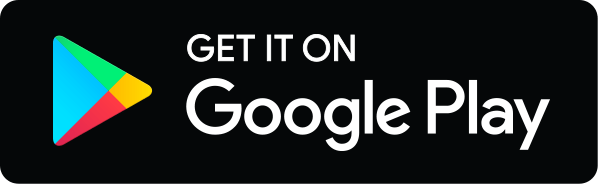