Summary
Patients with pulmonary hypertension must be evaluated using a multimodality approach to ensure a correct diagnosis and basal evaluation as well as a prognostic assessment. Beyond the assessment of pulmonary pressures, the echocardiographical examination allows the evaluation of right ventricular adaptation to elevated afterload. Numbers of variables are commonly used in the assessment of the pulmonary hypertension patient in order to detect changes in right heart geometry, right-to-left interaction and right ventricular dysfunction. Whereas an isolated change in one echocardiographical variable is not meaningful, multiple echocardiographical variable modifications together provide accurate information. In this review, we will link pulmonary hypertension pathophysiological changes with echocardiographical indices and describe the clinical implications of echocardiographical findings.
Résumé
L’imagerie cardiaque multi-modalité est indispensable à l’évaluation diagnostique et pronostique des patients atteints d’hypertension pulmonaire. Bien plus que la mesure des pressions pulmonaires, l’échocardiographie permet l’évaluation de la fonction ventriculaire droite traduisant l’adaptation du cœur droit à l’élévation des résistances pulmonaires. Alors que la modification isolée d’une variable échographique n’est que peu informative, celle de plusieurs paramètres échographiques est plus souvent pertinente. Dans cette revue, nous décrivons les modifications physiopathologiques du cœur droit et du couple VD-AP dans l’hypertension pulmonaire par l’approche échographique et soulignons l’intérêt clinique de ces différents paramètres échocardiographiques.
Background
Pulmonary hypertension is a progressive and severe pulmonary, vascular and cardiac disorder. Pulmonary hypertension is defined by an increase in mean pulmonary artery pressure (mPAP) to ≥25 mmHg at rest as determined by right heart catheterization, the ‘gold standard’ . Usually, patients are screened with echocardiography ( Table 1 ), and right heart catheterization allows measurement of cardiac output and differentiation between pre- and postcapillary pulmonary hypertension. Pulmonary hypertension can be divided into subsets sharing similar underlying pathophysiology, detailed in Fig. 1 .
TR V max | |
≤2.8 m/s + echocardiographical risk factors | PH possible |
2.9–3.4 m/s | PH possible |
>3.4 m/s | PH likely |
Echocardiographical risk factors suggesting pulmonary hypertension | |
Dilated right ventricle/right atrium | |
Increased PR velocity | |
Pulmonary acceleration time <105 ms | |
TAPSE <20 mm | |
‘D-shaped’ interventricular septum |

Although the degree of pulmonary hypertension characterizes this condition, it does not correlate with symptoms or survival. Right ventricular (RV) physiology (RV mass and function) is closely related to functional class, exercise capacity and survival . Advanced therapies, which aim to decrease pulmonary vascular resistance, usually significantly improve RV function, but the observed decrease in mPAP is only moderate.
In clinical practice, ultrasound is by far the most common RV imaging modality, especially in the setting of pulmonary arterial hypertension (PAH). Transthoracic echocardiography provides a number of variables for evaluating right heart haemodynamics ( Table 2 ). It is critical to recognize early signs of PAH with echocardiography in order to reduce the delay between first symptoms and time of diagnosis. Echocardiography is particularly suitable in pulmonary hypertension studies as it is non-invasive, cost-effective and widely available.
Clinical situation | Echocardiographical variables | Clinical relevance | |
---|---|---|---|
Diagnosis | TR V max | RV-RA gradient–estimation of sPAP (4 × V max 2 + RAP) | V max > 2.8 m/s sPAP > 35 mmHg |
PR V max | mPAP = 4 × V max 2 + RAP | mPAP ≥ 25 mmHg | |
Telediastolic PR velocity | dPAP = 4 × velocity 2 + RAP | sPAP = 3 mPAP–2 dPAP | |
Pulmonary acceleration time | <93 ms | ||
TAPSE | Systolic dysfunction | <18 mm | |
S’ | Predictor of sPAP > 40 mmHg | <12 cm/s | |
RV diameter (D1) | >40–42 mm | ||
3D RV end-diastolic volume | >89 mL/m 2 | ||
RV wall thickness | RA pressure | >5 mm | |
RA area | >18 cm 2 | ||
IVC diameter and compliance | >21 mm | ||
Aetiological approach | Intra- or extracardiac shunt | Contrast TTE sometimes useful (TOE if necessary) | Congenital PAH |
TR V max /pulmonary VTI | Increased pulmonary vascular resistance | >0.2 | |
E/E’m | LV filling pressures | >10 (group 2 patients) | |
PA thrombosis | Group 4 patients? (or pulmonary hypertension complicated by PA thrombosis) | ||
Prognosis | TAPSE | <16 mm | |
S’ | <10 cm/s | ||
RV fractional area change | <35% | ||
Peak longitudinal RV strain | ≥19% | ||
IVCv | <9 cm/s | ||
Tei index (PW) | >0.4 | ||
Main PA diameter | >29 mm | ||
LV eccentricity index | >1.4 | ||
Pericardial effusion | Presence | ||
Follow-up | RV diameter | Improves with advanced therapy | |
RV fractional area change | |||
Tei index | |||
Mitral E/A and E/E’ |
Thus, the aim of this paper is to describe the cardiac alterations related to pulmonary hypertension, and to review and discuss the extent to which echocardiography plays a key role in early diagnosis and prognosis in pulmonary hypertension.
Right ventricular anatomy and pathophysiology
The right ventricle (RV) is the most anterior chamber of the heart and can be divided into three chambers: the inlet, the apex and the infundibulum. In comparison with the left ventricle (LV), the RV is characterized by prominent trabeculations that limit the accuracy of contour tracing to delineate the endocardial border. A complex shape, opposite to the ellipsoidal shape of the LV, also characterizes the RV . When viewed in cross section, the RV is crescent shaped, whereas it appears triangular when viewed longitudinally or from the side. However, the RV shape is influenced by RV-LV interactions, depending on the position of the interventricular septum. In normal conditions, the RV volume is larger than the left ventricular (LV) volume, whereas the RV mass represents approximately only one fifth of the LV mass . The myofibre arrangement is also particular, in that almost only two muscle layers compose the RV wall: the superficial layer (circumferential) and the deep layer (longitudinally arranged), thus explaining in normal RVs the predominance of longitudinal contraction, with no intermediate circumferential layer.
Regarding RV contraction physiology, three individual components lead to normal contraction: the inward motion of the free wall; longitudinal shortening; and the traction of the free wall, secondary to LV contraction . In consequence, RV contraction relies mostly on LV-RV interaction and longitudinal deformation.
Right ventricle/pulmonary artery coupling
Under normal circumstances, the pulmonary circulation unit has lower vascular resistance and greater distensibility than the systemic circulation. There is a complex relationship between RV systolic function, preload and afterload. The RV has a heightened sensitivity to afterload elevation (i.e. mainly increased pulmonary vascular resistance). Preservation of RV-pulmonary artery (PA) coupling is critical for the maintenance of RV function: PAs facilitate the transition from an RV pulsed flow to an almost continuous flow at the capillary levels. Under pathological conditions, increased PA stiffness leads to higher pulsatile RV work and promotes RV dysfunction. Resistances in the small vessels mainly represent pulmonary vascular resistance. With regard to this definition, pulmonary vascular capacitance is a measure of global workload of the RV, accounting for large vessels and pulsatile elements. A high-capacitance allows the forward pulmonary blood to be ‘stored’ in the capacitant vessels and decreases the RV load. The RV evolution from compensated to decompensated follows changes in the pulmonary vascular bed: from a normal high-capacitance state to arterial vasoconstriction and proliferative obstructive vascular remodelling.
The RV adapts better to volume overload than to pressure overload. Different types of overload result in different types of RV adaptation. For example, experimental PA banding (pressure overload) leads to RV hypertrophy, mild RV dilatation and reduced exercise capacity with calcineurin activation, whereas volume loading (shunt) leads to hypertrophy and severe RV dilatation with preserved exercise capacity . Echocardiography is helpful for distinguishing volume overload with increased pulmonary pressures but normal pulmonary vascular resistances from normal (or reduced) flow elevated PA pressures and pulmonary vascular resistances (PAH). It is crucial to differentiate between these presentations, especially in atrial septal defect patients, in order to determine the need for defect closure or pulmonary vasodilators.
RV adaptation to increased pressure overload in pulmonary hypertension depends on the overload severity and the rapid onset of the disease; this suggests that RV remodelling has an important role in protecting against pulmonary hypertension (as in congenital PAH). RV myocardial hypertrophy is the first adaptative remodelling response. Changes in sarcomeric protein expression and renin-angiotensin system activation promote RV myocardial ischaemia, whereas dilatation occurs subsequently. The increased preload initially compensates for the reduced contractility, to maintain the stroke volume. The RV becomes more spherical due to the increased RV volume, compressing the LV and causing diastolic dysfunction. With chronic pressure overload, right atrial (RA) contractility and distensibility increase until RA pressure increases. Progressively, as the RV dilates, the filling pressures increase, leading ultimately to RV failure. The intensity of the neurohormonal activation contributes to RV adverse remodelling and failure. The presence of functional tricuspid regurgitation (TR), caused by RV/RA dilatation, also contributes to the reduced cardiac output.
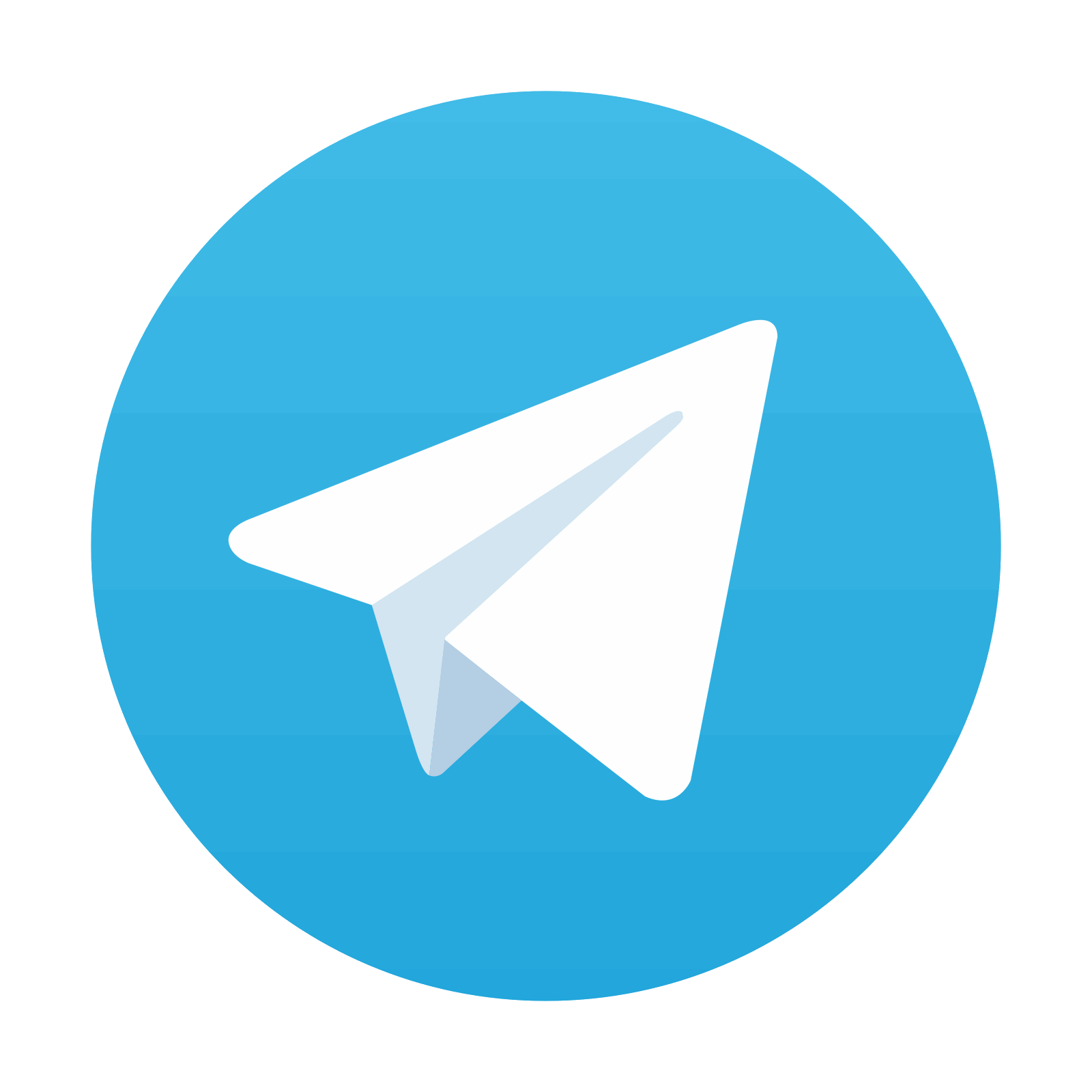
Stay updated, free articles. Join our Telegram channel

Full access? Get Clinical Tree
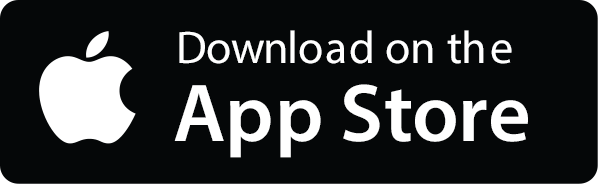
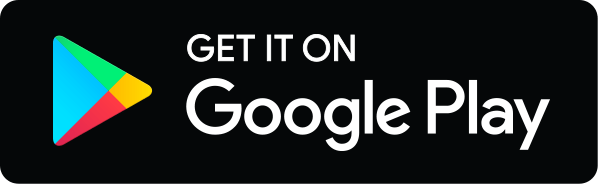