Chapter 6
Implementing respiratory muscle training
GENERAL PRINCIPLES OF FOUNDATION IMT
In Chapters 4 and 5, the functional benefits and practical methods of respiratory muscle training (RMT) were reviewed. From the evidence presented there, it was apparent that the most widely used and validated type of RMT is pressure threshold inspiratory muscle training (IMT). Accordingly, the guidance provided in this chapter is for the application of this type of training.
The diversity of potential applications for IMT makes it impossible to provide specific guidance for all potential applications. Accordingly, this chapter provides guidance that is either generic or applies to the most popular and best-supported application of pressure threshold inspiratory muscle training (IMT), viz., the improvement of inspiratory muscle function for the purposes of alleviating dyspnoea and / or improving exercise tolerance. However, in the context of indications for IMT, readers are encouraged to consider the application of the techniques described in this chapter to alternative settings, e.g., weaning from mechanical ventilation. In this respect, a number of non-exercise-related indications are also provided (see also Chs 3 and 4 and Box 6.1).
Context of RMT in the treatment pathway
The evidence presented in Chapters 3 and 4 identified a wide range of patients in whom: (1) disease can create an imbalance in the demand / capacity relationship of the respiratory pump, and / or (2) IMT has been shown to improve one, or multiple, clinically meaningful outcomes. The diversity of patients, and the healthcare systems that care for them, makes prescriptive guidance on the place of IMT in the care pathway inappropriate. However, the vast majority of patients who could benefit from IMT are living with long-term chronic conditions such as respiratory disease, heart failure, obesity and neuromuscular disease. For these patients, the point at which IMT is delivered will be determined by local policies for the management of chronic disease. At the time of writing, the United Kingdom’s National Health Service (NHS) is undergoing a major restructuring that is shifting the delivery of services to locations that are closer to its service users (patients). This inevitably means that services hitherto delivered in specialist units within secondary care will in future be delivered by ‘up-skilled’ primary care providers, or by local subcontractors to primary care providers. Simultaneously, there is likely to be an increase in the diversity of service providers for services such as rehabilitation, which have typically (but not exclusively) been provided in secondary care by NHS employees. These changes within the NHS are likely to bring its structure and delivery framework closer to that of the private healthcare systems that exist in other countries. The implications of such changes are that IMT could be provided in the patient’s home as part of, for example, a primary care ‘breathing clinic’ service delivered by a nurse specialist, or by a specialist rehabilitation service within secondary care – either of which might be delivered by private sector or NHS providers. In practical terms, these changes should make very little difference to the patients’ experience, provided that the appropriate expertise exists to assess their needs, and to provide the guidance required to support the optimal implementation of IMT whether in their own homes or within an outpatient setting.
The evidence presented in Chapter 4 supports the use of IMT both as a stand-alone intervention, and as part of a multi-dimensional rehabilitation programme, for a wide range of patients. The benign nature of IMT, and the ability of virtually all patients to meet the demands necessary to elicit improved function, make it a versatile intervention for patients with dyspnoea and exercise intolerance, or indeed for those who avoid activity because of their fear of exertional dyspnoea. Furthermore, co-morbidities that preclude exercise training are no barrier to IMT, making it an ideal intervention for severely compromised patients. A systematic review of home-based physiotherapy interventions for patients with chronic obstructive pulmonary disease (COPD) found that home-based IMT significantly improved dyspnoea (Transitional Dyspnoea Index) by 2.36 units, compared with controls (Thomas et al, 2010). Furthermore, a recent systematic review comparing IMT and rehabilitation concluded that the magnitude of improvement in exercise tolerance (and associated parameters) in response to IMT was indistinguishable statistically from that derived following a period of exercise training ‘(O’Brien et al, 2008). In other words, IMT yielded the same benefits as exercise training, making it a very cost-effective stand-alone intervention. The ideal setting for the stand-alone implementation of IMT is primary care. The barrier to implementation in this setting is a general lack of specialist expertise relating to IMT, which is not typically part of the curriculum for respiratory clinicians. This book endeavours to facilitate the acquisition of that expertise and the inclusion of IMT within the clinical curricula of specialist nurses, physiotherapists and physicians.
Finally, the context of IMT needs to be defined in terms of its application as either ‘Foundation IMT’ or ‘Functional IMT’ (see Ch. 7). In this book, the stand-alone implementation of IMT has been termed ‘Foundation IMT’, which is also the first phase of a two-phase process that leads to Functional IMT. Respiratory muscles have a number of important non-respiratory roles (see Ch. 3), which is why patients find walking more ‘dyspnoegenic’ than cycling. A training modality that is applied widely in sport is ‘functional’ training, i.e., the training of functional movements (e.g., a squat), rather than the training of muscles (e.g., leg extensors). This approach has not yet been applied to IMT in patients. However, for people who already have overloaded respiratory muscles, the competing functions of, say, postural control may be sufficient to render an everyday activity impossible because of intolerable dyspnoea. Accordingly, the rationale for implementing a training modality that addresses these competing demands is very strong. The specialist nature of Functional IMT makes its implementation the preserve of rehabilitation specialists and, therefore, probably unsuitable for implementation outside of the rehabilitation setting.
Indications and contraindications for IMT
Chapters 3 and 4 presented the theoretical and evidence-based rationales for RMT, respectively. The rationale for RMT is based upon ameliorating imbalance within the demand / capacity relationship of the respiratory muscles. This information is summarized in Box 6.1. The following section contains a more detailed overview of these issues.
Indications
Chapter 3 summarized the wide range of conditions in which respiratory muscle dysfunction is present, as well as the disease-specific factors that contribute to an imbalance of the demand / capacity relationship of the inspiratory and expiratory muscles. Chapter 4 summarized the evidence for improvement in clinical outcomes following RMT. For the reasons provided in Chapters 4 and 5, the current chapter deals with training of the inspiratory muscles. The simplistic approach to identifying patients who could benefit from rebalancing of the demand/capacity relationship is to assess maximal inspiratory pressure (MIP) and to apply a diagnostic criterion for ‘weakness’ based upon reference to population norms (see Ch. 1), e.g., 60% of the predicted normal value.
However, this approach fails to take account of a number of important issues. First, strength (MIP) is a one-dimensional index of muscle function that is difficult to interpret in limb muscles, but is infinitely more difficult for the respiratory muscles. This is primarily because MIP is merely a surrogate measure of strength, and is several steps removed from the true force-generating capacity of the inspiratory muscles (ATS / ERS, 2002). Secondly, in patients with a tendency to hyperinflate, MIP is not a fixed index of function but one that is dependent upon the prevailing degree of hyperinflation. Thirdly, the interpretation of MIP needs to be made in the context of the prevailing loading conditions, and work requirements, of the inspiratory muscles – in other words, whether the demand / capacity relationship of the inspiratory muscles is in a state of imbalance. In the presence of an increased demand for inspiratory muscle work, a muscle that appears ‘normal’ in absolute terms is rendered weak functionally.
In most chronic conditions, inspiratory muscle dysfunction manifests as dyspnoea and exercise intolerance. Thus, a more pragmatic approach to assessing the extent to which the inspiratory muscles are functionally overloaded is to consider the intensity of dyspnoea that an increased demand for inspiratory muscle work provokes. Any patients who complain of dyspnoea that limits their activities of daily living are therefore candidates for IMT (see also the sections ‘Patient selection’ below, and ‘Assessing patient needs’ in Ch. 7).
Notwithstanding the preceding rationale, the absence of exertional dyspnoea should not be a reason to discount IMT as a potential management tool for exercise intolerance. The inspiratory muscle metaboreflex described in Chapters 3 and 4 is probably the reason that a substantial number of patients with respiratory disease stop exercising because of leg discomfort, rather than dyspnoea (Hamilton et al, 1996). In such cases, improving inspiratory muscle function raises the intensity of inspiratory muscle work associated with metaboreflex activation. This can increase the exercise tolerance of non-dyspnoeic patients by improving leg blood flow.
Finally, readers are encouraged to consider the application of the techniques described in this chapter to ameliorating the demand / capacity imbalance of the inspiratory muscles in non-exercise-related contexts. Some indications for these applications are provided in Box 6.1.
Contraindications
Implicitly, the measurement of maximal respiratory pressures is associated with larger pressure changes, so it is perhaps appropriate to consider also the risks associated with these measurements, as they represent a ‘worst case scenario’ for the risk of barotrauma during IMT. The first published measurements of maximal respiratory pressures were made by Black & Hyatt in the late 1960s (Black & Hyatt, 1969), and the first studies of IMT by Leith & Bradley (1976). Thus, the research base spans over 40 years, but there is no mention of any complications or adverse events in any of the studies within the published literature. In other words, of the hundreds of studies of IMT and / or maximal inspiratory mouth pressure measurement (incorporating many thousands of patients), no study has ever reported a patient withdrawal due to an event precipitated by barotrauma. Similarly, there are no case studies of IMT reporting isolated incidents of adverse events during the treatment, or assessment of any person undertaking measurement of maximal inspiratory pressures (MIP), or IMT. Despite the intrathoracic pressure swings, even patients with heart failure experience no deterioration of their cardiac output during training (McConnell et al, 2005).
• A history of spontaneous pneumothorax (i.e., not due to traumatic injury)
• A pneumothorax due to a traumatic injury that has not healed fully
• A burst eardrum that has not healed fully, or any other condition of the eardrum.
There is also an important subgroup of asthma patients for whom IMT is inappropriate, i.e., those with unstable asthma and abnormally low perception of dyspnoea. In these patients, it is the disruption of the normal relationship between dyspnoea and bronchoconstriction that is thought to contribute to their poor adherence to medication and consequent clinical instability (Magadle et al, 2002). Since IMT may reduce their perception still further, it is inadvisable.
Unlike pharmacological treatments, IMT has no side effects or drug interactions.
Patient selection
There remains a perception that only patients with evidence of inspiratory muscle weakness (see section ‘Assessment of respiratory muscle function’) or ventilatory limitation during physical activity can benefit from IMT. The logic of this has always been questionable, particularly given that Olympic standard athletes are known to benefit, and they certainly have no compromise to the function of their ventilatory pump. The misconception is perpetuated by the fact that patients with a MIP < 60 cmH2O appear to show larger improvements than those with stronger inspiratory muscles (Lotters et al, 2002; Gosselink et al, 2011). However, even when inspiratory muscle weakness is not an inclusion criterion, improvements in dyspnoea and exercise performance still follow IMT (Lotters et al, 2002). A pragmatic way to view these observations is that weaker individuals have more to gain from IMT.
In addition, it is also worth noting that research has shown repeatedly that there is a close correlation between the post-IMT decrease in dyspnoea and the improvement in MIP, regardless of MIP at baseline, or whether the participants are patients or healthy young athletes. Thus, it is the extent to which MIP can be improved that dictates the magnitude of improvement in dyspnoea. In this respect also, weaker patients have more to gain, as they appear to show the largest improvements in MIP after IMT (Lotters et al, 2002; Gosselink et al, 2011).
Notwithstanding this, there is nevertheless a dose–response relationship that relates the magnitude of improvement to the number of completed IMT sessions (Winkler et al, 2000), i.e., the more diligently the IMT training regimen is adhered to the better is the outcome. This means that patient motivation is arguably the most important criterion for enrolment. Accordingly, the best candidates for IMT are well-motivated individuals with dyspnoea and / or exercise intolerance.
See Chapter 7 (section ‘Assessing patient needs’) for guidance on assessing dyspnoea and the functional limitations it imposes.
PRACTICAL ISSUES
Posture during training
Recumbent and semi-recumbent postures are known to impair respiratory muscle function, which is optimized in upright positions (Koulouris et al, 1989; Griffiths & McConnell, 2012). Accordingly, posture has an influence on the ability to overcome a given load during IMT. This concept will be developed in Chapter 7 in the context of functional IMT, but posture also has a role to play in obtaining optimal results during the Foundation phase, especially in patients who are unable to adopt an upright position. The premise for this is that larger improvements can be achieved in a shorter time frame if training overload is maximized. Since inspiratory muscle function is optimized in upright positions, the highest training loads can be tolerated when seated or standing, and these represent the ideal positions to commence a period of IMT.
However, there are some caveats to the use of seated or standing postures. The respiratory muscles contribute to postural control, which is a confounding influence during IMT. If the inspiratory muscles are relieved of their postural role, they are able to ‘focus’ upon breathing. Consequently maximal inspiratory pressure (MIP), maximal expiratory pressure (MEP) and maximal voluntary ventilation (MVV) are all higher in a standing, supported, leaning posture (Cavalheri et al, 2010). Thus standing, and leaning forward against the back of a chair, table, window ledge or just by placing the hands on the knees, increases the tolerable training load and the number of repetitions that can be achieved. The logic of this is immediately apparent when one considers what people do when they are breathless: they instinctively lean on something so that their respiratory muscles can be focussed on the act of breathing.
Optimizing breathing technique
Maximizing the training stimulus to the inspiratory muscles, as well as the range of adaptations it elicits, is arguably the most important objective in terms of achieving the best possible training results. Choosing the right type of training device is the first step to maximizing results. In the case of the methods described in this book, the focus is on pressure threshold IMT, for which there are two evidence-based products available (see Ch. 5, Table 5.1). Once the training device has been selected, the second step is to optimize the training stimulus that it generates. Unfortunately, simply breathing through a training device will not achieve this. However, the following section explains the optimal approach for pressure threshold IMT and the physiology that underlies this approach. Firstly, the fundamentals of ‘good’ breathing technique will be considered, i.e., how to develop efficient, comfortable, diaphragm-focussed breathing. Secondly, the optimization of breathing pattern (the combination of respiratory flows, volumes and the timing of breaths) for IMT will be explained.
Diaphragm breathing
Disease-related changes to the mechanics of breathing can lead to a reduction in diaphragm mobility, and, consequently, its contribution to breathing. This phenomenon has been well documented in COPD where it is largely due to hyperinflation (Unal et al, 2000). Diaphragm breathing training programmes have been shown to improve diaphragm mobility and exercise tolerance (Paulin et al, 2007; Yamaguti et al, 2012), as well as breathing pattern and oxygen saturation (Fernandes et al, 2011). However, in patients with COPD who have asynchronous thoracoabdominal motion, diaphragm breathing may exacerbate dyspnoea (Fernandes et al, 2011) and reduce the efficiency of breathing (Gosselink et al, 1995). Consequently, the appropriateness of diaphragm breathing for a specific patient needs to be evaluated on a case-by-case basis. Given that the likely readers of this book are healthcare professionals with an interest, and considerable expertise, in the realm of ‘breathing’, there is a danger of ‘teaching grandmother to suck eggs’ in providing advice on diaphragm breathing. However, relearning normal efficient breathing strategies is the ‘essential groundwork’ that underpins Foundation IMT. Accordingly, expert readers are merely encouraged to ensure that patients receive appropriate diaphragm breathing training as part of their Foundation IMT. For those readers who would welcome some further guidance on teaching diaphragm breathing, please feel free to read on, but also feel free to seek this elsewhere, as the following section is merely included for the sake of completeness.
This first phase of the process of developing efficient breathing can be thought of as ‘getting in touch with the diaphragm’. Human babies breathe almost exclusively with their diaphragm, but most human adults have lost touch with their most important respiratory muscle and become ‘chest breathers’, i.e., they breathe using the accessory muscles of the rib cage. Without re-establishing diaphragm breathing, IMT will neglect the most important inspiratory muscle. However, this emphasis upon diaphragm breathing should not be confused with the objective of focussing the IMT upon the diaphragm; this is not what is being advocated (the reasons for this are explained below in the section ‘Breathing pattern during IMT’). Rather, the objective is to ensure that the complex inspiratory musculature is used holistically, and in concert, during both training and activities of daily living. This section provides guidance on how to reintroduce the diaphragm into the normal, instinctive process of breathing, so that when breathing is challenged, the diaphragm is a subconscious, central part of the response. The mechanism by which isolated, conscious activation of muscles restores their automatic activation is poorly understood, but it has been likened to flipping a rope back into a well-worn groove. Short-term repetition of motor tasks has been shown to induce cortical reorganization in a range of activities including the practice of diaphragm breathing (Demoule et al, 2008). These changes are consistent with the ability of task repetition to enhance neuromuscular functioning. For example, for muscles that are involved in automatic, anticipatory postural adjustments, such as transversus abdominis, it is thought that isolated specific training can normalize previously abnormal patterns of motor activation (Tsao & Hodges, 2007).
Visual and tactile feedback both provide very helpful means of coaching diaphragm breathing. To achieve this, the patient should be positioned in front of a mirror (large enough to see their torso), with palms placed lightly on the ribs with the fingers facing forwards and the tips of the fingers almost touching at the end of a normal exhalation (Fig. 6.1). The exercise should commence by describing (to the patient) the movement of the diaphragm during inhalation and the effect this has on the abdominal wall, i.e., the diaphragm flattens and moves downwards like a piston, pushing the abdominal organs downwards and outwards. A demonstration is helpful, after which the following instructions can be used to guide the patient’s breathing:
Figure 6.1 Placing the hands on the abdomen to feel movement of the abdominal wall during diaphragm breathing: (A) inhalation, (B) exhalation.
• Commence diaphragm breathing at the end of a normal exhalation
• Keep your abdomen, shoulders and chest relaxed, and take a deep, slow inhalation through your nose
• Watch the movement of your abdomen and rib cage in the mirror
• On inhalation, you should see and feel your abdomen bulge forwards and your ribs move sideways and forwards
• Watch your finger tips and try to move them apart using your abdomen as you inhale
• If your chest rises, the diaphragm is not being used properly – relax the shoulders and chest
• Exhalation should be relaxed, with no muscle activity – allow the air to ‘fall out’ of your chest as your lungs and rib cage ‘spring’ back
• You may find it helpful to purse your lips as this helps you to breathe out further, and also slows your exhalation, but be careful not to force the air out – relax and let the air fall out
• Be careful not to hold the breath at the end of the inhalation – relax and let the air fall out.
Use of an external breathing pacer that provides an auditory and visual cue (obtainable via www.physiobreathe.com/apps) can be very helpful for supporting the process of developing an efficient breathing pattern, i.e., deep breaths accompanied by a slow exhalation. In addition, a Theraband®, or similar wide elastic resistance band, wrapped around the lower ribs can help to focus effort on this area. The resistance of the band intensifies the sensation of working with the diaphragm (if the patient still finds the exercise too challenging, see below). Diaphragm breathing should be practised until the patient feels confident about how to activate the diaphragm, and what a diaphragm inhalation feels and looks like. Once this technique has been mastered, it should be tried with eyes closed, focussing on the sensation of the air filling the lungs and the sensation of the diaphragm plunging down into the abdomen.
During the diaphragm breathing exercise, breathing rate should be no more than 12 breaths per minute, and preferably 8. With practice it might be possible to reduce this to 6 breaths per minute. The inhalation phase will typically be slightly longer than exhalation because exhalation is passive, i.e., the only phase that is actively controlled is inhalation. However, this will not be the case in obstructed patients, who typically take longer to exhale. Patients can count the breath in and out. For example, for an obstructed patient, if the objective is 10 breaths per minute this means that each breath should take 6 seconds (60 / 10); count ‘1–2’ during inhalation, and ‘3–4–5–6’ during exhalation. It is vital that the extension of the breath duration is not achieved by breath holding at the end of inhalation or exhalation; the increase in duration should come about through a slower, deeper, more controlled breath so that each phase starts immediately the other ends. Remind patients that exhalation should be passive, and that the air should ‘fall out’ of the lungs as quietly as possible. There are some useful biofeedback applications for smartphones and tablet computers that can facilitate the development of slow, deep breathing, which healthcare professionals can use in a clinic setting and that can be suggested to patients as tools to help them to practise at home (see www.physiobreathe.com/apps). These tools set the duration of each phase of breathing and the breathing frequency, count the number of breaths, and time the practice session.
Developing breath control is a skill that can and should be practised because it maximizes breathing efficiency and minimizes the distracting influence of breathing discomfort. The first stage in achieving this is achieving diaphragm-focussed breathing, which provides the ‘groundwork’ for good breath control; once this becomes second nature the second phase is much easier. Phase two involves developing breath control to overcome the natural desire to breathe as quickly as possible during periods when breathing demand is high and when breathing discomfort / distress is present. By not allowing breathing to become rapid and shallow, voluntary breathing control imposes a deep, slow, calm and efficient pattern. The only time that this can be practised properly is in situations where breathing demand / distress is high, but only when patients are clinically stable. So they should be encouraged to practice deep, slow breath control during periods when they feel dyspnoeic. Keeping breathing calm and relaxed under stressful conditions can help to minimize stress and anxiety, and build a sense of mastery. See also Chapter 7 (section on ‘Breath control’) for some exercises that promote deep, slow, controlled breathing.
Breathing pattern during IMT
Tidal volume
Although muscle is a very adaptable tissue, training adaptations are also highly specific to the nature of the training stimulus. Adaptations elicited by IMT are specific to a number of characteristics of the training stimulus, including the lung volume at which training takes place (see Ch. 5). The practical implication of this is that IMT should be undertaken across the widest range of lung volume possible, i.e., from as close to residual volume as possible to the point at which it is impossible to inhale any more. Failure to do this will lead to suboptimal adaptation at some lung volumes, which is particularly important in patients who hyperinflate when minute ventilation increases. An important consideration in maximizing tidal volume (VT) during IMT is the training load; loading too ‘heavily’ can compromise both the VT that can be achieved and the amount of work that can be undertaken during training, which will also impair the training response (Box 6.2). This impairment occurs because VT has a strong influence upon the amount of work done per breath, and the most important determinant of the ability to inhale deeply is the training load (McConnell & Griffiths, 2010). Functional weakening of the inspiratory muscles during inhalation means that, if the load is too high, the inspiratory muscles are not able to overcome the load at higher lung volumes (where the inspiratory muscles are weaker), despite maximal effort. The heavier the load, the more severely the breath is ‘clipped’.
This means that the training load must be set with these factors in mind. Figure 6.2 illustrates the interrelationships of maximal inspiratory pressure (MIP), lung volume and training load, as well as the effect of fatigue on the VT that can be achieved. Note that the breath volume is clipped progressively earlier in the breath with increasing loads.
Figure 6.2 The interactions between inspiratory muscle strength (black line), various training loads (40%, 50%, 60% and 70% of maximal inspiratory pressure [faded lines]), and the breath volume that can be achieved during training, as well as the effect of fatigue (dotted lines). For example, at 40% of MIP it is possible to inhale to around 60% of vital capacity, whereas at 70% of MIP it is only possible to inhale to around 35% of vital capacity. (Adapted from McConnell AK, 2011. Breathe strong, perform better. Human Kinetics, Champaign, IL, with permission.)
Inspiratory flow rate
To understand the advice below, it is necessary to return briefly to the force–velocity relationship of muscle. Essentially, this property dictates that the faster a muscle contracts, the less force it is able to generate, and vice versa (see Ch. 4, Fig. 4.1). An example of the force–velocity relationship at work is the difference in force one can exert on the pedals when cycling in a low gear compared with a high gear. This property can be exploited to optimize the training stimulus that the muscle receives. For example, assume that, because of the force–velocity relationship, as the rate of muscle contraction doubles the force it can generate is halved, despite the same [maximal] effort being applied under both conditions. When muscles contract maximally at any speed, the number of muscle fibres that are recruited to the contraction is also maximized, despite the fact that faster contractions result in lower forces. Now consider the effect of doubling the rate of contraction slightly differently: when a muscle is contracting very slowly to move a load that requires, say, half its maximal force-generating capacity, doubling the rate of contraction against the same load now requires ~100% of the muscle’s force-generating capacity (because its ability to generate force has been halved). This means that ~100% of the muscle fibres are recruited for half the force. This can be turned to an advantage during IMT because it means that it is possible to train close to 100% of a muscle’s force-generating capacity no matter what load is being applied, provided that the load is moved as fast as possible (i.e., with maximal effort). Under any given loading condition, fast muscle contractions recruit more muscle fibres than slow contractions (Aagaard et al, 2000). Furthermore, recent evidence suggest that training improvements following bench press training are maximized at higher velocities of muscle shortening (Padulo et al, 2012). Therefore, maximal effort ensures maximum velocity, and the recruitment of the greatest number of muscle fibres.
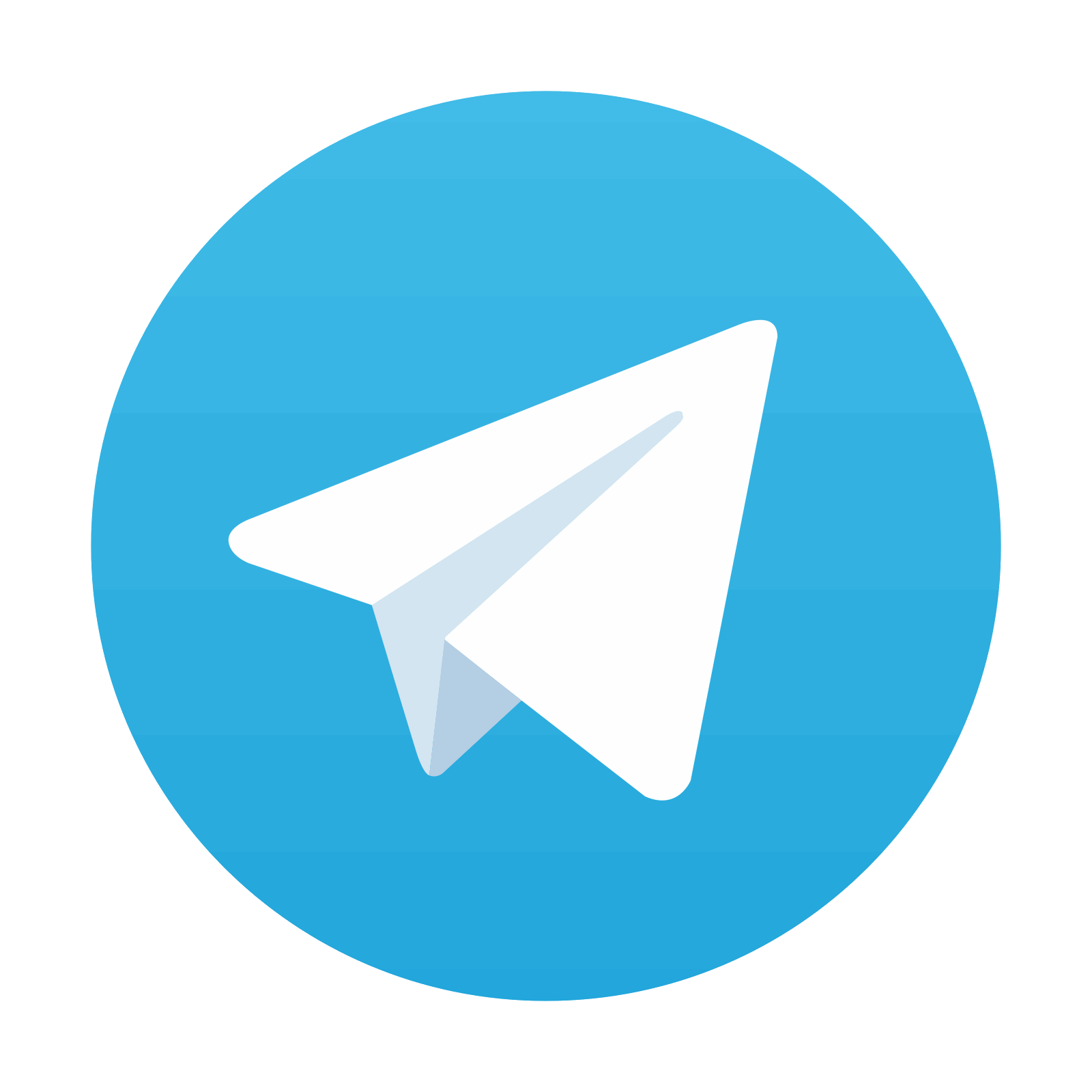
Stay updated, free articles. Join our Telegram channel

Full access? Get Clinical Tree
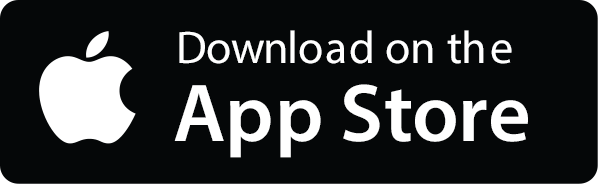
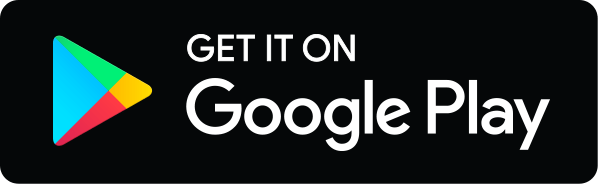
