Background
Left ventricular (LV) afterload could be associated with reduced myocardial contractility. The aim of this study was to evaluate the relative impact of increased afterload on LV myocardial deformation indices in chronic aortic constriction, with regard to hypertrophy, myocardial fibrosis, and mitochondrial function, and to differentiate acute versus chronic afterload effect.
Methods
Young pigs underwent aortic banding ( n = 11) or sham ( n = 7) operations. Nineteen weeks later, LV morphology and systolic function, including myocardial deformation, were assessed by echocardiography before and after banding release or acute aortic constriction (in the sham group). After the animals were euthanized, mitochondrial function and LV interstitial fibrosis were assessed.
Results
The chronic banding group ( n = 8) presented with significant LV hypertrophy compared with the sham group ( n = 7), and longitudinal strain (LS) was significantly altered (16.9 ± 0.7% vs 20.3 ± 0.7%, P = .001) while circumferential, radial strain, and ejection fraction were not. LS abnormalities were situated mostly on the basal and mid segments and on the septal wall. There was also significantly more myocardial fibrosis in the chronic banding group compared with the sham group, while mitochondrial function was preserved. The relative contributions of hypertrophic and fibrotic remodeling and of afterload to alter global LS were 62%, and 38%, respectively. Acute aortic banding also significantly altered LS. The ratio of LS to septal wall thickness enabled differentiation between chronic and acute afterload increase (1.9 ± 0.2 in the chronic group vs 2.9 ± 0.3 in the acute group, P = .001).
Conclusions
LS is susceptible to both hypertrophic and fibrotic remodeling and afterload increase, particularly on the basal and mid LV segments of the septum. The ratio of LS to septal wall thickness enables differentiation of acute from chronic afterload LS alteration.
Left ventricular (LV) pressure chronic overload secondary to chronic progressive moderate to severe aortic valve stenosis or systemic hypertension leads to morphologic hypertrophic remodeling of the left ventricle. It also generates impairments of longitudinal myocardial deformation, although LV ejection fraction (LVEF) may be preserved. LV hypertrophy and myocardial fibrosis are reported to be cofactors of LV deformation alteration. LV hypertrophic remodeling induces a decrease in coronary flow reserve and an increase in oxygen myocardial consumption and consequently myocardial ischemia, which results in interstitial myocardial fibrosis. Moreover, myocardial hypertrophy is considered an adaptive response to increased workload, while compelling evidence also suggests mitochondrial (dys)function to mechanistically contribute to heart failure development.
However, it has been demonstrated that an acute increase in LV afterload alone could also result in a significant reduction in LV longitudinal strain (LS), as well as circumferential strain (CS), radial strain (RS), and LVEF.
The main rationale of this study was that the relative roles of afterload, myocyte bioenergetics, LV hypertrophy, and myocardial fibrosis in global LS reduction, in the presence of chronic afterload increases, are not precisely understood. Moreover, to date, no experimental model has attempted to differentiate the effects of chronic or progressive afterload increases from those pertaining to acute afterload increases.
We hypothesized, in the event of chronic aortic stenosis, that afterload increases and hypertrophic remodeling should contribute to a higher extent to reduced LV deformation than either myocardial fibrosis or myocyte mitochondrial function, at least at an early stage. Releasing chronic afterload would allow us to evaluate the relative contribution of each factor. Moreover, in the event of acute aortic constriction, only afterload increases and, although to a lower extent, myocyte mitochondrial function are likely to be involved. Consequently, indices taking in account both deformations and LV wall thickness should be of interest and enable us to differentiate between the effects of chronic and acute afterload increases on myocardial deformation.
The main aim of this study was to evaluate the relative contribution of increased afterload on LV global and regional myocardial deformations assessed by strain in chronic aortic constriction, with regard to hypertrophic and fibrotic myocardial remodeling, as well as expected mitochondrial myocyte metabolism alterations. A debanding (afterload release) experiment was also performed to evaluate the respective participation of LV remodeling (hypertrophy and fibrosis) and impairment on residual deformation abnormalities. Furthermore, an acute aortic constriction experiment was produced to assess the effects of afterload taken out of the context of LV remodeling and to differentiate between acute and chronic afterload effects on myocardial deformations.
Methods
The study was conducted as a prospective intervention (chronic banding) versus control (sham operation) study on male piglets with a mean body weight of 13.0 ± 1.5 kg (mean age, 10.3 ± 0.7 weeks). All experiments were conducted in agreement with the National and European Research Council Guide for the care and use of laboratory animals, and the protocol used was accepted by our local committee (No. 50120208-A).
In the chronic banding group ( n = 11), we induced a progressive increase in afterload by placing a Teflon band around the supracoronary ascending aorta (initially without any constriction), which became stenosing during growth. In the control group ( n = 7), we performed sham operations.
At a median 19.0 weeks’ follow-up, animals in the two groups were compared at baseline after median thoracotomy. The study protocol is presented in Figure 1 .

Initial Surgical Procedure
The piglets were preanaesthetized with an intramuscular injection of ketamine hydrochloride and medetomidine. Propofol 1% was then given in an ear vein. The animals were intubated and ventilated in volume-controlled mode (Cato M33285; Dräger, Lübeck, Germany). During the surgical procedure, the maintenance of anesthesia was ensured by 1.5% isoflurane sufentanil. A continuous infusion of fluids (Ringer’s acetate) was administered. Surgical procedure consisted in a left lateral minithoracotomy in the fourth intercostal space. After pericardial opening and interaorticopulmonary space dissection, the ascending aorta was exposed. The circumference of the supracoronary ascending aorta was estimated by placing a suture around it. A nonstenosing Teflon band was placed and sutured around the aorta. The thoracic wall was closed in layers. Prophylactic antibiotics and analgesics were intravenously administered. Finally the animals were extubated and transported back to the farm. A sham operation was performed on the control animals. Instead of banding the aorta, the procedure consisted only of pericardial opening and then closure of the thoracic wall.
Follow-Up Protocol
After a median of 19 weeks, the animals were anesthetized and intubated per the initial procedure and cisatracurium was added. A 4-Fr fluid-filled catheter was advanced into the proximal aorta via the left carotid artery for continuous monitoring of systemic arterial pressure. An 8-Fr fluid-filled catheter was advanced into the left jugular vein for fluid infusion. An 8-Fr fluid-filled catheter was advanced into the right jugular vein, and a Swan-Ganz catheter (Swan-Ganz CCO; Edward Lifesciences, Irvine, CA) was advanced into the pulmonary artery, under echocardiographic guidance. After injection of heparin, an 8-Fr dual field micromanometer-tipped pressure-conductance catheter (Millar Ventri-Cath 510; Millar Instruments, Houston, TX) was introduced along the long axis of the left ventricle, via the right carotid artery and through the ascending aorta (and through the banding if present), with the pigtail tip in the apex of the left ventricle. The correct position was confirmed echocardiographically. The animals were then stabilized for 30 min before thoracic surgery. After midline sternotomy, the heart was suspended in a pericardial cradle and the aorta localized. After 30 min of stabilization (baseline), hemodynamic and echocardiographic recordings were digitally acquired during brief suspension of mechanical respiration (10–15 cardiac cycles). In the chronic banding group, the next step consisted to release the aorta by sectioning the Teflon banding. Hemodynamic and echocardiographic recordings were performed after 30 min of stabilization in this condition (debanding).
In the sham group, acute banding was applied by a monitored constriction of the aorta, producing hemodynamically a ≥20 mm Hg peak-to-peak LV-aortic pressure gradient. Hemodynamic and echocardiographic recordings were made after a stabilization period of 10 min, and aortic banding was then released.
At the end of the protocol, the animals were euthanized during deep anesthesia, and the heart was excised for postmortem examinations. Each heart was collected in the operating room and immediately perfused with and stored in cold cardioplegic solution at 4°C during transport and dissection. The cardioplegic solution contained 110 mmol/L NaCl, 1.2 mmol/L CaCl 2 , 16 mmol/L KCl, 16 mmol/L MgCl 2 , and 10 mmol/L NaHCO 3 , as previously described.
Hemodynamic and Echocardiographic Evaluation
In each condition (baseline, debanding, and acute banding [sham]), heart rate, systolic and diastolic blood pressures, LV maximal, minimal, and end-diastolic pressures, cardiac output (CO), dP/dt max , and dP/dt min were recorded and stored digitally (IOX; Emka Technologies, Paris, France). After midline thoracic and pericardial opening, the transducer, fixed in a saline-filled latex bag, was placed on the epicardium. The echocardiographic recordings were performed with a 4.0-MHz transducer coupled to a Vivid 7 ultrasonic echocardiographic machine (GE Vingmed Ultrasound AS, Horten, Norway).
All pigs were scanned in five standard views: parasternal long- and short-axis views and four-, two-, and three-chamber views from an apical acoustic window. The short-axis view was obtained on basal segments until the papillary muscles were no longer visible. To evaluate the severity of aortic stenosis, we made pulsed and continuous Doppler waves in the view, allowing the best alignment with the LV outflow tract and the ascending aorta. An estimation of the pressure across the banding could be calculated from a simplified form of the Bernoulli equation. All recordings were performed by the same physician, an expert in echocardiography. The zoom function was used to assess the LV outflow tract as well as the septal and lateral wall thicknesses. Pulsed-wave Doppler acquisitions were performed at the tip of the mitral leaflets and Doppler tissue imaging at the lateral and septal sides of the mitral annulus. For speckle-tracking strain analysis, short-axis and apical views were also recorded at a mean frame rate of 70 ± 10 Hz.
Echocardiographic Analyses
Offline, a second independent blinded operator, also an expert in echocardiography, performed all measurements using EchoPAC software (GE Vingmed Ultrasound AS), calculating the mean of three cardiac cycles for all parameters. LV end-diastolic septal and lateral free wall thicknesses and LV end-diastolic and end-systolic diameters were measured in two-dimensional mode at the tip of the mitral leaflets. LV mass was calculated using the recommended formula. LV end-diastolic and end-systolic volumes and LVEF were calculated using the biplane Simpson method. Mitral pulsed-wave Doppler velocities were also measured. LV filling pressures were estimated using the ratio of peak E and septal and lateral annular E′ peak velocities (mean E/E′ ratio). S′ and A′ peaks, per spectral tissue Doppler imaging, were also estimated at both the septal and lateral mitral annular side and on the free side of the right ventricle. Systolic pulmonary artery pressure was calculated. LV outflow tract diameter was precisely measured. The dimensionless aortic velocity index through the proximal ascending aorta was measured by the ratio of LV outflow tract velocity-time integral over aortic velocity-time integral. According to continuity equation, cross-sectional aortic area was calculated, and the mean transaortic pressure gradient was measured.
For two-dimensional speckle-tracking strain analysis, the endocardial markers were placed in an end-diastolic frame, and the software automatically tracked the contour over subsequent frames. Adequate tracking could be verified in real time and corrected by adjusting the region of interest or by manually correcting the contour to ensure optimal tracking. End-systolic RS and CS were quantified in the parasternal midlevel short-axis view (mean of the six midlevel segments). End-systolic LS was assessed in apical views. The average of the 17 segments’ LS was calculated from the three apical views in relation to the strain magnitude at aortic valve closure. LS and CS are expressed in absolute terms throughout the report to avoid confusion in the data interpretation. Global LS is usually considered normal in humans when <18%. The ratio of LS to septal wall thickness was also calculated.
Postmortem Examinations
For histologic analysis, two transmural 5- to 10-mm biopsies were obtained: on the basal region of the lateral wall of the left ventricle and on the basal region of the interventricular septal wall. After fixation in 10% neutral buffered formalin, the samples were paraffin embedded, cut into 7-μm sections, and stained with hematoxylin and eosin. A sirius red coloration was used to determine cardiac collagen density. The colored sections were then microscopically examined, and the percentage of interstitial fibrosis area (planimetry) over the total area was quantified on 10 samples for each animal, using the free software ImageJ version 1.49 (National Institutes of Health, Bethesda, MD), available online. The operator was blinded with respect to which sections were issued from a banding animal and which were from a sham animal.
For mitochondrial respiration analysis, two subendocardial sections of 2 to 5 mm were obtained from the basal region of the LV septal and lateral walls and from the left atrium. Mitochondrial respiration was investigated in skinned cardiac muscle fibers in which the sarcolemma was made permeable by pretreatment with saponin. All the respirometric data obtained were normalized to tissue dry weight. The apparent Michaelis constant ( K M ) of mitochondrial respiration rate for adenosine diphosphate (ADP) was next determined by plotting oxidation rates in the presence of glutamate/malate as a function of the ADP concentration through the Michaelis-Menten enzyme kinetics–fitting model. The operator was blinded with respect to which sections were issued from a banding animal and which were from a sham animal.
Statistical Analysis
All statistical analyses were performed using SPSS version 17.0 (SPSS, Chicago, IL). Data are expressed as mean ± SD. The Mann-Whitney U test was used to compare continuous variables. Pearson correlation analyses were performed between the different variables of interest and the more altered myocardial deformation (LS). Cox univariate proportional-hazard analysis was performed to investigate the relative effects of afterload increase, LV hypertrophy, fibrosis, and mitochondrial activity on myocardial deformation alteration. A P value < .05 was considered to indicate statistical significance.
Results
Study Population
Table 1 displays main characteristics of the two groups of animals just before surgery. The mean age of the piglets when initially instrumented was 10.5 ± 0.5 weeks in the chronic banding group and 10.0 ± 0.8 weeks in the sham group ( P = .25). Three animals with aortic banding died during the first week after operation (two of scar infection and one of an unexplained cause). Consequently, at a median of 19.0 weeks (week 19) after the surgery, 15 animals (eight in the chronic group and seven in the sham group) were alive and were included in the evaluation protocol. The mean body weight at this time was 32.7 ± 3.0 kg.
Variable | Sham ( n = 7) | Chronic banding ( n = 8) | P |
---|---|---|---|
Body weight (kg) | 12.4 ± 1.7 | 13.6 ± 1.3 | .25 |
LV end-diastolic diameter (mm) | 34.3 ± 3.5 | 32.1 ± 3.0 | .53 |
LV end-systolic diameter (mm) | 21.0 ± 2.4 | 20.8 ± 2.6 | .89 |
End-diastolic septal wall thickness (mm) | 4.9 ± 0.2 | 4.8 ± 0.7 | .92 |
End-diastolic lateral wall thickness (mm) | 4.4 ± 0.8 | 4.3 ± 0.5 | .43 |
LV mass (g) | 36.5 ± 7.1 | 31.4 ± 7.2 | .44 |
LV end-diastolic volume (mL) | 46.1 ± 8.8 | 42.4 ± 9.7 | .63 |
LV end-systolic volume (mL) | 12.4 ± 2.2 | 14.3 ± 4.7 | .83 |
LVEF (%) | 67.9 ± 4.9 | 66.3 ± 7.8 | .99 |
Left atrial volume (mL) | 2.1 ± 0.4 | 2.6 ± 0.9 | .89 |
Mean LV-aorta gradient (mm Hg) | 2.1 ± 0.4 | 2.6 ± 0.9 | .26 |
Mitral E wave (cm/sec) | 68.0 ± 12.2 | 70.0 ± 19.5 | .84 |
Mitral A wave (cm/sec) | 69.3 ± 9.8 | 79.5 ± 18.2 | .14 |
E deceleration time (msec) | 91.7 ± 22.8 | 86.8 ± 14.1 | .99 |
Mean E′ by DTI (cm/sec) | 12.2 ± 3.5 | 11.3 ± 2.7 | .59 |
Mean A′ by DTI (cm/sec) | 13.6 ± 2.8 | 13.1 ± 3.7 | .49 |
Mean S′ by DTI (cm/sec) | 7.1 ± 1.0 | 7.8 ± 1.2 | .48 |
Mean E/E′ | 5.9 ± 1.3 | 6.6 ± 2.2 | .73 |
S′ by DTI in the right ventricle (cm/sec) | 9.9 ± 1.9 | 9.8 ± 1.8 | .99 |
Systolic pulmonary artery pressure (mm Hg) | 20.2 ± 4.4 | 23.9 ± 8.2 | .48 |
Global RS (%) | 45.6 ± 11.7 | 43.0 ± 7.9 | .60 |
Global CS (%) | 18.3 ± 1.3 | 18.2 ± 0.3 | .90 |
Global LS (%) | 21.3 ± 0.9 | 21.6 ± 1.0 | .63 |
Mean basal LS (%) | 19.7 ± 0.6 | 19.9 ± 1.0 | .73 |
Mean mid LS (%) | 22.0 ± 1.2 | 22.1 ± 1.3 | .73 |
Mean apical LS (%) | 23.3 ± 1.5 | 23.2 ± 1.9 | .83 |
Mean septal LS (%) | 20.5 ± 1.5 | 22.0 ± 1.7 | .29 |
Mean lateral LS (%) | 21.3 ± 1.5 | 20.6 ± 1.3 | .44 |
Baseline hemodynamic and Echocardiographic Characteristics
The chronic banding group presented with significantly higher systolic LV pressure than the sham group ( Table 2 ). There were no significant differences between the two groups concerning body weight, blood pressure, CO, stroke volume, dP/dt max , and dP/dt min .
Variable | Sham ( n = 7) | Chronic banding ( n = 8) | P |
---|---|---|---|
Body weight (kg) | 33.9 ± 3.4 | 31.8 ± 2.3 | .39 |
Hemodynamic data | |||
Systolic blood pressure (mm Hg) | 97.7 ± 11.1 | 101.9 ± 12.1 | .84 |
Diastolic blood pressure (mm Hg) | 64.0 ± 11.9 | 71.5 ± 14.6 | .33 |
Heart rate (beats/min) | 112.6 ± 11.9 | 99.3 ± 13.3 | .09 |
Maximal systolic LV pressure (mm Hg) | 99.0 ± 12.4 | 119.0 ± 14.7 | .03 |
Minimal LV pressure (mm Hg) | 11.4 ± 3.0 | 8.4 ± 4.4 | .19 |
End-diastolic pressure (mm Hg) | 17.0 ± 2.8 | 16.0 ± 2.3 | .65 |
CO (mL/min) | 3,357 ± 702 | 3,043 ± 1,312 | .99 |
Stroke volume (mL) | 35.3 ± 11.4 | 25.0 ± 9.8 | .15 |
dP/dt max (mm Hg/sec) | 1,585 ± 626 | 1,276 ± 113 | .31 |
dP/dt min (mm Hg/sec) | 1,709 ± 252 | 1,600 ± 762 | .75 |
Echocardiographic data | |||
LV end-diastolic diameter (mm) | 40.4 ± 5.2 | 39.6 ± 3.8 | .99 |
LV end-systolic diameter (mm) | 26.4 ± 3.6 | 25.6 ± 4.5 | .99 |
End-diastolic septal wall thickness (mm) | 7.1 ± 0.6 | 8.9 ± 1.1 | .002 |
End-diastolic lateral wall thickness (mm) | 6.3 ± 0.5 | 7.4 ± 0.7 | .009 |
LV mass (g) | 76.3 ± 10.7 | 87.1 ± 9.3 | .05 |
LV end-diastolic volume (mL) | 61.3 ± 19.1 | 69.2 ± 15.4 | .46 |
LV end-systolic volume (mL) | 22.7 ± 8.6 | 25.2 ± 9.4 | .32 |
LVEF (%) | 63.1 ± 6.1 | 64.1 ± 6.3 | .89 |
Left atrial volume (mL) | 19.9 ± 3.6 | 31.3 ± 6.4 | .006 |
CO (mL/min) | 3,160 ± 537 | 2,987 ± 369 | .53 |
Dimensionless velocity index | 0.76 ± 0.11 | 0.32 ± 0.06 | .001 |
Cross-sectional aortic area (cm 2 ) | 1.45 ± 0.16 | 0.61 ± 0.11 | .001 |
Mean LV-aortic gradient (mm Hg) | 2.3 ± 0.5 | 17.9 ± 8.3 | .001 |
Mitral E wave (cm/sec) | 64.4 ± 16.8 | 63.6 ± 25.3 | .37 |
Mitral A wave (cm/sec) | 79.1 ± 16.3 | 72.9 ± 15.0 | .58 |
E deceleration time (msec) | 94.4 ± 41.5 | 101.1 ± 37.5 | .37 |
Mean E′ by DTI (cm/sec) | 9.8 ± 1.4 | 10.0 ± 4.2 | .99 |
Mean A′ by DTI (cm/sec) | 8.3 ± 1.7 | 12.3 ± 3.6 | .03 |
Mean S′ by DTI (cm/sec) | 6.9 ± 2.2 | 8.3 ± 2.1 | .18 |
Mean E/E′ | 7.6 ± 1.6 | 6.9 ± 1.3 | .99 |
S′ by DTI in the right ventricle (cm/sec) | 8.0 ± 1.8 | 10.3 ± 0.8 | .06 |
Systolic pulmonary artery pressure (mm Hg) | 24.5 ± 2.7 | 26.2 ± 8.3 | .41 |
Global RS (%) | 48.5 ± 8.2 | 41.8 ± 9.6 | .21 |
Global CS (%) | 18.7 ± 2.4 | 17.2 ± 1.5 | .24 |
Global LS (%) | 20.3 ± 0.7 | 16.9 ± 0.7 | .001 |
Mean basal LS (%) | 18.9 ± 0.5 | 15.2 ± 1.2 | .001 |
Mean mid LS (%) | 20.2 ± 1.0 | 17.0 ± 1.1 | .005 |
Mean apical LS (%) | 22.1 ± 1.3 | 19.4 ± 1.4 | .01 |
Mean septal LS (%) | 19.1 ± 1.5 | 14.9 ± 1.1 | .001 |
Mean lateral LS (%) | 20.8 ± 1.9 | 18.0 ± 1.8 | .05 |
LS/septal wall thickness | 2.9 ± 0.3 | 1.9 ± 0.2 | .001 |
At echocardiography, the chronic banding group presented more LV hypertrophic concentric remodeling compared with the sham group (mean septal wall thickness, 8.9 ± 1.1 vs 7.1 ± 0.6 mm, respectively, P = .002; calculated LV mass, 87.1 ± 9.3 vs 76.3 ± 10.7 g, respectively, P = .05). Moreover, the animals with chronic banding presented a significantly lower dimensionless aortic velocity index through the ascendant part of the aorta compared with sham animals, lower cross-sectional aortic area (0.61 ± 0.11 vs 1.45 ± 0.16 cm 2 , respectively, P = .001), and higher transaortic mean pressure gradient compared with sham (17.9 ± 8.3 vs 2.3 ± 0.5 mm Hg, respectively, P = .001). LVEF and CO were not significantly different between the two groups. The banding group also had higher left atrial volume, but no significant differences concerning diastolic function parameters, systolic pulmonary artery pressure, or right ventricular systolic function were observed ( Table 2 ).
Regarding LV deformations, all strain values were decreased in the chronic banding group compared with the sham group, but this was not significant for RS and CS ( Table 2 , Figure 2 ). Global LS was significantly reduced in the chronic banding group compared with sham animals (16.9 ± 0.7% vs 20.3 ± 0.7%, respectively, P = .001), particularly in the basal and mid LV segments ( Table 2 , Figure 3 ). In addition, in the chronic banding group, LS of septal segments was significantly lower than LS of lateral segments (14.9 ± 1.1% vs 18.0 ± 1.8%, respectively, P = .002).
The ratio of global LS to septal wall thickness was significantly lower in the chronic banding animals compared with the sham animals (1.9 ± 0.3 vs 2.9 ± 0.3, respectively, P = .001) ( Table 2 ). Figure 4 depicts the typical adaptive hypertrophic remodeling secondary to significant chronic aortic constriction.
Effect of Debanding Compared with Baseline Characteristics
As presented in Table 3 , after debanding in the chronic group, we observed a significant decrease of mean transaortic pressure gradient. Moreover, dimensionless aortic velocity index and cross-sectional aortic area significantly increased but without complete normalization compared with sham. LV dimensions and LVEF were not significantly modified by debanding, as well as RS and CS. After debanding, we observed a significant improvement of global LS from 16.9 ± 0.7% to 18.2 ± 1.2% ( P = .004) without normalization compared with sham. This improvement was particularly marked on basal segments ( Table 3 , Figure 3 ). The ratio of LS to septal wall thickness remained lower than in sham after debanding (2.1 ± 0.2 vs 2.9 ± 0.3, respectively, P = .001) ( Table 3 ).
Baseline sham ( n = 7) | Baseline chronic banding ( n = 8) | Debanding ( n = 8) | P , debanding vs chronic banding | P , debanding vs sham | |
---|---|---|---|---|---|
Hemodynamic data | |||||
Systolic blood pressure (mm Hg) | 97.7 ± 11.1 | 101.9 ± 12.1 | 95.6 ± 10.1 | .58 | .94 |
Diastolic blood pressure (mm Hg) | 64.0 ± 11.9 | 71.5 ± 14.6 | 65.5 ± 11.0 | .45 | .84 |
Heart rate (beats/min) | 112.6 ± 11.9 | 99.3 ± 13.3 | 106.0 ± 15.7 | .49 | .33 |
Maximal systolic LV pressure (mm Hg) | 99.0 ± 12.4 | 119.0 ± 14.7 | 96.6 ± 13.1 | .022 | .92 |
Minimal LV pressure (mm Hg) | 11.4 ± 3.0 | 8.4 ± 4.4 | 8.2 ± 2.9 | .80 | .10 |
End-diastolic pressure (mm Hg) | 17.0 ± 2.8 | 16.0 ± 2.3 | 14.7 ± 2.4 | .34 | .20 |
CO (mL/min) | 3,357 ± 702 | 3,043 ± 1,312 | 3,221 ± 1,785 | .94 | .88 |
Stroke volume (mL) | 35.3 ± 11.4 | 25.0 ± 9.8 | 29.6 ± 15.8 | .21 | .50 |
dP/dt max (mm Hg/sec) | 1,585 ± 626 | 1,276 ± 113 | 1,400 ± 316 | .45 | .56 |
dP/dt min (mm Hg/sec) | 1,709 ± 252 | 1,600 ± 762 | 1,540 ± 873 | .67 | .66 |
Echocardiographic data | |||||
LV end-diastolic volume (mL) | 61.3 ± 19.1 | 69.2 ± 15.4 | 57.8 ± 17.1 | .30 | .99 |
LV end-systolic volume (mL) | 22.7 ± 8.6 | 25.2 ± 9.4 | 22.0 ± 8.4 | .53 | .89 |
LVEF (%) | 63.1 ± 6.1 | 64.1 ± 6.3 | 62.4 ± 7.5 | .85 | .67 |
Dimensionless aortic velocity index | 0.76 ± 0.11 | 0.32 ± 0.06 | 0.57 ± 0.11 | .0006 | .02 |
Cross-sectional aortic area (cm 2 ) | 1.45 ± 0.16 | 0.61 ± 0.11 | 1.10 ± 0.19 | .0006 | .05 |
Mean LV-aortic gradient (mm Hg) | 2.3 ± 0.5 | 17.9 ± 8.3 | 7.6 ± 6.0 | .017 | .003 |
Global RS (%) | 48.5 ± 8.2 | 41.8 ± 9.6 | 41.0 ± 14.4 | .72 | .53 |
Global CS (%) | 18.7 ± 2.4 | 17.2 ± 1.5 | 18.3 ± 2.7 | .79 | .59 |
Global LS (%) | 20.3 ± 0.7 | 16.9 ± 0.7 | 18.2 ± 1.2 | .004 | .001 |
Mean basal LS (%) | 18.9 ± 0.5 | 15.2 ± 1.2 | 17.2 ± 2.2 | .014 | .07 |
Mean mid LS (%) | 20.2 ± 1.0 | 17.0 ± 1.1 | 18.1 ± 1.1 | .038 | .008 |
Mean apical LS (%) | 22.1 ± 1.3 | 19.4 ± 1.4 | 19.6 ± 1.4 | .68 | .02 |
Mean septal LS (%) | 19.1 ± 1.5 | 14.9 ± 1.1 | 16.5 ± 1.6 | .04 | .04 |
Mean lateral LS (%) | 20.8 ± 1.9 | 18.0 ± 1.8 | 19.1 ± 2.3 | .45 | .14 |
LS/septal wall thickness | 2.9 ± 0.3 | 1.9 ± 0.2 | 2.1 ± 0.2 | .16 | .001 |
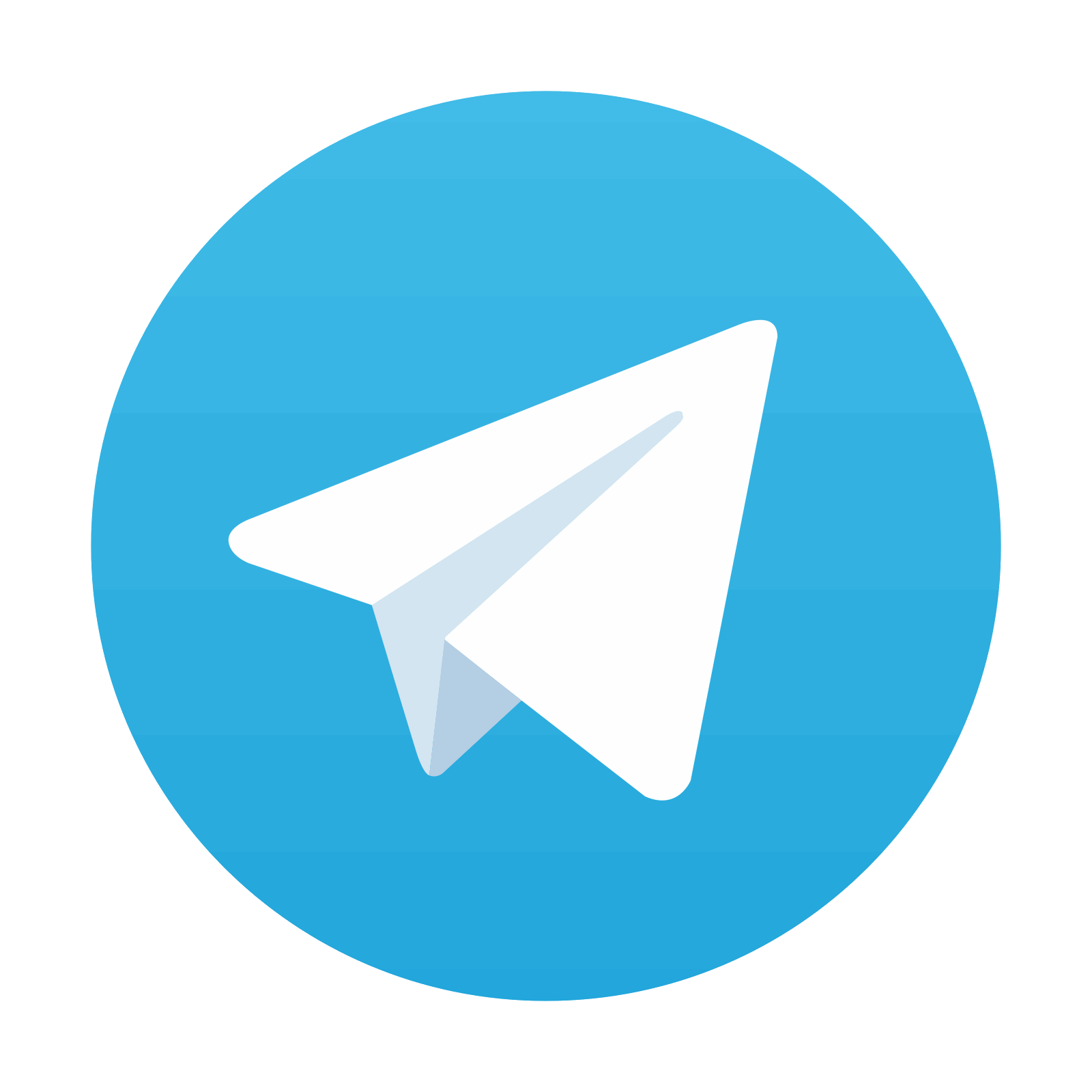
Stay updated, free articles. Join our Telegram channel

Full access? Get Clinical Tree
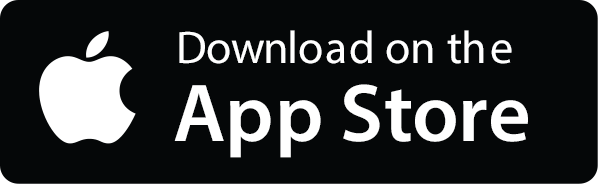
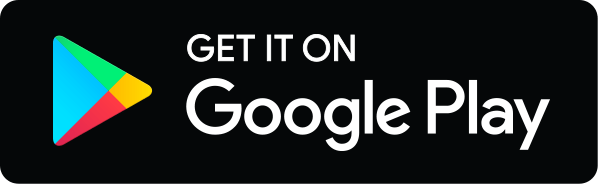
