Fig. 8.1
Cine SSFP images; (a) two chamber right during diastole showing the opened tricuspid valve (b) two chamber right during systole showing closed tricuspid valve (c) short axis at atrioventricular level showing the tricuspid valve area (arrows). S superior leaflet, I inferior leaflet, SSFP steady state free-precession

Fig. 8.2
Cine image of SSFP showing four chambers during systole, jet of tricuspid valve incompetence in the right atrium (red arrow). SSFP steady state free-precession, RA right atrium, LA left atrium, LV left ventricle, RV right ventricle
Advantages
Direct measurement of valve orifice area for stenosed valves by planimetry rather than calculation, and assessment of regurgitant orifices if required [25].
A semi-automatic algorithm based on cine CMR images and 3D reconstruction can help to assess TV annulus morphology and motion and to better depict its ellipsoid saddle shape [6].
Limitations
The thin nature of TV (1–2 mm) makes it particularly prone to partial volume effects due to the slice thickness of CMR images (typically 5–8 mm). Care is therefore required in placing image slices perpendicular to the valve plane to minimize these effects and in minimizing slice thickness to 4–5 mm, but some aspects of finer valve anatomy may be too difficult to visualize well with CMR.
The need to acquire cine images over several cardiac cycles which results in suboptimal visualisation of small or more chaotically mobile objects such as vegetation.
Signal voids seen on SSFP imaging are related to the acceleration of blood rather than the velocity alone, and may underestimate the degree of flow disturbance when assessing the degree of regurgitation. Narrow (mild) jets may be difficult to visualize due to the lack of shear layers at the edge of the jet. SGE sequences are more sensitive than SSFP sequences for evaluating the presence and magnitude of turbulent jets and this sensitivity is increased with lengthening echo time [26, 27].
Assessing the severity of regurgitation with visual assessment of SSFP cine images requires care and caution as the technique is subject to slice positioning, and partial volume effects.
Assessment of Tricuspid Valve Flow
The ability to quantify flow directly using phase-contrast velocity mapping is a unique advantage of CMR, and does not rely on calculation based on complex equations, as echocardiography or invasive catheterization techniques require [28].
CMR Sequence and Image Acquisition
Phase-contrast velocity mapping (other names include velocity-encoded cine, Q flow, or velocity mapping) are used for velocity measurements, and based on the accumulated phase of moving protons. In this sequence, bipolar gradients oriented in the expected direction of blood flow are applied to each frame of the imaging slice of interest to induce phase shifts. Phase refers to the angular position of an individual proton’s spin vector with respect to a frame of reference. Stationary objects within this slice have a net phase of zero, because all phase induced by the first lobe of the bipolar gradient is reversed by the second lobe. Moving objects (blood) gain a net phase depending on the direction of blood flow, and this net phase is proportional to the velocity of blood. This net phase can be displayed as a phase map with differences in signal intensity representing different velocities. Pixels depicting flow in the phase-encoding direction appear bright, and flow opposite to the phase encoding direction appears dark objects with a phase shift of zero (stationary) are grey or speckled, as can be seen in the lungs or chest wall.
Velocity mapping produces two sets of images: magnitude image and phase velocity image . The magnitude image is used for anatomic orientation of the imaging slice and to identify the boundaries of the vessel imaged Fig. 8.3. Blood has an increased signal, whereas turbulent flow is depicted with signal loss within the magnitude image. The phase image encodes the velocities within each pixel. Using both images, a region of interest can be traced on each time frame of the data set. The region of interest must be drawn for each frame of the cardiac cycle carefully because of movement and deformation of the vessel Fig. 8.4. Within each region of interest, the peak instantaneous velocity for each time frame can also be obtained with the simplified Bernoulli equation; the peak instantaneous gradient can be estimated by substituting in the peak instantaneous velocity. Mean pressure gradients are obtained by averaging all of the instantaneous velocities over systole [29].



Fig. 8.3
Phase-contrast velocity mapping images, showing two sets of image (a) magnitude image, (b) phase velocity image, demonstrating the through plane phase-contrast velocity mapping through the tricuspid valve (arrow)

Fig. 8.4
Post processing analysis of through plane phase-contrast velocity mapping images across the tricuspid valve using MASS® program, red contours drawn around the tricuspid valve through the whole cardiac cycle that delivers a time flow curve of tricuspid valve flow
Importantly, in clinical practice there are alternative methods for calculating TV regurgitant volume:
Subtract RV stroke volume (SV) (calculated by SSFP cine images) from forward flow volume in the pulmonary artery (measured on phase-contrast velocity mapping). The difference between the two volumes yields the TV regurgitant volume. The accuracy of this method for TV regurgitant measurement is lower in the presence of irregular rhythms or pulmonary valve regurgitation.
TV regurgitation volume can also be obtained by calculating the difference in RV-SV and LV-SV if only the TV is involved [32].
Recently developed 4D CMR flow resolves the problem of TV annulus motion, owing to retrospective valve tracking and velocity encoding in three orthogonal directions simultaneously during the cardiac cycle allowing the visualisation of complex flow patterns Fig. 8.5 [33]. 4D CMR Flow refers to phase-contrast CMR with flow-encoding in all three spatial directions that is resolved relative to all three dimensions of space and to the dimension of time along the cardiac cycle (3D + time = 4D).


Fig. 8.5
Schematic representation of the reformation of MV flow and TV flow with 3D three-directional VE MR imaging. The 3D acquisition volume for three-directional VE MR imaging is placed at the basal level of the heart. Special attention is paid to the position of the MV and TV remaining inside this 3D volume during the whole cycle. The positions of the respective valvular planes are indicated manually in each of the phases of the cardiac cycle in the two- and four-chamber (4CH) views. The through-plane velocities in the MV plane and the TV plane are reconstructed. Integration of the velocities over the annulus, subtracted by the through-plane velocity acquired in the myocardium, results in the flow graph of the respective valve. Arrows indicate the order in which the steps of the procedure for flow assessment at the particular valves are performed. LV left ventricle, RV right ventricle [33]. Adopted from Westenberg et al., Radiology 2008 December;249(3):792–800
4D CMR Flow visualizations offer more versatile and comprehensive depictions of flow fields than any other in-vivo imaging technique. Further, advanced 4D CMR Flow analysis parameters are currently used in the research setting but require testing for clinical utility. Future work explore the utility of this technique for TV lesions and other areas of clinical utility [34].
Assessment of Right Ventricle Volume and Function
CMR provides accurate and reproducible assessment of cardiac function through acquisition of high spatial and temporal resolution images. It is recognized as the gold standard for quantification of ventricular volumes and function [35]. One of the main advantages of CMR is the ability to acquire images in any plane, which allows clear views of the cardiac valves and their inflow/outflow tracts, irrespective of thoracic anatomy or difficult cardiac anatomy. This is particularly useful for right-sided valves which can be challenging to visualize with echo [36, 37].
CMR Sequence and Image Acquisition
SSFP cine images assess the dynamic changes of cardiovascular geometry during the c ardiac cycle, allowing non-invasive assessment of global and regional cardiac function. [38].
In practice, a slice thickness of 6–10 mm in adults and 5–8 mm in children can be recommended. Slices do not necessarily need to be contiguous, and a small slice gap (e.g., 2–4 mm) is acceptable to reduce the measurement and post-processing time without influencing the accuracy of measurements. Accurate measurement of ventricular volumes, function and mass are vital for assessing the impact of valve lesions on the ventricles. RV volumes are particularly useful as these are difficult to achieve by other methods. CMR-derived ventricular stroke volumes can also be used to quantify mitral and tricuspid regurgitation by stroke volume difference calculation [39].
The basic protocol for ventricular function assessment is very simple. A series of SSFP sequences are acquired for qualitative and quantitative assessment of regional and global systolic function. From the localizers we can obtain the vertical long axis images, followed by the horizontal long axis and the short axis images as shown in the image above Fig. 8.6 [40].


Fig. 8.6
Planning for ventriculography. An axial stack of cine images for ventriculography is planned by adjusting the slice locations on both coronal and sagittal images (top row). A short-axis stack of cine images for ventriculography is planned by adjusting the slice locations on 4- chamber (4C) and left ventricular 2-chamber (LV 2C) images in diastole (bottom row). Note that both the axial and short-axis stacks are prescribed to ensure complete coverage of the left and right ventricles. In this short-axis example, the slices are oriented perpendicular to the ventricular septum on the 4C view, and care is taken to ensure that coverage includes the anterior portion of the dilated right ventricle which extends above the tricuspid valve plane. An alternative short-axis planning approach is to orient the slices parallel to the atrioventricular valve plane on the 4C view (not shown) [40]
RV volumes assessment can be also obtained from acquiring an axial stack. Which can make the identification of the basal slice borders much easier [41]. There are some standardized recommendations by the society of CMR that can help maintain the accuracy and reproducibility of CMR volumetric assessment, with special emphasis on the RV [42]; (1) RV end-diastolic volumes, RV end-systolic volume, RV ejection fraction, RV-SV, and cardiac output, are better indexed to body surface area, (2) The contiguous stack of short axis or axial cine images is evaluated with computer-aided analysis packages, (3) Endocardial borders are contoured at end- diastole and end-systole phases. The RV end-diastolic image should be chosen as the image with the largest RV blood volume. For its identification, the full image stack has to be evaluated and one phase has to be identified as end-diastole for all short axis/axial locations. All contours are included just up to the pulmonary valve and but not superior to this level. Trabeculations of the RV are ignored and a smooth endocardial border is drawn to improve reader reproducibility Fig. 8.7.


Fig. 8.7
Assessment of RV volumes in a patient with hypertrophied systemic RV after atrial switched for transposition. Short-axis view from a multi-phase steady state free precision sequence in end systole (left) and end diastole (right) showing inclusion of trabeculations and papillary muscles in the ventricular cavity. LV left ventricle, RV right ventricle
Assessment of Myocardial Fibrosis
Imaging of myocardial injury or scarring has been described back in the mid-1980s. It was limited by insufficient contrast between normal and injured myocardium.
CMR Sequence and Image Acquisition
The procedure of late gadolinium enhancement (LGE) was described by Kim et al. in 2003. Injection of gadolinium chelate via an intravenous line in a bolus of 0.1–0.2 mmol/kg was described. After a 10–15 min delay the LGE sequence is acquired by a segmented T1 inversion recover gradient echo sequence [43]. An inversion time (TI) has to be properly selected to null the signal from normal myocardium, so that the contrast between scar tissue and normal myocardium is maximized Fig. 8.8 [43, 44]. The quality of LGE imaging depends on the effective nulling of signal from normal myocardium. This requires very precise selection of the appropriate inversion time.


Fig. 8.8
Phase sensitive inversion recovery sequence. By using a phase sensitive reconstruction, the sign of magnetisation is reflected in the displayed signal intensity. The signal intensity is mapped onto a grey scale showing values of zero at the centre of the grey scale, positive values as higher pixel intensities (towards white) and negative values as lower pixel intensities (towards black). Using this method, the effect of a small error in the choice of optimal TI is reduced. The normal myocardial signal can be suppressed by careful windowing of grey scale [43]. Adopted from Ridgway et al. Cardiovascular MR Manual; 2015. p. 129–50
This can also be achieved by performing a so-called TI-scout, which acquires images at multiple time delays after a single inversion pulse within the same acquisition. A sequence called TI scout is acquired with multiple inversion times to choose from before performing the actual IR-GRE sequences. The optimal TI is usually within the range of 200–250 ms [45].
Then the phase sensitive inversion recovery sequences (PSIR) was developed. It did not depend much on the choosing the accurate inversion time. Yet it showed a lower contrast to noise ratio compared to the regular inversion recovery sequences [46, 47]. 2D- and 3D-acquisition sequences are available. 3D acquisitions enable imaging of the entire heart in a single breath-hold, but are prone to image blurring.
Limitations
Most studies with LGE have focused on the assessment of fibrosis in the LV. Desai and co-workers, as well as Amano et Kumazaki, suggested that the RV has a shorter optimal TI than LV. Others as Grosse-Wortmann et al. suggested that this was probably due to partial volume averaging caused by the thin wall of the RV. They suggested that if the spatial resolution was sufficient to visualize clearly the thickness of the RV myocardium, then the TIs for the RV and LV myocardium are most likely similar Fig. 8.9. In cases of TV disease, the presence or absence of myocardial fibrosis has not been proven to influence prognosis. It has been shown to be of more importance in cases of congenital anomalies of the TV [48].




Fig. 8.9
Delayed contrast hyper-enhancement (arrow) showing fibrosis in the RVOT using LGE sequence. LGE late gadolinium enhancement, RVOT right ventricle out flow tract
Classification of TV Dysfunction
Tricuspid Valve Regurgitation
The most common TV lesion is tricuspid regurgitation (TR). Trivial to mild TR is common (60–70%) and appears to be more common in patients more than 50 years old. [49] Although little is known about the significance of mild TR; moderate to severe TR has been associated with increased mortality [49, 50]. Causes of TR are either primary (i.e. intrinsic, organic, or structural) or secondary (i.e. functional TR with anatomically normal TV). The valve morphology can help differentiate the both groups.
Secondary Tricuspid Valve Regurgitation
This is the most common cause of the TR, representing 75% of all TR causes. TV is morphologically normal but its leaflets do not coapt completely during diastole because of valvular annulus dilation and traction of chordae tendinae secondary to RV dilation. Function TR in RV dysfunction (due to left heart disease, RV dysplasia, ischemic RV, and systemic RV) or in RV increased afterload—due to pulmonary hypertension, pulmonary stenosis, or pulmonary embolism.
RV dilatation leads to a distortion of the normal geometric relationships of the TV leaflets, chords, and papillary muscles. With annulus flattening, the lowest point of the annulus is displaced away from the papillary muscles, resulting in tethering of the leaflets and regurgitation due to incomplete coaptation of the leaflets. The critical diameter for annular dilatation to cause functional TR is approximately 27 mm/m2 [51].
Primary Tricuspid Valve Regurgitation
This represents 25% of all TR causes , sub-classified as either congenital or acquired lesions:
Congenital lesions : Ebstein anomaly (the most common), leaflet cleft, and double orifice TV.
Acquired lesions : Rheumatic heart disease, infective endocardiltis, endomyocardial fibrosis, carconid, tumors, traumatic, Iatrogenic (post VSD surgical closure, pacemaker, drugs or radiation).
CMR Imaging for Secondary Tricuspid Valve Regurgitation
The most important role of CMR in secondary TR is to assess the RV volumes and function, since both parameters have been considered independent predictors of TR significant [5]. CMR is considered the gold standard imaging modality to evaluate the ventricular volumes, due to its accuracy and reproducibility. Reference values of normal RV volumes and function for healthy adults are displayed in Table 8.1.
Table 8.1
Reference values of normal RV parameters in healthy volunteers
Parameters | |
---|---|
• RV end-diastolic volume indexed | <108 mL/m2 |
• RV end-systolic volume indexed | <48 mL/m2 |
• Ejection Fraction (EF%) | >47% |
• RV Mass indexed | >20 g/m2 |
Although, there are no defined cut-off values in terms of RV volumes or EF% to indicate surgery in patients with TR, It has been stated that a preoperative RV end-diastolic volume of 164 mL/m2 can effectively discriminated patients with normal RV EF from those with depressed EF at follow-up [56]. However, based on the European Society of Cardiology (ESC) guidelines for the management of grown-up congenital heart disease, significant RV enlargement exists when the end-diastolic volume is ≥150 mL/m2 and significant RV dysfunction when the EF is ≤45% [57].
Patients with a systemic RV pose a challenging group for measuring RV volumes, as the method of delineating the cavity relative to the hypertrophied trabeculations and papillary muscles could affect RV volume and function measurements in this group of patients [58]. Although the influence of these structures on the measured ventricular volumes in individuals with normal cardiac anatomy seems of no clinical importance [59, 60].
It has been reported by Winter et al. [61] that including trabeculations and papillary muscles in the systemic RV cavity lead to a substantially higher measured RV volumes and a substantially lower calculated EF, compared to excluding these structures from the volume of the cavity.
Delineation of the RV cavity boundary outside the trabeculations and papillary muscles had the advantages of shorter analysis time and better inter-observer reproducibility. We therefore recommend the use of this approach in routine CMR measurements of systemic RV volumes Fig. 8.10.




Fig. 8.10
Assessment of systemic right ventricular volumes using two different analysis protocols. Short axis view from a multi-phase steady-state free precision sequence in end systole (left) and end diastole (right) obtained in a patient with an atrially switched TGA demonstrating the two analysis protocols. Method A depicts the inclusion of trabeculations and papillary muscles in the ventricular cavity. Method B depicts the exclusion of trabeculations and papillary muscles from the ventricular cavity. LV left ventricle [60]. Adopted from Winter et al., J Cardiovasc Magn Reson 2008 August 19;10:40
CMR Imaging for Primary Tricuspid Valve Regurgitation
Ebstein’s Anomaly
Ebstein’s anomaly is a congenital abnormality that may present very early in neonatal life by severe cardiomegaly and heart failure, which can be seen on conventional radiography as the classic “box-shaped heart”. It is a rare congenital anomaly of the TV (less than 1% of all congenital heart disease) occurring in about 1–5 per 200,000 live births [62].
The main diagnostic criteria of Ebstein’s anomaly are; tethering of the TV leaflets (mainly the septal leaflet) to the underlying myocardium (failure of delamination); apical displacement of the functional annulus; Dilatation of the “atrialized” portion of the RV, with varying degrees of thinning of the RV wall; Redundancy of the anterior leaflet which becomes elongated “sail-like”; and dilatation of the right atrioventricular junction (true tricuspid annulus). The most typical feature is apical displacement of the hinge point of the valve from the atrioventricular ring of more than 8 mm/m2 body surface area (Fig. 8.11) [63]. The anatomic severity of Ebstein’s anomaly was classified based on echocardiographic appearance as mild, moderate or severe; primarily based on the amount of displacement of the leaflets, the degree of tethering of the anterior leaflet and the degree of RV dilatation [64].


Fig. 8.11
Four chamber view from a patient with Ebstein’s anomaly; (a) Cardiac magnetic resonance image. (b) Autopsy specimen. Solid, short arrows point to the FRV. Solid, long arrows point to insertion of the apically displaced septal tricuspid valve leaflet. Broken arrows show the measurement of displacement of the septal tricuspid leaflet. ARV atrialized right ventricle, FRV functional right ventricle, LA left atrium, LV left ventricle, RA right atrium [62]. Adopted from Attenhofer et al. Int J Cardiovasc Imaging 2012 June;28(5):1147–59
In 1988, Carpentier et al. [65] proposed the following classification of Ebstein’s anomaly; Type A: the volume of the true RV is adequate, Type B: a large atrialized component of the RV exists, but the anterior leaflet of the TV moves freely, Type C: the anterior leaflet is severely restricted in its movement and may cause significant obstruction of the right ventricular outflow tract, and Type D: almost complete atrialization of the RV except for a small infundibular component. The goals of surgery are to improve functional status and reduce the risk of sudden death in patients with Ebstein’s anomaly.
The American College of Cardiology/American Heart Association (ACC/AHA) 2008 guidelines for surgical intervention included other criteria, as symptoms or deteriorating exercise capacity; cyanosis (O2 saturation less than 90%); paradoxical embolism; progressive cardiomegaly on chest x-ray; and progressive RV dilation or reduction in RV systolic function. Nevertheless, the decision about whether to perform surgery in individual patients with Ebstein’s anomaly is often debated because of unpredictable surgical outcomes [66].
Axial SSFP cine images used for visualizing the septal and anterior TV leaflets, coronal SSFP cine images used for assessing the atrialized RV, the RV, and the TV posterior leaflet and sagittal SSFP cine images show the infundibulum and the pattern of attachment of the TV anterior leaflet. Axial or short axis SSFP cine images are usually used for quantification of anatomical and functional RV in Ebstein’s anomaly. Usually an axial stack is preferred, as the basal images in such patients are very difficult to assess in terms of there the outlines of the functional ventricle are Figs. 8.12 and 8.13.



Fig. 8.12
A 14 year old girl known to suffer from Ebstein’s anomaly with no previous surgical intervention. Image (a) is a long axis four chamber plane SSFP cine images showing the marked displacement of the septal leaflet of the tricuspid valve with marked dilatation of the RV which shows a large atrialized portion. Image (b) shows a series of short axis SSFP cine images. The RV is hugely dilated with very wide mouth of the TV and associated mild pericardial effusion (*). LV left ventricle, RV right ventricle, RA right atrium, SSFP steady state free procession

Fig. 8.13
A 16 year old boy with Ebstein’s anomaly, s/p TV replacement. Long (a) and short axis (b) planes of SSFP cine images showing marked displacement of the leaflets of the TV, mainly the septal leaflet (arrow), with dilated anatomical RV and small functional portion of the RV (star). Susceptibility artifact is caused by the artificial TV (red arrow). LV left ventricle, RV right ventricle
CMR limitations patients with Ebstein’s anomaly would be mainly the visualization of small atrial septal defects or patent foramen ovale, as well as sharp visualization of TV leaflet morphology which can be easily answered by echocardiography. Yet CMR offers quantifying of blood flow which allows reliable estimation of shunt volume. Old SGE sequences can replace SSFP sequences for better assessment of TV morphology and abnormal flow jets.
There are also emerging future CMR techniques such as T1-Mapping sequence and CMR Feature Tracking (CMR-FT) sequence that have been used in patients with Ebstein’s anomaly on research basis but not established yet on the clinical. T1-Mapping measures the depiction of diffuse myocardial fibrosis through measurement of myocardial T1 times using the modified look-locker inversion recovery (MOLLI) sequence with and without gadolinium-enhanced inversion recovery-prepared sequences. CMR-FT measures the intra- and inter- ventricular dyssynchrony within, and between, the cardiac chambers by quantitating the differences in time to peak strain duration within the LV and RV and between the two chambers respectively demonstrating RV intra-ventricular dyssynchrony [67].


Rheumatic Heart Disease
Rheumatic heart disease remains a major origin of heart valve disease in the developing world [68, 69]. TV involvement is seen in 6.0–8.5% of cases, and TR develops in two-thirds of these patients. Imaging criteria include thickened leaflets with restriction in motion, diastolic doming (i.e. hemispheric shape of the leaflets due to adhesion), and encroachment of the leaflet tips on the ventricular inlet [69].


Infective Endocarditis
Infective endocarditis (IE) can involve a native TV or valve prosthesis (5–10%) [70]. TV IE is usually associated with the intravenous drug abuse. Associated findings include thickening, shortening, perforations, or complete destruction of the leaflets and intramural or para-valvular abscesses.
Infection-induced endothelial damage leads to cell death and surface deterioration [71]. Further damage may occur if endocarditis progresses into myocarditis, or if vegetation causes coronary artery embolization leading to ischemia, or if infection is spread causing destruction of the conductive tissues. Such effect of IE on the heart may be detected by CMR myocardial damage can be demonstrated non-invasively by detecting gadolinium contrast enhancement in the late phase [72]. These areas of LGE have been shown to be consistent with irreversible myocardial damage and fibrosis. Moreover, the recent CMR sequences T1 and T2 mapping help in tissue characterization [73].
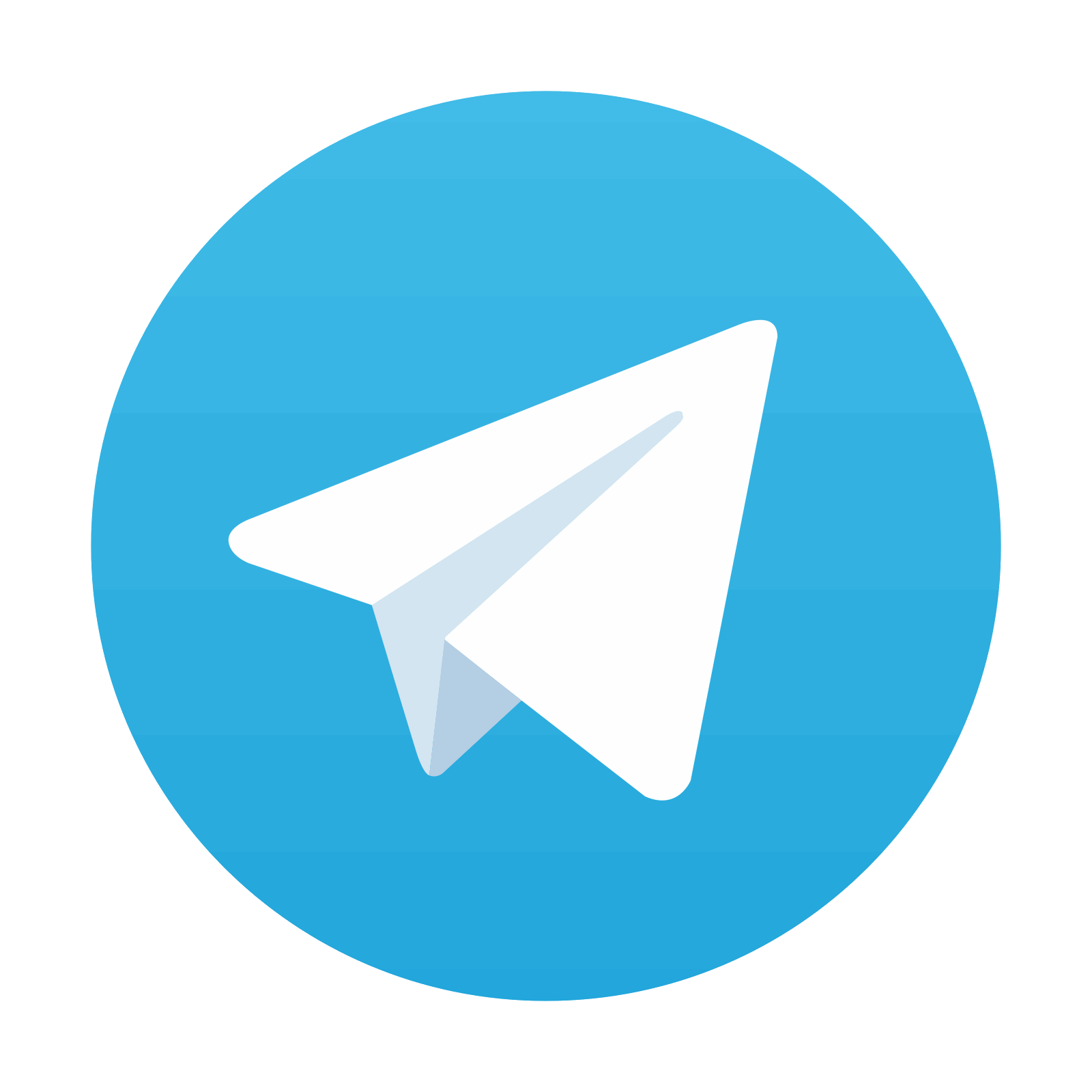
Stay updated, free articles. Join our Telegram channel

Full access? Get Clinical Tree
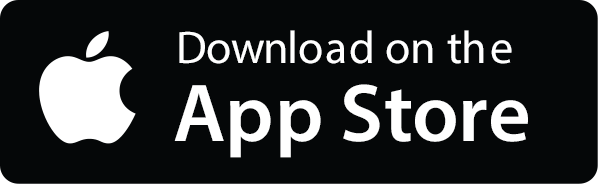
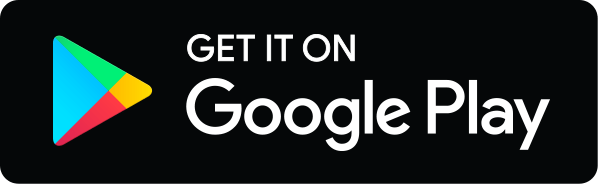