Background
Left ventricular (LV) dysfunction is common in adults late after repair of tetralogy of Fallot (TOF). The early detection of myocardial dysfunction may be important, but LV myocardial strain and dyssynchrony are not well studied in children with TOF. The objective of this study was to investigate LV strain and dyssynchrony in asymptomatic children and adolescents after contemporary repair of TOF. The hypothesis was that impaired LV myocardial mechanics are related to pulmonary regurgitation, right ventricular (RV) enlargement, and exercise capacity.
Methods
Children and adolescents were prospectively studied after TOF repair. LV regional strain and dyssynchrony were assessed using two-dimensional speckle-tracking echocardiography. Ventricular volumes, ejection fraction, and pulmonary regurgitation were assessed using magnetic resonance imaging. Exercise capacity was determined using metabolic exercise testing.
Results
One hundred twenty-four subjects (53 patients with TOF and 71 controls) were studied. Regional circumferential (e.g., basal lateral wall, −15.0 ± 7.0% vs −19.0 ± 7.0%, P = .02) and radial (e.g., basal posterior wall, 32.0 ± 18.0% vs 48.0 ± 21.0%, P < .001) LV strain and longitudinal septal strain (−18.5 ± 3.5% vs −20.2 ± 2.8%, P = .01) were significantly reduced in patients with TOF compared with controls. LV mechanical dyssynchrony indices were not significantly different between groups (e.g., standard deviation of time to peak circumferential strain, 52.5 ± 40.4 vs 50.5 ± 27.1 msec, P = .81). Higher pulmonary regurgitation volume and larger RV end-diastolic volume were associated with decreased LV radial strain ( P = .09). There was no association between LV longitudinal, radial, or circumferential dyssynchrony and indexed pulmonary regurgitation flow volume, RV end-diastolic volume, or RV ejection fraction.
Conclusions
LV circumferential and radial strain are significantly reduced in children and adolescents after TOF repair and are associated with pulmonary regurgitation and RV dilatation. Resting LV mechanical dyssynchrony does not appear to contribute to early impaired LV strain in this population.
Although tetralogy of Fallot (TOF) predominantly affects the right ventricle, up to 20% of adults with repaired TOF exhibit late left ventricular (LV) dysfunction, which is an important risk factor for adverse clinical outcomes. Impaired LV myocardial strain and wall motion are potential etiologies for LV dysfunction after TOF repair, as are factors more traditionally associated with right ventricular (RV) impairment, such as pulmonary regurgitation (PR) and RV dilatation. Although a handful of studies have considered these factors in the context of LV dysfunction, their results have been variable, and most were focused on older, symptomatic populations. As with other late complications in these patients, it seems likely that LV dysfunction follows a progressive pathophysiology, with its origins in the decades before clinical manifestation. The early detection of LV dysfunction in patients with TOF could have an important impact on patient follow-up and clinical care. However, few studies have focused on LV myocardial performance after TOF repair, and the etiology and mechanisms of LV dysfunction remain poorly understood. In particular, little is known about LV dysfunction in the younger age group. Likewise, there are scant data regarding the possible association of impaired LV mechanics with exercise intolerance in children and adolescents after TOF repair.
Accordingly, the aim of this study was to investigate LV myocardial strain and mechanical dyssynchrony in asymptomatic children and adolescents who had undergone contemporary surgical repair of TOF. As a secondary objective, we investigated the association of LV mechanics with recognized risk factors for worse clinical outcomes, such as PR, RV dilatation, QRS duration prolongation, and exercise intolerance. We hypothesized that impaired LV myocardial mechanics are related to these adverse parameters.
Methods
Study Population
We prospectively recruited asymptomatic children and adolescents (aged 0–18 years) after TOF repair who were scheduled for elective outpatient echocardiography. Patients with residual intracardiac shunts, pulmonary atresia, or identified ventricular arrhythmias and those with implanted pacemakers were excluded. Clinical data, including demographics, age at surgery, type of RV outflow reconstruction, and whether a ventriculotomy was performed, were obtained from the medical records. LV strain analysis, the primary objective of this study, was performed in all patients. The association between RV volumes and LV strain was assessed in the subset of patients who underwent magnetic resonance imaging (MRI). A group of healthy subjects were recruited as controls for LV myocardial mechanical analysis. Control subjects were either healthy volunteers or children undergoing echocardiography for the evaluation of innocent murmurs. They otherwise had normal cardiovascular histories and normal results on physical examination and echocardiography. Informed consent was obtained from subjects or their guardians. The study was approved by the institutional research ethics board.
Echocardiography
Echocardiography was performed using a Vivid 7 ultrasound system (GE Healthcare, Wauwatosa, WI) using probe frequencies appropriate for patient size. Images were optimized for gain, compression, depth, and sector width and acquired at frame rates of 60 to 90 frames/sec. Apical four-chamber, three-chamber (long-axis), and two-chamber views and parasternal short-axis views at the basal, mid, and apical ventricular levels were acquired. Images were acquired during quiet respiration, as breath holding is not consistently feasible in children.
Strain Analysis
Digital echocardiographic images were transferred to a dedicated workstation for offline analysis (EchoPAC version 6.0.1; GE Vingmed Ultrasound AS, Horten, Norway). Strain analysis was performed by a single observer blinded to the clinical data. Strain and strain rate were measured using two-dimensional (2D) speckle-tracking echocardiography. The LV endocardium was manually traced, and the region of interest was manually adjusted to the LV wall thickness. The software tracks myocardial motion through the cardiac cycle, calculating strain from echogenic speckles in the B-mode image. Adequate tracking was visually assessed, and strain curves were accepted only if tracking appeared adequate. Longitudinal strain was measured at the basal and mid third of the lateral LV wall and septum. Radial and circumferential strain and strain rate were measured at 18 basal, mid (papillary muscle level), and apical LV segments (six segments at each level). In patients with TOF, the anteroseptal segment was excluded because of the presence of a ventricular septal defect patch in that region. Examples of strain curves in patients with TOF and controls are shown in Figures 1 to 3 .



Measurement of LV Dyssynchrony
LV longitudinal mechanical dyssynchrony was measured as the absolute difference in time to peak longitudinal strain between the basal septum and basal LV lateral wall. LV radial mechanical dyssynchrony was measured by the septal-posterior wall delay as well as by the standard deviation of time to peak radial strain in six segments at the midventricular level. LV circumferential mechanical dyssynchrony was measured by the standard deviation of time to peak circumferential strain in six segments at the midventricular level. The midventricular level was chosen for radial and circumferential dyssynchrony analysis to avoid the ventricular septal defect patch. Mechanical dyssynchrony measurements were corrected for heart rate (index/R-R interval × 1,000).
Metabolic Exercise Testing
Peak oxygen uptake (V o 2 ) and calculated percentage of predicted peak V o 2 were recorded from a metabolic exercise test and were available in 81% of patients with TOF, performed at a median interval of 0 days from echocardiography.
MRI
MRI data were recorded when these had been obtained for clinical purposes (at the cardiologist’s discretion). LV and RV volumes, ejection fraction (EF), and PR flow indexed for body surface area were recorded. In patients who had not undergone MRI, LV EFs were calculated from M-mode echocardiographic images (using the Teicholz formula). MRI was performed on a 1.5-T scanner (Avanto; Siemens Healthcare, Erlangen, Germany). For ventricular volumetry, a short-axis cine stack was acquired during breath-hold, using a steady-state free precession gradient-echo sequence with minimum echo and repetition times, a flip angle of 70°, bandwidth of 89 kHz, number of excitations of 1, views (lines) per segment to allow 20 true reconstructed phases per cardiac cycle, slice thicknesses 5 of 7 mm, number of slices of 10 to 12, gap adjusted to cover both ventricles, and in-plane spatial resolution of 1.5 to 2.5 mm. For pulmonary flow volumes, phase-contrast velocity mapping was performed perpendicular to the right and left pulmonary arteries, with the following parameters: minimal repetition time and echo time; flip angle, 30°; bandwidth, 50 kHz; views per segment, 1 or 2, adequate to achieve 25 true phases per cardiac cycle; free breathing; number of excitations, 2; velocity encoding range, ± 150 cm/sec; slice thickness, 5 mm; and in-plane resolution, 1.5 to 2.5 mm. A dedicated workstation (Mass Analysis and CV Flow; Medis Medical Imaging Systems, Leiden, The Netherlands) was used for volumetric and flow analysis. For total pulmonary blood flow and regurgitation volumes, right and left pulmonary artery net forward flow volumes indexed to body surface area and reverse flow volumes indexed to body surface area, respectively, were added. Quantifying PR by volumes indexed to body surface area, rather than by regurgitant fraction, provides a more accurate assessment of PR severity, because PR volume, rather than fraction, affects RV response.
Intraobserver and Interobserver Reliability Analysis
Intraobserver and interobserver reliability analysis of peak LV radial, circumferential and longitudinal strain, RV longitudinal strain, and time to peak strain of each of these measures was performed in 10 randomly chosen patients with TOF. Curves were traced anew, and strain curves generated anew, on the same cardiac cycle by the same observer and independently by a second observer for intraobserver and interobserver reliability, respectively. Bland-Altman limits of agreement analysis was used for intraobserver and interobserver reliability.
Statistical Analysis
LV strain and mechanical dyssynchrony data were compared between patients with TOF and controls using nonpaired two-tailed Student’s t tests. Associations between LV mechanics and PR, RV end-diastolic volume, QRS duration, and exercise capacity were investigated using univariate linear regression models. Parameter estimates and standard errors are reported. Parameter estimates represent the change in the dependent variable (e.g., V o 2 ) for each increase of 1 unit in the independent variable (e.g., LV strain) or for the presence (vs absence) of the independent variable for binary variables. This informs as to the direction of the association (positive or negative) and its strength (how much change was associated with each unit increase in the independent variable). Statistical analyses used SAS version 9.2 (SAS Institute Inc., Cary, NC). P values < .05 were considered statistically significant.
Results
Study Population
One-hundred twenty-four subjects, including 53 patients with TOF (mean age, 12 ± 3 years) and 71 healthy controls (mean age, 10 ± 5 years), were studied. Characteristics of the TOF population are summarized in Table 1 . All patients were in sinus rhythm. Twenty-eight patients (53%) were repaired using transannular outflow tract patches, 15 (28%) with infundibular patches, two (4%) with infundibulectomy plus valvotomy, and two (4%) with the placement of an external RV–pulmonary artery conduit. Information about surgical procedures was unavailable in six patients. The mean RV outflow gradient in patients with TOF was 25 mm Hg (range, 8–77 mm Hg). Eight patients (15%) had undergone second procedures for pulmonary valve replacement. MRI data were available in 64% of patients with TOF, performed at a median interval of 14 days (range, 0–261 days) from echocardiography.
Variable | n | Value |
---|---|---|
Age (y) | 53 | 12 ± 3 (6–18) |
Male gender | 53 | 57% |
Body surface area (m 2 ) | 51 | 1.36 ± 0.37 (0.78–2.23) |
Age at operation (mo) | 50 | 17 ± 16 (1–84) |
Previous shunt surgery | 47 | 13% |
Ventriculotomy | 47 | 51% |
Heart rate (beats/min) | 53 | 70 ± 14 |
QRS duration (msec) | 53 | 141 ± 23 (82–202) |
Peak V o 2 (mL/kg/min) | 42 | 29 ± 7 (14.8–43.6) |
Predicted peak V o 2 (%) | 42 | 66 ± 15 (32–93) |
Indexed PR flow (mL/min/m 2 ) | 33 | 2.05 ± 1.00 (0.1–4.7) |
RV end-diastolic volume index (mL/m 2 ) | 36 | 157 ± 39 (74.1–252.9) |
RV EF (%) | 36 | 49.0 ± 8.8 (28.3–73.1) |
LV end-diastolic volume index (mL/m 2 ) | 36 | 82.5 ± 15.9 (40.8–117.0) |
LV end-systolic volume index (mL/m 2 ) | 36 | 35.7 ± 9.4 (21.1–64.0) |
LV ejection fraction (%) | 53 | 59.9 ± 6.7 (45–77) |
LV Function and Strain
LV EFs were ≥55% in 43 patients (81%). Ten patients had LV EFs < 55%; eight of these had LV EFs between 49% and 54%. In two patients, LV EFs were 45% and 47%.
Results for regional longitudinal, radial, and circumferential strain and strain rate are shown in Tables 2 to 4 . Longitudinal septal peak longitudinal strain was reduced in patients with TOF compared with controls (−18.5 ± 3.5% vs −20.2 ± 2.8%, P = .01), but LV lateral wall longitudinal strain was not different between the groups (−16 ± 5% vs −17.5 ± 4.7%, P = .22) ( Table 2 ). Regional circumferential and radial LV strain were significantly reduced in patients with TOF compared with controls ( Tables 3 and 4 ).
Segment ∗ | Patients with TOF | Controls | P |
---|---|---|---|
Strain (%) | |||
Lateral | −16.3 ± 5.6 | −17.5 ± 4.7 | .22 |
Septal | −18.5 ± 3.5 | −20.2 ± 2.8 | .007 |
Anteroseptal | — | −19.6 ± 3.4 | — |
Posterior | −16.8 ± 5.0 | −17.8 ± 5.1 | .36 |
Anterior | −19.1 ± 6.5 | −20.8 ± 4.5 | .17 |
Inferior | −19.8 ± 5.4 | −21.1 ± 5.0 | .21 |
Strain rate (sec −1 ) | |||
Lateral | −1.2 ± 0.5 | −1.4 ± 0.3 | .11 |
Septal | −1.1 ± 0.3 | −1.2 ± 0.2 | .10 |
Anteroseptal | — | −1.2 ± 0.3 | — |
Posterior | −1.0 ± 0.9 | −1.3 ± 0.4 | .06 |
Anterior | −1.3 ± 0.6 | −1.3 ± 0.4 | .60 |
Inferior | −1.4 ± 0.4 | −1.5 ± 0.9 | .63 |
∗ All values are the average of the basal and mid wall segments.
Segment | Patients with TOF | Controls | P |
---|---|---|---|
Strain (%) | |||
Basal | |||
Anterior septum | — | 34.0 ± 15.0 | — |
Anterior | 28.0 ± 14.0 | 39.0 ± 17.0 | .001 |
Lateral | 29.0 ± 16.0 | 45.0 ± 19.0 | <.001 |
Posterior | 32.0 ± 18.0 | 48.0 ± 21.0 | <.001 |
Inferior | 34.0 ± 16.0 | 46.0 ± 20.0 | <.001 |
Inferior septum | 34.0 ± 14.0 | 40.0 ± 17.0 | .04 |
Mid | |||
Anterior septum | 36.0 ± 14.0 | 44.0 ± 20.0 | .02 |
Anterior | 36.0 ± 19.0 | 51.0 ± 22.0 | <.001 |
Lateral | 37.0 ± 20.0 | 56.0 ± 21.0 | <.001 |
Posterior | 38.0 ± 17.0 | 57.0 ± 22.0 | <.001 |
Inferior | 38.0 ± 14.0 | 52.0 ± 21.0 | <.001 |
Inferior septum | 37.0 ± 13.0 | 45.0 ± 20.0 | .01 |
Apical | |||
Anterior septum | 33.0 ± 17.0 | 47.0 ± 22.0 | .002 |
Anterior | 40.0 ± 23.0 | 53.0 ± 18.0 | .005 |
Lateral | 43.0 ± 23.0 | 59.0 ± 19.0 | <.001 |
Posterior | 41.0 ± 20.0 | 58.0 ± 20.0 | <.001 |
Inferior | 38.0 ± 18.0 | 52.0 ± 20.0 | <.001 |
Inferior septum | 35.0 ± 16.0 | 46.0 ± 20.0 | .003 |
Strain rate (sec −1 ) | |||
Basal | |||
Anterior septum | — | 1.6 ± 0.5 | — |
Anterior | 1.4 ± 0.7 | 1.7 ± 0.5 | .004 |
Lateral | 1.5 ± 0.9 | 1.9 ± 0.8 | .01 |
Posterior | 1.6 ± 1.0 | 2.0 ± 0.8 | .02 |
Inferior | 1.6 ± 0.8 | 2.1 ± 0.8 | .007 |
Inferior septum | 1.5 ± 0.5 | 1.9 ± 0.8 | .004 |
Mid | |||
Anterior septum | 1.4 ± 0.4 | 1.6 ± 0.4 | .004 |
Anterior | 1.4 ± 0.5 | 1.9 ± 0.8 | <.001 |
Lateral | 1.4 ± 0.4 | 1.9 ± 0.7 | <.001 |
Posterior | 1.5 ± 0.4 | 1.9 ± 0.5 | <.001 |
Inferior | 1.5 ± 0.4 | 1.9 ± 0.5 | <.001 |
Inferior septum | 1.5 ± 0.4 | 1.9 ± 0.5 | <.001 |
Apical | |||
Anterior septum | 1.4 ± 0.6 | 1.8 ± 0.9 | .01 |
Anterior | 1.8 ± 0.9 | 1.9 ± 0.6 | .46 |
Lateral | 1.7 ± 0.8 | 2.0 ± 0.7 | .06 |
Posterior | 1.6 ± 0.7 | 1.9 ± 0.6 | .10 |
Inferior | 1.5 ± 0.8 | 1.8 ± 0.6 | .08 |
Inferior septum | 1.5 ± 0.7 | 1.8 ± 0.6 | .03 |
Segment | Patients with TOF | Controls | P |
---|---|---|---|
Strain (%) | |||
Basal | |||
Anterior septum | — | −23.0 ± 6.0 | — |
Anterior | −18.0 ± 8.0 | −18.0 ± 7.0 | .87 |
Lateral | −15.0 ± 7.0 | −19.0 ± 7.0 | .02 |
Posterior | −14.0 ± 7.0 | −16.0 ± 7.0 | .10 |
Inferior | −11.0 ± 7.0 | −16.0 ± 6.0 | <.001 |
Inferior septum | −18.0 ± 8.0 | −19.0 ± 11.0 | .53 |
Mid | |||
Anterior septum | −24.0 ± 6.0 | −22.0 ± 7.0 | .22 |
Anterior | −18.0 ± 6.0 | −20.0 ± 6.0 | .10 |
Lateral | −16.0 ± 7.0 | −19.0 ± 6.0 | .02 |
Posterior | −11.0 ± 7.0 | −14.0 ± 7.0 | .12 |
Inferior | −12.0 ± 5.0 | −16.0 ± 6.0 | <.001 |
Inferior septum | −20.0 ± 5.0 | −23.0 ± 6.0 | .04 |
Apical | |||
Anterior septum | −22.0 ± 6.0 | −21.0 ± 6.0 | .4 |
Anterior | −20.0 ± 7.0 | −21.0 ± 7.0 | .56 |
Lateral | −18.0 ± 7.0 | −16.0 ± 5.0 | .10 |
Posterior | −15.0 ± 8.0 | −13.0 ± 7.0 | .23 |
Inferior | −18.0 ± 6.0 | −18.0 ± 6.0 | .95 |
Inferior septum | −22.0 ± 5.0 | −22.0 ± 6.0 | .88 |
Strain rate (sec −1 ) | |||
Basal | |||
Anterior septum | — | −1.7 ± 0.5 | — |
Anterior | −1.4 ± 0.5 | −1.8 ± 0.6 | .002 |
Lateral | −1.5 ± 0.8 | −1.7 ± 0.5 | .19 |
Posterior | −1.6 ± 0.8 | −1.8 ± 0.7 | .19 |
Inferior | −1.4 ± 0.6 | −1.6 ± 0.6 | .10 |
Inferior septum | −1.4 ± 0.4 | −1.7 ± 0.6 | .006 |
Mid | |||
Anterior septum | −1.4 ± 0.4 | −1.5 ± 0.5 | .07 |
Anterior | −1.3 ± 0.4 | −1.6 ± 0.5 | .02 |
Lateral | −1.2 ± 0.5 | −1.6 ± 0.5 | <.001 |
Posterior | −1.1 ± 0.5 | −1.3 ± 0.7 | .11 |
Inferior | −1.1 ± 0.5 | −1.5 ± 0.4 | .002 |
Inferior septum | −1.3 ± 0.5 | −1.6 ± 0.4 | <.001 |
Apical | |||
Anterior septum | −1.5 ± 0.5 | −1.6 ± 0.6 | .37 |
Anterior | −1.7 ± 0.6 | −1.8 ± 0.6 | .43 |
Lateral | −1.5 ± 0.6 | −1.5 ± 0.5 | .94 |
Posterior | −1.2 ± 0.5 | −1.4 ± 0.7 | .10 |
Inferior | −1.2 ± 0.6 | −1.5 ± 0.8 | .08 |
Inferior septum | −1.4 ± 0.4 | −1.5 ± 0.4 | .10 |
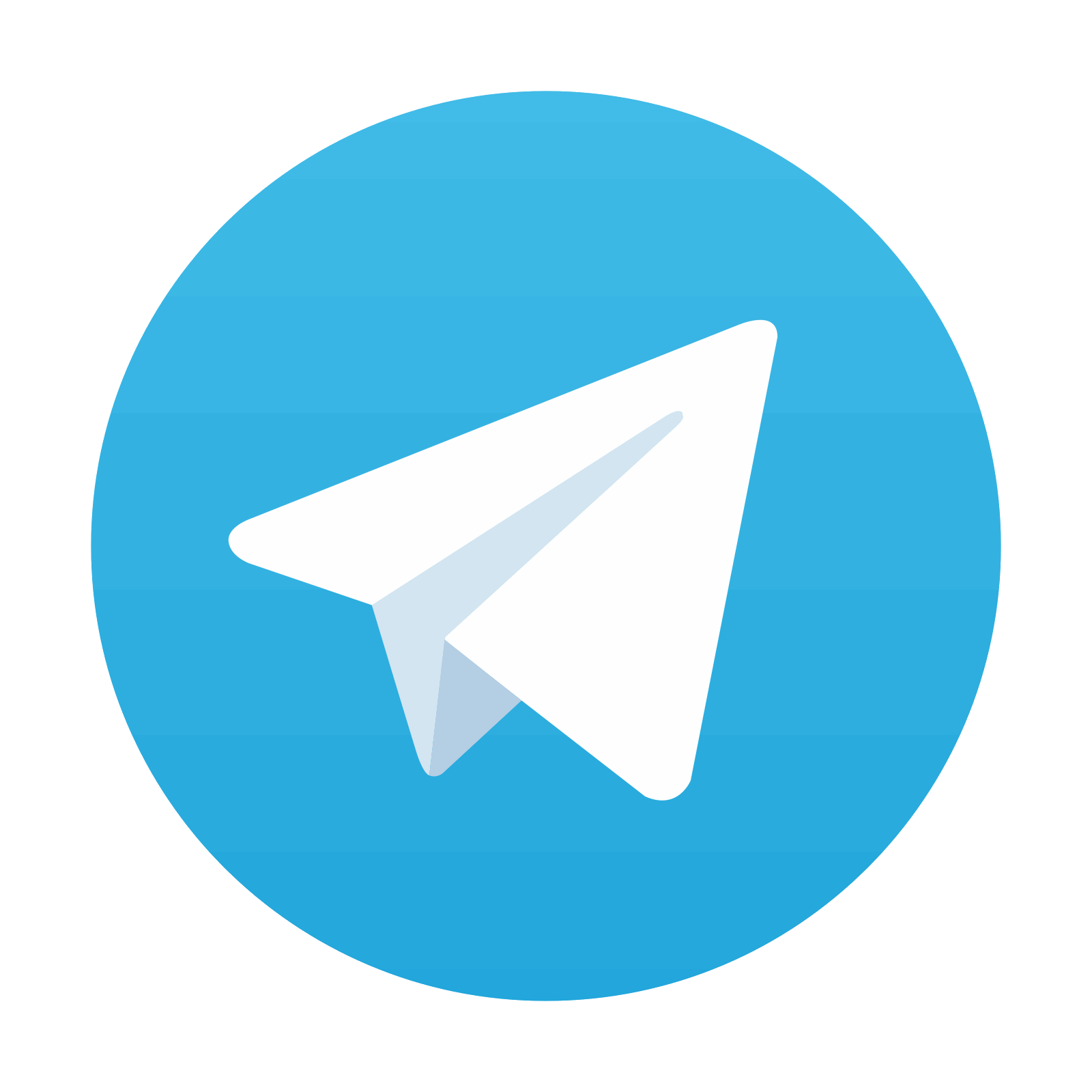
Stay updated, free articles. Join our Telegram channel

Full access? Get Clinical Tree
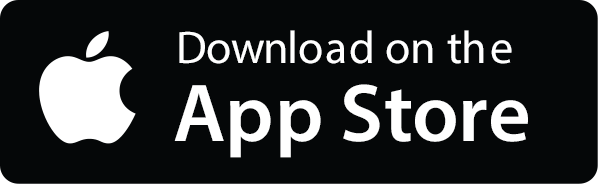
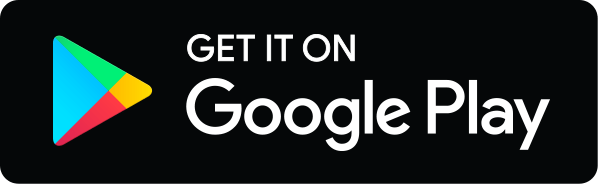
