Background
Patients with hypertrophic cardiomyopathy (HCM) present unusual myocardial mechanics. The aim of this study was to assess the impact of hypertrophy on global and regional two-dimensional (2D) strain derived from both tomographic images (2D/2D) and volumetric image acquisition (2D/three-dimensional [3D]) in patients with HCM compared with control subjects.
Methods
Comprehensive resting 2D and 3D echocardiography was performed in 40 patients with HCM and in 53 control subjects, with comparable distributions of age, gender, and left ventricular (LV) ejection fraction. LV global and segmental measurements of all 2D/2D and 2D/3D peak strain components (global and segmental longitudinal strain, global and segmental circumferential strain, global and segmental radial strain, and global and segmental area strain) and 3D indexed LV end-diastolic myocardial mass were obtained from all patients. LV wall thickness was assessed in short-axis views and classified in four quartiles (<10.5, 10.5–13.0, 13.0–16.5, and >16.5 mm).
Results
The reproducibility of 2D/3D strain was similar or greater and more consistent for all components compared with 2D/2D strain analysis. There was a significant correlation between 3D LV end-diastolic mass and all 2D/3D strain components ( P < .05). Two-dimensional/3D global circumferential strain had the strongest association with 3D LV ejection fraction ( r = 0.50, P = .001). For segmental deformation, patients with HCM had lower longitudinal deformation whatever the LV wall thickness, whereas circumferential function was increased in nonhypertrophied and poorly hypertrophied segments compared with control subjects.
Conclusions
Two-dimensional/3D strain is a reliable technique to assess myocardial deformation. Myocardial mass is related to 2D/3D strain components in patients with HCM. Circumferential deformation, compared with longitudinal deformation, seems to be the main component of the maintenance of systolic function in HCM.
With an approximate prevalence of 0.2%, hypertrophic cardiomyopathy (HCM) is the most frequent genetic cardiomyopathy. This pathology is characterized by a myocardial asymmetric or concentric hypertrophy associated with myocardial fiber disarray and fibrosis, leading to global and regional variability and heterogeneity of systolic and diastolic deformation. As mentioned in a recent guideline document, “a better assessment of underlying pathophysiology and anatomy is crucial to improve the management of HCM patients.” Therefore, assessment of systolic deformation by either magnetic resonance imaging or echocardiography has been extensively studied ( Figure 1 A–1C). Impairment of the global and regional longitudinal deformation of the myocardium is well recognized in this population. However, there are some discrepancies in the literature regarding other deformation parameters, such as circumferential strain. Assessment of deformation in the three directions with two-dimensional (2D) speckle-tracking can be difficult because of out-of-plane motion, left ventricular (LV) foreshortening, changes in heart rate and loading conditions. Furthermore, acquisitions of the 6 required LV views can be time consuming. Moreover, matching different segments between apical and short-axis views may be challenging. Two-dimensional strain calculated from three-dimensional (3D) echocardiographic acquisitions (2D/3D) has emerged as a potential solution, allowing measurement of all strain components in all LV segments from a single acquisition, thereby also addressing the difficulty of matching different segments ( Figure 1 D–1G). The potential interest of 3D echocardiography has been suggested in some recent studies in patients with HCM, but further investigation is needed to confirm the feasibility and reliability of this approach.

The primary aim of our study was to compare patients with HCM and control subjects regarding classical echocardiographic parameters and 2D- and 3D-derived deformation parameters and to determine the impact of global myocardial mass and regional thickness on all 2D/3D global and regional strain components. The secondary aim of our study was to investigate the feasibility and the reliability of 2D/3D strain analysis and to determine if deformation parameters may be related to systolic function and/or clinical events in patients with HCM.
Methods
The study group consisted of 40 patients with HCM diagnosed using previous validated criteria (LV hypertrophy with LV wall thickness ≥ 15 mm for isolated cases and ≥13 mm for familial screening, with asymmetric distribution associated with a nondilated and hyperdynamic chamber in the absence of another cardiac or systemic disease [e.g., hypertension or aortic stenosis] associated with preserved LV ejection fraction [LVEF] [>50% assessed both by 2D and 3D methods ]), who were referred to our university center from January 2008 to January 2013. All patients underwent comprehensive 2D and 3D resting echocardiography as part as routine evaluation and therefore were prospectively included. The distribution of hypertrophy in patients with HCM was defined as described by Maron et al. : focal, two or fewer hypertrophied segments; intermediate, three to seven hypertrophied segments; and diffuse, more than seven segments. A control group composed of 53 patients with normal clinical and echocardiographic findings and no family histories of HCM, who were included in a prospective survey approved by the institutional review board, underwent the same echocardiographic protocol. All patients with HCM gave their oral consent to use their data in this study.
Echocardiographic Imaging and Analysis
Echocardiography was performed with commercially available standard ultrasound scanners (Vivid 7 and Vivid 9; GE Vingmed Ultrasound AS, Horten, Norway) with a 2.5-MHz transducer and a 3D probe (4V or 4D Volume Phased Array Probe).
Two-dimensional echocardiographic images of the left ventricle were obtained from the parasternal long-axis and short-axis views at the basal, median, and apical levels and from the three standard LV apical views (four, two, and three chambers). From the apical position, a full LV volume was acquired in a wide-angled acquisition “full-volume” mode. In this mode, a number of wedge-shaped subvolumes (four to six) are acquired over consecutive cardiac cycles during a single breath-hold and stitched together to create one pyramidal volume sample. All images were acquired at frame rates of ≥60 frames/sec for 2D imaging and 25 volumes/sec for 3D imaging.
Two-Dimensional Echocardiographic Parameter Acquisition and Analysis
Classical 2D echocardiographic parameters were measured: interventricular septal and posterior wall thicknesses and end-diastolic and end-systolic LV diameters were measured from the parasternal long-axis view, and LVEF was calculated by using the biplane Simpson method from the apical four- and two-chamber views. Left atrial (LA) and right atrial areas were measured from the apical four-chamber view. Mitral inflow velocities of the E wave, the A wave, and the deceleration time of the E wave were assessed by Doppler imaging. Septal and lateral e′ velocities at the level of the mitral annulus were recorded with Doppler tissue imaging from the apical four-chamber view.
Maximal end-diastolic LV wall thickness was measured from the 2D short-axis view for each segment according to a 16-segment model.
All echocardiographic parameters were measured in accordance with the latest European guidelines.
Three-Dimensional Image Acquisition and Analysis
Three-dimensional echocardiographic images were recorded from the apical position in a wide-angled “full-volume” acquisition mode. Special care was taken to include the entire LV cavity within the pyramidal 3D volume and to adjust width to improve the temporal and spatial resolution.
The software (4D Auto LVQ; GE Healthcare, Little Chalfont, United Kingdom) automatically displays three apical and three short-axis views. Endocardial and epicardial surfaces are automatically detected with manual adjustments possible when necessary. The following 3D standard parameters are displayed: 3D LV end-diastolic and end-systolic volumes, LVEF, and LV end-diastolic mass.
The system then performs the wall deformation analysis and calculates 2D strain from 2D projections of the 3D images (slice thickness is determined automatically by the software, and the default value was used) and provides both the 2D/3D LV global peak value of each strain component (global longitudinal strain [GLS], global area strain, global radial strain, and global circumferential strain [GCS]) and segmental values (segmental longitudinal strain [SLS], segmental area strain, segmental radial strain [SRS], and segmental circumferential strain).
Clinical End Points
Clinical follow-up was performed. Cardiovascular events were defined as hospitalization for heart failure, atrial fibrillation, and/or sustained ventricular arrhythmias.
Statistical Analysis
Continuous variables are expressed as mean ± SD and categorical variables as numbers and percentages. One-way analysis of variance was performed to determine global P value for the comparison between quartiles. Data comparisons between quartiles and between groups were performed using Student’s unpaired t tests, χ 2 tests, or Fisher exact tests as appropriate. The relationships between LV E/e′ ratio and other continuous variables were evaluated by using simple linear regression. P values < .05 were considered statistically significant. The intra- and interobserver variability of all 2D/2D segmental strain and all 2D/3D segmental strain methods was analyzed by using Bland-Altman analysis (segmental analysis of 85 segments from five patients with HCM). All statistical analyses were performed with JMP version 10.0.2 (SAS Institute Inc, Cary, NC).
Results
Demographic and Clinical Data at Inclusion and after Follow-Up for Patients wtih HCM
When classified according to Maron et al. , our HCM population included 10 patients with focal hypertrophy, 17 patients with intermediate hypertrophy, and 13 patients with diffuse hypertrophy. Seventeen patients had subaortic gradients (mean, 61 ± 36 mm Hg) at rest.
In addition, 11 patients had isolated septal hypertrophy, 23 patients had septal hypertrophy associated with hypertrophy of another wall (11 anterior, five inferior, and seven lateral), and six demonstrated other patterns of hypertrophy (either isolated inferior wall hypertrophy or very diffuse hypertrophy).
Thirty patients were treated with β-blockers at the time of examination. None of the patients with HCM had previous coronary disease (exclusion by bicycle exercise testing with or without myocardial scintigraphy).
Comparison between Patients with HCM and Control Subjects for General Characteristics and 2D and 3D Echocardiographic Parameters
There were no differences between the groups regarding age, gender, 2D LVEF, and heart rate ( Table 1 ). Patients with HCM had significantly different mitral inflow velocities, with higher E waves (0.84 ± 0.36 vs 0.72 ± 0.13 m/sec, P = .03) but also longer E-wave deceleration times (234 ± 91 vs 196 ± 46 ms, P = .01) than control subjects. There was a significantly lower mean LV e′ velocity (0.06 ± 0.001 vs 0.11 ± 0.02 m/sec, P < .001), associated with higher mean LV E/e′ ratio (15.0 ± 9.1 vs 6.8 ± 1.4, P < .0001), in patients with HCM. Both LA and right atrial areas were greater in the HCM group, and transtricuspid gradient was higher. Mean LV E/e′ ratio was significantly associated with transtricuspid gradient and LA area (respectively, r 2 = 0.46, P = .0001, and r 2 = 0.30, P = .004).
Variable | Control group ( n = 53) | HCM group ( n = 40) | P |
---|---|---|---|
Age (y) | 56 ± 12 | 55 ± 13 | .70 |
Women | 29 (55%) | 21 (52%) | .00 |
Heart rate (beats/min) | 64 ± 9 | 62 ± 13 | .38 |
IVSd (mm) | 1 ± 0.25 | 1.96 ± 0.70 | <.0001 |
LV PWd (mm) | 0.9 ± 0.15 | 1.24 ± 0.32 | <.0001 |
IVSd/LV PWd | 1.1 ± 0.2 | 1.68 ± 0.70 | <.0001 |
2D LVEF (%) | 65 ± 5 | 65 ± 7 | 1.00 |
LV E velocity (m/sec) | 0.72 ± 0.13 | 0.84 ± 0.36 | .03 |
LV E deceleration time (msec) | 196 ± 46 | 234 ± 91 | .01 |
A velocity (m/sec) | 0.64 ± 0.13 | 0.71 ± 0.27 | .10 |
E/A ratio | 1.17 ± 0.38 | 1.34 ± 0.66 | .12 |
Lateral LV e′ velocity (m/sec) | 0.13 ± 0.03 | 0.07 ± 0.02 | <.0001 |
Septal LV e′ velocity (m/sec) | 0.10 ± 0.02 | 0.06 ± 0.02 | <.0001 |
Mean LV e′ velocity (m/sec) | 0.11 ± 0.02 | 0.06 ± 0.01 | <.0001 |
Lateral LV E/e′ ratio | 6.3 ± 1.4 | 14.3 ± 8.4 | <.0001 |
Septal LV E/e′ ratio | 7.8 ± 1.7 | 15.6 ± 10.5 | <.0001 |
Mean LV E/e′ ratio | 6.8 ± 1.4 | 15.0 ± 9.10 | <.0001 |
LA area (cm 2 ) | 15.5 ± 3.8 | 25.4 ± 5.9 | <.0001 |
TTG (mm Hg) | 19.6 ± 4.2 | 29.8 ± 11.3 | <.0001 |
RA area (cm 2 ) | 13.4 ± 4.7 | 16.0 ± 4.2 | .007 |
There were no differences between the groups for 3D LVEF, 3D LV end-systolic volume, and 3D LV end-diastolic volume ( Table 2 ). Indexed 3D LV end-diastolic mass was increased in patients with HCM, with a mean value of 110 g/m 2 . All 3D global strain absolute values were lower in patients with HCM than in control subjects, except for 3D GCS, which was similar in the two groups.
Variable | Control group ( n = 53) | HCM group ( n = 40) | P |
---|---|---|---|
3D LVEF (%) | 63 ± 4 | 64 ± 8 | .43 |
Indexed 3D LV end-diastolic mass (g/m 2 ) | 71 ± 8 | 110 ± 30 | <.0001 |
3D LVESV (mL) | 36 ± 12 | 34 ± 16 | .49 |
3D LVEDV (mL) | 97 ± 28 | 94 ± 33 | .63 |
3D GLS (%) | −19.9 ± 2.5 | −13.7 ± 3.6 | <.0001 |
3D GAS (%) | −34.5 ± 3.3 | −27.6 ± 5.1 | <.0001 |
3D GRS (%) | 53.3 ± 10.3 | 44.3 ± 11.6 | .0002 |
3D GCS (%) | −18.4 ± 3.2 | −18.6 ± 3.3 | .77 |
Impact of Myocardial Mass on 2D/3D Global Strain
Three-dimensional global strain values were all significantly but moderately correlated with indexed LV end-diastolic mass ( Figure 2 ). The weakest association was found with 3D GCS ( r = 0.63, P = .001; Figure 2 D).
Impact of Segmental Myocardial Thickness on 2D/2D Segmental Strain
Compared with control subjects, there was a continuous decrease in all systolic deformation from the second to the fourth quartile of wall thickness, except for 2D/2D SRS, which decreased between the third and fourth quartiles ( Figure 3 A–3C).
For longitudinal deformation, all quartiles had lower longitudinal deformation compared with control subjects ( P < .05 for all; Figure 3 A). For circumferential deformation, the first quartile had higher circumferential deformation compared with control subjects ( P = .05), there was no difference for the second quartile, and circumferential function was lower in the third and fourth quartiles ( P < .05 for both; Figure 3 B). For radial deformation, only the third and fourth quartiles demonstrated lower radial deformation compared with control subjects ( Figure 3 C).
Impact of Segmental Myocardial Thickness on 2D/3D Segmental Strain
All absolute values of strain decreased from the second to the fourth quartile of myocardial thickness ( Figure 4 A–4D).
Compared with control subjects, 3D SLS and 3D segmental area strain were decreased in all quartiles ( P < .05; Figure 4 A and 4B). There was no significant difference in 3D SRS for the first quartile and lower values from the second to the fourth quartile ( P < .05; Figure 4 D) and 3D segmental circumferential strain was higher in no or poorly hypertrophied segments (first and second quartiles), similar in the third quartile, and lower in the fourth quartile ( P < .05; Figure 4 A).
Comparison of 2D/2D Strain Analysis and 2D/3D Analysis
There was a correlation between segmental 2D/2D and 2D/3D strain values. For longitudinal deformation, this correlation was quite good ( r = 0.67, P < .0001), though it was moderate to poor for circumferential and radial deformation (respectively, r = 0.60, P < .0001, and r = 0.32, P < .0001).
The feasibility of both 2D/2D and 2D/3D SLS was good (respectively, 83% and 81%). Tracking of myocardial segments deformation was better fo 2D/3D strain than for 2D/2D strain (respectively, 81% and 62%).
Compared with 2D/2D strain analysis, the 2D/3D method had similar or greater reproducibility for all strain analysis ( Table 3 ).
Variable | Intra observer reproducibility | Inter observer reproducibility | ||||
---|---|---|---|---|---|---|
R | Mean ± SD | Variability (%) | R | Mean ± SD of differences | Variability (%) | |
2D/2D SLS | 0.87 | 0.9 ± 3.6 | 25.2 | 0.70 | −0.65 ± 4.27 | 26 |
2D/2D SCS | 0.85 | 1.61 ± 4.61 | 35.5 | 0.72 | −2.24 ± 6.08 | 37.2 |
2D/2D SRS | 0.50 | 3.82 ± 9.9 | 31.3 | 0.39 | −0.45 ± 10.49 | 31.9 |
2D/3D SLS | 0.84 | −0.7 ± 3.7 | 25.6 | 0.84 | −0.6 ± 3.8 | 26.3 |
2D/3D SCS | 0.81 | −1.5 ± 4.2 | 21.5 | 0.82 | −1.1 ± 4.0 | 22.2 |
2D/3D SRS | 0.79 | 4.8 ± 14.5 | 30.4 | 0.82 | 3.9 ± 11.9 | 28.5 |
2D/3D SAS | 0.84 | −1.8 ± 5.5 | 20.4 | 0.81 | −1.1 ± 5.9 | 21.8 |
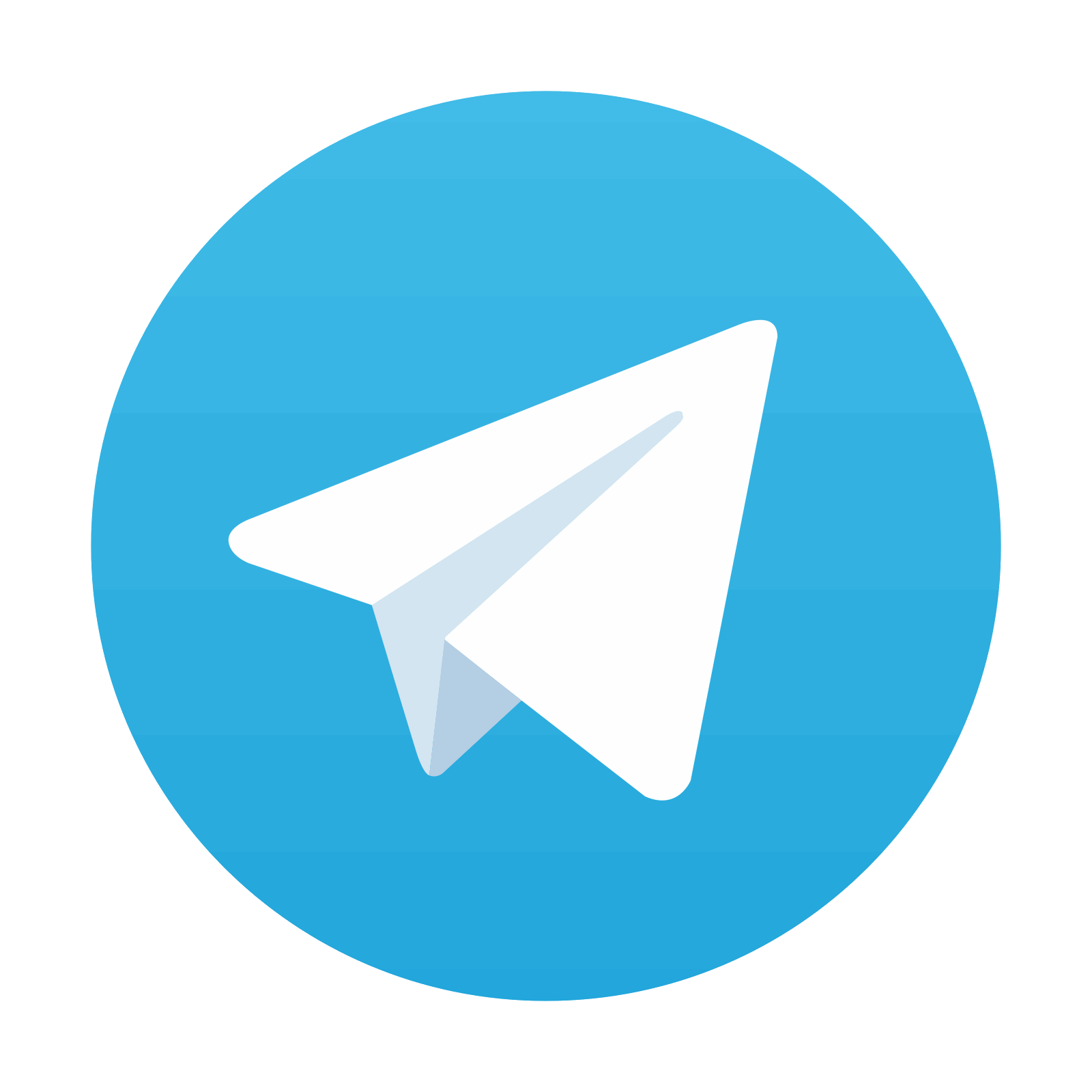
Stay updated, free articles. Join our Telegram channel

Full access? Get Clinical Tree
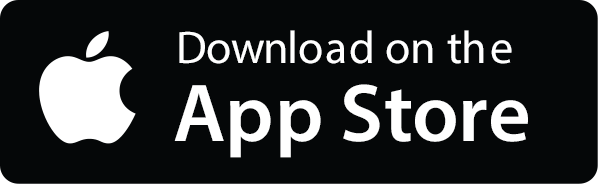
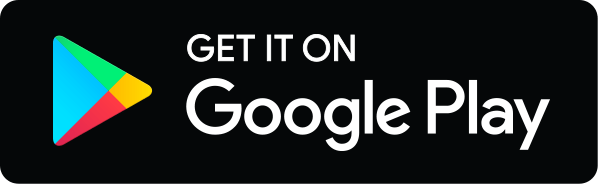
