Key Words:
angiography , embolization , therapeutic/methods , tomography , x-ray , computed , ultrasonography , Doppler , duplex
Historical Background
The use of imaging for the evaluation and treatment of vascular injury has evolved in part due to the evolution of available technologies. Egas Moniz, a neurologist, is recognized as the first to use arteriography in 1927. Subsequent to that, surgeons performed arteriography via translumbar or open surgical approaches. The technique for safe percutaneous access and catheter exchange over a wire was developed in 1953 by Sven-Ivar Seldinger, a radiologist. Radiology became the dominant specialty to perform angiography, but the increased use of endovascular and image-guided therapies by other specialties, including surgery, has broadened the use of angiography.
The introduction of new imaging capabilities has changed medical practice. For example, arteriography was once the standard for nonsurgical evaluation of penetrating extremity wounds without “hard signs” of vascular injury, but noninvasive tests became widely adopted with the introduction of Doppler and ultrasound imaging options (coupled with the observation that there were many negative arteriographic examinations). Computed tomography (CT) scan was first introduced to clinical practice in 1972, but it took more than 2 decades of innovation (including helical acquisition with high-resolution multidetector arrays and the development of image postprocessing capabilities) to develop systems that could be used for detailed vascular evaluation. CTA is now a standard for many vascular trauma applications.
Imaging Modalities
Angiography
Angiography is the direct imaging of any vascular structure while arteriography and venography refer more specifically to imaging of arteries or veins, respectively. Intravascular injection of contrast agents allows visualization of anatomy and can be accomplished after direct vascular puncture and placement of a needle or a catheter or through a catheter that is manipulated into the desired position from a remote access site. Angiographic techniques are standard parts of general, trauma, and vascular surgical practice and are typically applied in the resuscitation or operating room (OR). Diagnostic studies and interventions are also performed by radiologists in specialized imaging suites.
Transcatheter angiography provides the highest-resolution imaging of most vascular beds. It provides anatomic definition and a road map for surgical planning or intervention ( Tables 6-1 and 6-2 ). Vascular injury associated with hemorrhage can be demonstrated by extravasation of contrast if bleeding is brisk and ongoing, but it may not be visualized if the bleeding is slow or under tamponade. Bleeding may also be missed if the contrast bolus is too small, if it is injected into a different vessel, or if image acquisition is terminated too soon.
Lesion | Findings | Notes |
---|---|---|
Arterial stenosis |
|
|
Arterial occlusion |
|
|
Active hemorrhage |
|
|
Pseudoaneurysm |
|
|
Arteriovenous fistula |
|
|
Lesion | B-Mode Image | Color Doppler | Pulsed Doppler | Notes |
---|---|---|---|---|
Arterial stenosis |
|
|
|
|
Arterial occlusion |
|
|
|
|
Pseudoaneurysm |
|
|
|
|
Arteriovenous fistula |
|
|
| |
Venous thrombosis |
|
|
|
|
Angiographic interruption of vessel continuity usually indicates vessel disruption or thrombosis. With sufficient contrast injected, distal reconstitution of flow (i.e., beyond the disrupted segment) from collateral vessels can typically be demonstrated. Imaging of distal arterial beds can be compromised by hypoperfusion and vasoconstriction, typical manifestations of hemorrhagic shock. Vasospasm, with tapering of arteries (sometimes to occlusion) and slow flow, can be more prominent in young patients who have a greater degree of vasomotor reactivity.
Early venous filling after intraarterial contrast injection indicates the presence of an arteriovenous fistula. Traumatic pseudoaneurysms result from focal disruption of arterial-wall integrity, with blood flow contained by adventitia or surrounding tissues. A pseudoaneurysm appears as a focal outpouching of contrast beyond the normal artery wall. Intimal flaps and focal segments of nonocclusive thrombosis may be detected as filling defects or lucencies within the contrast column, sometimes with a delay in distal contrast flow.
Indications
Transcatheter angiographic evaluation is a useful intraoperative tool for trauma patients who have been taken to the OR, either to diagnose or to localize and treat vascular injury. CTA, which is commonly used for evaluation of the injured patient, can be limited by artifacts from metallic fragments and occasionally by soft-tissue air or streak artifacts. In such cases, conventional angiography may be necessary. Shotgun or fragmentation wounds that have multiple metallic fragments are often best evaluated with direct catheter angiography. Arteriography is also indicated when a known vascular injury requires detailed anatomic characterization to guide management.
In a modern wartime experience at Walter Reed Army Medical Center, military surgeons found that examination and clinical assessment were not sensitive for detection of delayed or occult arterial injuries from complex extremity wounds. Routine arteriography in this patient group helped identify lesions that were amenable to endovascular therapies. Transcatheter arteriography should be considered in cases of complex trauma involving high-energy, penetrating mechanisms or wounding patterns in proximity to named vessels.
Transcatheter angiography is often used as part of a strategy for endovascular therapy of vascular injury. If angiography demonstrates significant arterial injury in the acute setting, low-pressure inflation of a compliant balloon may be used for inflow occlusion. In such cases, endovascular techniques may be used for hemorrhage control or even definitive management of the injury.
Preparation
As intravascular contrast agents are used for transcatheter arteriography and CTA and have the potential for nephrotoxicity, it is important to be aware of risk factors for contrast-induced nephropathy (CIN). This complication of contrast use is usually defined as either a greater than 25% increase of serum creatinine or an absolute increase in serum creatinine of 0.5 mg/dL after use of a contrast agent. Factors associated with increased CIN risk include hypotension (systolic blood pressure <80 mm Hg), heart failure, advanced age (>75 years), anemia, diabetes, preexisting renal insufficiency, and increasing volume of contrast.
Baseline assessment of renal function is advised. Patients with a history of allergic reactions to contrast may be premedicated with intravenous corticosteroids and histamine blockers. Avoiding use of large doses of contrast (as may occur in patients undergoing multiple imaging procedures), avoiding hypovolemia (a practical concern in many trauma patients), and monitoring renal function are recommended. There is limited evidence suggesting that the use of N-acetylcysteine, theophylline, sodium bicarbonate, and statins reduce incidence of CIN. Other interventions such as renal replacement therapy, angiotensin-converting enzyme (ACE) inhibitors, dopamine, and Fenoldapam do not have confirmed benefits.
Pitfalls and Danger Points
The risks of contrast angiography include the following:
- •
Vascular access site complications such as vessel injury (e.g., hematoma, pseudoaneurysm, embolization, thrombosis)
- •
CIN
- •
Anaphylactoid response to contrast
- •
Technical and time requirements
- •
False-negative studies
- •
Risks of ionizing radiation
Catheterization for angiography poses a small risk of iatrogenic vascular injury at the site of access. The risk of access site injury is minimized with the use of ultrasound guidance, direct operative exposure, and/or the initial use of small-caliber access needles and wires (i.e., micropuncture devices). Use of isoosmolar contrast (iodoxinol) and reduced volumes of contrast reduce CIN risk. Allergies to shellfish do not predict risk of a contrast reaction, but history of prior contrast reactions may. As premedication to reduce risk of a reaction requires steroid treatment hours before exposure, use of alternative imaging modalities may be needed if a patient is determined to be a high-risk for a reaction.
Diagnostic yield depends on the angiographic technique used and the size and location of the vessel being imaged. Injuries may be missed in high-flow vessels (such as the aorta) due to rapid washout of contrast, or an injury may be missed if imaging is performed in only a single plane. Improper timing or an insufficient contrast bolus may result in poor quality imaging, especially if the contrast is not directly administered into the vessel of interest via selective or subselective catheterization.
There is attendant risk with the use of ionizing radiation (x-rays) for angiography, though the risks to most patients are usually negligible. Surgeons and staff who have regular exposure to X-rays during procedures should have specific training in radiation safety and practices to minimize their own occupational radiation exposures. It is important to note that scatter from the patient is the main source of radiation to which medical personnel are exposed. Practices to maintain exposure to levels as low as reasonably achievable (ALARA) should be mandated. Occupational radiation doses may be affected by factors that cannot be readily modified, including the size of the patient and the part of the body being imaged. Specific actions to reduce radiation doses include reduction of the time of exposure, increase of the distance from the source of the scattered radiation, and effective use of shielding (including lead garments and glasses).
Operative Strategy
There are three tiers of technical sophistication for trauma arteriography, as follows: (1) simple “on table”; (2) portable C-arm; and (3) fixed, floor, or wall-mounted system either in an imaging suite (i.e., radiology department unit) or in a traditional OR space. Resource availability and clinical circumstances typically dictate which approach is used. Patients who are hemodynamically or physiologically unwell may need to be taken immediately to the OR for management of their injuries. Although modern trauma centers are moving to building fixed imaging systems in their trauma or resuscitative ORs, the advanced features associated with this capability may not be available in most centers. As such, on-table angiography using several single-picture x-rays or with a portable C-arm using basic cine loop angiography (with or without digital subtraction) is required.
In addition to diagnostic capability, endovascular techniques can be used to manage hemorrhagic shock and certain patterns of vascular trauma. Temporary deployment of an endovascular balloon can provide proximal occlusion for hemorrhage control during open operative resuscitation or surgical management of vascular injuries. In selected cases, transcatheter management can provide definitive therapy. Endovascular treatment with embolization is standard in the management hemodynamically unstable patients with pelvic fractures. The yield of angiographic identification of a pelvic source of bleeding ranges from 43% to 78%. Sources of hemorrhage include injuries to major pelvic arterial and venous structures, but a small vessel disrupted by fractures can bleed substantially and transcatheter angiography often directly identifies these sources of bleeding.
Angiographic findings indicative of vascular injury or disruption include uncontained extravasation of contrast, pseudoaneurysm or contained extravasation of contrast, arteriovenous fistulae, intimal tear, spasm, or occlusion. Selective catheterization with flow-directed particulate embolization is one method of controlling bleeding from small arteries at sites of injury. Embolic coils may be deployed to proximally occlude an injured vessel, but temporary occlusion alone may be inadequate for pelvic trauma. As such, catheter-directed use of inexpensive and readily available materials such as gelfoam pledgets or slurry into the source vessels (Upjohn, Kalamazoo, MI) can also be effective.
Traumatic injuries of the descending thoracic aorta are preferentially managed with thoracic endovascular aortic repair (TEVAR). Early experience with TEVAR highlighted shortcomings of first-generation graft devices, which were designed primarily to treat aortic aneurysms. However, current devices are available in sizes that better fit a normal-caliber aorta and that better appose to the distal transverse arch and proximal descending thoracic aorta.
Covered stents and other endovascular strategies can be used for extremity vascular injuries as well although their benefit is less obvious in this setting. Because injuries in patients who are in shock require immediate attention and because extremity vascular injuries are often associated with skeletal, soft-tissue, or other trauma, open surgical repair remains more common than an endovascular approach. However, endovascular techniques may be advantageous when the extremity exposure is difficult or associated with considerable morbidity, as with injuries to the subclavian or axillary arteries.
The operative strategy will vary with the situation. Factors to consider include the patient’s hemodynamic and physiologic status, the level of interventional expertise, the quality of the available imaging systems, and the inventory. Endovascular approach maneuvers may temporize or definitively control hemorrhage (e.g., balloon catheter occlusion or embolization). Some endovascular therapies may be delayed for hours or even days (e.g., TEVAR for traumatic aortic injury [TAI]).
Surgeon training and experience, or the availability of an interventional specialist, may determine whether an open surgical therapy, an endovascular treatment, or a mixed or hybrid approach is most practical. Simple endovascular maneuvers for arterial access and pressure monitoring, hemorrhage control and resuscitation (i.e., resuscitative endovascular balloon occlusion of the aorta), and arteriography should be in the armamentarium of general and trauma surgeons. Advanced endovascular techniques, subselective catheterization, use of aortic endografts, and other complex maneuvers require additional training and credentialing.
Most endovascular procedures for management of vascular trauma or hybrid procedures that combine an open and endovascular approach can be performed with portable or mobile C-arm imaging systems. While imaging capabilities are often superior in specialized fixed imaging suites or hybrid ORs, basic diagnostic and therapeutic maneuvers are supported with mobile fluoroscopy. When possible, more complex vascular interventions should be performed where the best imaging is available.
Angiography and simple endovascular interventions can be performed with a relatively limited inventory of access needles, wires, and sheaths as well as catheters, working wires, balloons, and stents. As more complex interventions are contemplated, it becomes necessary to include sufficient inventory to ensure success. Supplies for involved interventions include aortic endograft systems, large sheaths and compliant aortic balloons, snares, microcatheters, embolic devices and agents, and covered stents. It is also important to have an appropriate range of sizes to meet a variety of needs. The availability of anticipated supplies must be confirmed before embarking on a plan of therapy.
Operative Technique
On-table angiography requires rudimentary skills and equipment. Contrast is injected by hand, and a single radiograph is obtained. This technique may be of practical use during operative management of extremity injuries when the presence, location, or extent of an injury is uncertain ( Fig. 6-1 ). It can also be used to evaluate the technical result of a vascular repair. Vascular access is obtained, either in a percutaneous manner or after open surgical exposure of the vessel. The artery in question is accessed using a needle, a butterfly set, a catheter, or a sheath placed using the Seldinger over-the-wire exchange technique. The imaging plate can be inserted in a sterile wrap and positioned on the surgical field under a limb to be imaged.

While this simple and useful arteriographic technique is available for use in any situation, it has limitations. First, the delay between contrast injection and imaging must be estimated, and errors in timing the transit of contrast to the area of interest will result in failure to opacify the vascular segment of interest. Second, this technique provides only one image per injection. Each individual image must be processed for evaluation of the adequacy of the technique, the projection, and the field of view. This can be time consuming.
The limitations of single-image, on-table angiography can be overcome by the use of a portable C-arm fluoroscopy system with cine loop recording and digital subtraction capabilities. By using cine loop angiography timing of imaging is less critical as many images are recorded per second of any single contrast injection. Digital subtraction angiography (DSA) provides superior definition of vessels as it removes the image of overlying or surrounding structures from the vessels of interest. Because of this advantage, DSA generally requires less contrast than nonsubtracted angiography including that of the aorta and visceral vessels.
Use of mobile cine loop fluoroscopy also enables imaging of selective catheterization maneuvers with shaped wires and catheters. Mobile fluoroscopy provides the imaging needed for endovascular interventions such as embolotherapy or placement of covered stents ( Fig. 6-2 ). It is important to note that in order for fluoroscopy to be used effectively, the patient must be positioned on a radiolucent operating table designed for imaging. Many of the endovascular tables float to allow easy positioning of the patient by the surgeon while the fluoroscopy unit remains stable. Alternately, the patient may be positioned on any operating table that is radiolucent in the area to be imaged, as long as the C-arm can be positioned appropriately. Many tables used for trauma surgery and orthopedic procedures, including the Jackson table, are radiolucent and suffice for basic fluoroscopic imaging and endovascular intervention. Use of a fixed table, however, requires the radiology technician to be more actively involved in C-arm positioning to center the field of view.

Fixed imaging systems refer to those that are wall, ceiling, or floor mounted and that are integrated with a contrast management or injection system. Fixed imaging units are also programmed with the table and projection screens to allow efficient performance of catheter-based angiographic procedures. Fixed imaging systems also provide larger imaging fields and magnification capability which provides striking detail and resolution of select vessels of interest ( Fig. 6-3 ). Centers that have invested in fixed imaging suites in the OR (i.e., hybrid ORs) also have a more extensive inventory of catheters, guidewires, interventional supplies, and accessory equipment.

The additional capabilities associated with a fixed imaging unit come with a price because these rooms are expensive and require specially trained radiology or cardiovascular technologists for operation. The physical space, the manpower requirement, and the baseline cost may limit the availability of fixed imaging rooms for trauma in most centers today. However, as diagnostic and therapeutic imaging for vascular trauma continues to prove more and more useful, the widespread availability of these hybrid ORs seems inevitable.
Postoperative Care
Removal of arterial catheters and sheaths should be done by trained personnel following correction of coagulation abnormalities. Use of percutaneous arterial closure devices may be relatively contraindicated if there is concern for a break in sterile technique, because the presence of foreign material may increase risk of vascular infection. The arterial access site and the extremity in which the access was obtained should be monitored for evidence of injury following sheath removal. Neurologic and extremity examinations should look for signs of bleeding and/or embolic or thrombotic complications. Postprocedure laboratory tests should include serum creatinine and hemoglobin measurements.
Patients who have had interventions for hemorrhage control or ischemia require careful observation to ensure that there has been sustained technical success. Such patients should be monitored with periodic physical examination, and repeat measurement of hematocrit should be performed to ensure the absence of bleeding. Stent grafts placed for traumatic aortic injury are evaluated with intraoperative imaging, but postoperative pulse checks in the upper and lower extremities are needed to confirm that there has not been coverage of the left subclavian artery or infolding of the graft resulting in distal ischemia. CT angiography is generally used for endograft imaging and surveillance in the days and weeks following placement. It is important to note that for many endovascular therapies, postprocedure noninvasive duplex ultrasound can be used to confirm patency of treated segments. Duplex scans are inexpensive and avoid the use of contrast, and radiation and can be used for longer-term surveillance.
Complications
Inadequate hemostasis at the arterial puncture site leads to bleeding and hematoma, and any communication between the access artery and vein results in an arteriovenous fistulae. An intimal flap, distal embolization, or de novo thrombosis at the arterial access site can lead to varying degrees of limb ischemia with or without neurologic deficit. As such, vascular access sites should be carefully assessed for the presence of ecchymosis, a mass, or bruit; and the distal limb should be examined for signs of ischemia.
Ultrasound
Ultrasound imaging has many advantages that make it useful in the care of the injured patient. It is noninvasive, relatively inexpensive, and increasingly available for point-of-care examinations; and it can be used to image numerous organs or regions of interest. Ultrasound is of particular utility in evaluation of neck and extremity vessels following trauma due to their relative superficial location. Good image quality, a selection of imaging modes and processing features, and a range of transducer options are now available with most ultrasound systems. The introduction of compact systems for point-of-care imaging has made it possible to perform ultrasound examinations in prehospital settings (including austere or remote environments), as well as in a range of clinical settings (including emergency rooms, ORs, and intensive care settings). Imaging without the use of ionizing radiation allows ultrasound systems to be used without concern for patient or provider radiation exposure.
Early generations of compact, portable ultrasound systems were substantially inferior to the larger, heavier, full-featured systems used by radiology departments and vascular laboratories. However, continued evolution in beam-forming and image-processing technologies narrowed the capability and quality gap between compact, highly portable devices and traditional high-end systems. Size and mass of ultrasound systems continues to decrease, making for a small device footprint, and acquisition costs for these systems continues to decrease. As a result, use of point-of-care ultrasound systems for trauma has become increasingly common.
B-mode imaging provides a 2-dimensional (2-D) gray scale representation of tissue in the scan plane. Blood is hypoechoic. The lumen of vessels will appear dark on B-mode imaging. Real-time imaging can demonstrate dynamic features of vessels, including the pulsatile expansion of arteries and collapsibility of patent veins if external pressure is applied with the probe (scan head) during the examination.
The resolution of B-mode ultrasound is related to the transducer frequency and the depth of the imaged structure. Resolution decreases with the use of lower-frequency transducers, which are used for the examination of deeper structures. When superficial anatomic features are evaluated with high-frequency transducers, details of vessel walls can be seen, including atherosclerotic plaque, dissection or intimal flap. Conversely, use of B-mode ultrasound as a stand-alone modality may be insensitive for detection of vascular injury when deeper vessels are evaluated. In these instances the only abnormal finding may be a hematoma in proximity to the vascular injury. Patient discomfort (or the agitation or the presence of wounds), external fixators, or dressings may limit ultrasound examinations for trauma.
Duplex scanning, with the addition of Doppler flow detection to the B-mode image, increases the utility of diagnostic vascular ultrasound. Flow information from a specific point of interest is displayed by the pulsed Doppler flow velocity waveform. Color flow duplex scanning displays areas with flow in color overlying the B-mode image of anatomy. Color flow imaging assigns colored (rather than gray scale) pixels in regions where moving tissue (e.g., blood) returns a Doppler-shifted echo. The color flow display provides information about the location of the flow, its direction, and its velocity. In addition, a speaker provides an audio output of the Doppler signal. With experience, users can learn to recognize characteristic signatures of abnormal flow, including higher pitch with elevated velocities; abrupt blunted signal proximal to an occlusion; course sound with spectral broadening from turbulence; or continuous low-resistance, diastolic flow associated with an arteriovenous fistula.
Use and interpretation of vascular ultrasound is integral to the training of surgeons. The Registered Physician in Vascular Interpretation (RPVI) credential of the Association of Registered Diagnostic Medical Sonographers (ARDMS) has been established as a prerequisite for vascular surgery board certification. Vascular ultrasound may be useful for trauma care, even if vascular specialty expertise is not available. Most radiologists have training in general ultrasound, and many general and trauma surgeons have skills with the use of point-of-care ultrasound. Measurements of vessel size (detection of aneurysms), detection of arterial or venous flow, assessment for deep-vein patency, mapping of superficial veins, and other simple evaluations can be learned without extensive formal training.
Indications
Focused assessment with sonography for trauma or FAST as part of the secondary survey of the injured patient may identify pericardial effusion and hemoperitoneum. The extended FAST or eFAST includes ultrasound assessment of both thoraces looking for pneumothorax. Although not part of the traditional FAST, ultrasound can also confirm endotracheal tube positioning and can provide an indication of intravascular volume by evaluating ventricular filling and the dimensions of the inferior vena cava. A very practical aspect of ultrasound in this setting is its ability to be repeated over time to confirm initial impressions or show trends.
Duplex ultrasound scanning is indicated to detect or characterize vascular injuries of the neck and extremities, especially where vessels are relatively superficial. Dissection, stenosis, thrombosis, and arteriovenous fistula can all be demonstrated using this imaging modality. Because duplex is safe, inexpensive, and noninvasive, it is especially useful to confirm a normal physical examination in patients who have injury mechanisms that are associated with a risk of vascular injury, such as penetrating trauma, posterior knee dislocation, hyperextension, and supracondylar fracture.
In most cases, the presence of an extremity vascular injury can be excluded with a combination of physical examination and noninvasive pressure measurement. Using continuous-wave Doppler, the cuff occlusion technique measures the systolic blood pressure in the injured limb, which can be compared to the pressure in the uninjured contralateral limb. An injured extremity index of <0.90 suggests a flow-limiting arterial stenosis or occlusion. Duplex scanning complements nicely a measurement of the injured extremity index; however, in the absence of clinically evident ischemia or bleeding, there is little risk in delaying the duplex scan by hours or even days.
When minor vascular injuries are diagnosed, most may be managed nonoperatively with expectation of spontaneous healing. Injuries with low risk of late complications include intimal injuries (intimal flap) that are associated with <50% stenosis. The absence of a pressure gradient across the injured segment (i.e., a normal injured extremity index) or a duplex finding of peak systolic velocity increased by less than a factor of two suggests the absence of a hemodynamically significant injury. The noninvasive nature of duplex allows for serial examination to confirm a benign injury outcome.
Severely injured patients are at risk for venous thrombosis and pulmonary embolism. The presence of significant injury causes thrombophilia (a procoagulant condition). Duplex scanning is the diagnostic test of choice for the detection of venous thrombosis of the extremities. New unilateral limb swelling is the best clinical sign that predicts deep vein thrombosis (DVT), but clinical evaluation alone lacks the sensitivity or specificity. Thus, duplex scanning to evaluate for DVT is indicated when there are signs or symptoms of DVT or to screen for DVT in asymptomatic high-risk patients. Simple point-of-care testing should be aimed at probe compression to verify that the popliteal and common femoral vein collapse under manually applied pressure. With this basic bedside maneuver, one can exclude the presence of a major proximal limb DVT. To diagnose iliac vein thrombosis, nonocclusive or limited segmental DVT, or calf vein thrombosis, a complete examination should be performed by a vascular technologist.
As has been noted, the noninvasive and available nature of ultrasound means that this imaging modality can be repeated over time for surveillance or follow-up examination as needed. For example, if limited thrombosis (e.g., isolated calf vein DVT) is observed and is not treated with anticoagulation, repeat duplex examination 5 to 7 days later may be used to detect proximal thrombus progression. Intraoperatively, ultrasound can be useful for localization of vessel injuries and for evaluation of technical results after repair of vascular trauma ( Fig. 6-4 ). Duplex can identify defects that may lead to early thrombosis or late complications including abnormalities of the intima at the site of vascular clamp placement (i.e., clamp injury). Duplex can also detect flow-limiting stenosis at the anastomosis of a vascular repair or the presence of intraluminal thrombus. By detecting these injuries or technical defects intraoperatively, surgical revision can be performed before leaving the OR.

Preparation
No specific preparation is needed for most ultrasound examinations. However, fasting before abdominal duplex may reduce the amount of bowel gas that obscures the view of deeply positioned abdominal, retroperitoneal, and pelvic vessels.
Pitfalls and Danger Points
The hazards related to or limitations of ultrasound are negligible but include the following:
- •
Results may be operator dependent.
- •
Tissue disruption, obesity, or edema may limit imaging.
- •
Ultrasound transmission through air is poor; imaging of intrathoracic structures is limited; and bowel gas may obscure abdominal and pelvic imaging.
- •
Bowel gas may be increased in nonfasting patients and in trauma patients who have been ventilated with a mask before intubation.
Strategy
Providers at the point of care can perform vascular-specific examination or a technologist may perform an ultrasound during the tertiary survey or at any subsequent step of a patient’s care. Ultrasound can be used as a screening tool, to detect injuries or vascular complications that are not evident from clinical assessment. Examples of screening examinations include evaluation of limbs with “soft” or no signs of vascular injury that sustained mechanisms of trauma known to predispose to vessel injury. Ultrasound can be used as a diagnostic tool, either alone or in combination with other testing modalities. Arterial disruption, intimal dissection or flap, thrombosis and arteriovenous fistula can be definitively diagnosed using ultrasound, especially in the extremities. If duplex is combined smartly with thorough physical examination, additional imaging such as CTA or angiography may be unnecessary. As has been noted, duplex may complement other diagnostic tests or maneuvers such as Doppler pressure measurements in the initial screening of an injured extremity. In many cases, if the initial examination is normal and there is no hard sign of vascular injury, a more thorough evaluation with duplex can follow on an elective basis.
The utility of ultrasound as a tool to guide invasive procedures should not be overlooked. This modality has become increasingly the standard to guide percutaneous arterial or venous access and to help with localizing a vascular lesion during operative surgical management. Focal pseudoaneurysms of extremity arteries may be managed with US-guided thrombin injection, a technique that is commonly used to treat iatrogenic femoral artery pseudoaneurysms, but it can also be used for other arterial pseudoaneurysms ( Fig. 6-5 ). Finally, ultrasound can be used for assessment of outcomes, either during a procedure (when corrective action can be taken if a technical defect is found) or later, if surveillance is indicated.

Technique
The hand-held transducer (probe) transmits ultrasound energy and receives reflected echoes. As air has high acoustic impedance, a water-based gel is used for acoustic coupling between the transducer and the skin for routine applications; but blood or saline irrigation provides suitable coupling during intraoperative use.
A transducer that is appropriate for the depth and the location to be evaluated is selected. Deeper structures require the use of lower ultrasound frequencies (1 to 5 MHz). High-frequency transducers (6 to 12 MHz) provide better imaging resolution, but with limited imaging depth. Transducer elements can be mounted in a curved or linear array to create a sector or boxlike image. Phased array transducer can provide a compact footprint, with a sectorlike scan. Probes designed specifically for intraoperative use may have a T-shaped or hockey-stick design to facilitate use in the operative field. Transducers are typically designed to operate over a range of frequencies (broadband) for greater versatility and better imaging. Specifics of transducer design vary among manufacturers and systems used.
Vessels in the neck and extremities can be imaged directly. Because air in the lungs and viscera interferes with ultrasound transmission, ultrasound imaging to evaluate for truncal vascular injury is limited. Subcostal and parasternal windows allow for evaluation of the heart and pericardial sac, but the thoracic aorta cannot be visualized with a transthoracic approach. The presence of intraabdominal fluid (blood) on a FAST examination is indirect evidence of vascular disruption or injury either of a main axial vessel or of those within the hilum or parenchyma of a solid organ. Direct vascular examination of intraabdominal vessels is seldom performed in the early phases of trauma evaluation and management (i.e., as part of the FAST).
When ultrasound is used for procedural guidance or intraoperative assessment, the transducer is sheathed in a sterile sleeve. Acoustic coupling gel needs to be in the sleeve, with no air gap or bubbles between the transducer face and the sleeve. Sterile gel is used on the field for ultrasound-guided endovascular procedures. Vascular access is facilitated with routine ultrasound use for venous and arterial sites. During this process, an 18- or 21-gauge needle can be observed or directed into the vessel lumen with ultrasound. Needles with a stippled surface may be more echogenic and easier to visualize with ultrasound. Imaging the vessel in a longitudinal scan plane allows the site of vascular entry to be selected, while changing to a transverse image ensures that vessel entry is precisely at the 12 o’clock position. Ultrasound imaging can also confirm intraluminal positioning of catheters and guidewires.
There are some ultrasound imaging applications that require specialized capabilities. Transesophageal echocardiography (TEE) can be used to evaluate the thoracic aorta for potential injury. TEE for trauma evaluations usually requires general anesthesia and endotracheal intubation for airway control. TEE may provide the initial diagnosis of thoracic aortic injury (TAI) if a patient is taken immediately to the OR for surgical stabilization, bypassing the CT scanner.
Intravascular ultrasound (IVUS) is an invasive technology requiring vascular access, catheterization of a target vessel, and introduction of an imaging catheter over a guidewire. The aorta can be evaluated with an 8 French catheter over a 0.035-inch wire. IVUS is not appropriate as a screening test, but it can provide detailed morphologic information that can guide the choice of therapeutic devices (stent grafts) for the endovascular treatment of TAI. IVUS can be used to improve the accuracy of sizing of aortic endografts for TEVAR as CTA may underestimate aortic diameter, especially in the setting of intravascular hypovolemia. IVUS allows real-time imaging and diameter measurements in both systole and diastole while the patient is on the OR table.
Postexamination Care
There are no specific postexamination care concerns.
Complications
Ultrasound is safe, is noninvasive, and is not associated with any direct risk of complications. Tissue heating is negligible with diagnostic ultrasound applications, and there is essentially no risk of injury in typical clinical applications. The primary risks associated with the use of diagnostic ultrasound are the risks of interpretation errors.
Computed Tomography
Computed tomography (CT) is the workhorse imaging technology in contemporary emergency medicine and trauma care including vascular trauma. Multidetector CT (MDCT) with high-speed helical scanning has reduced imaging times to minutes; and the availability of CT scanning has become nearly ubiquitous, even in forward locations on the battlefield. Data from a U.S. survey of nearly 100 million patients who underwent CT scanning in emergency departments (EDs) found CT use increased by 330% between 1996 and 2007.
The best application of CT scanning in trauma is controversial. In most institutions CT is routine for evaluation of injuries to the brain, face, chest, abdomen, and pelvis, as well as other skeletal injuries. However, recently some have recommended more targeted use to avoid unnecessary health-care costs and radiation-exposure risks. At the other end of the spectrum, some advocate routine single-pass, whole-body CT scanning (i.e., pan scan) for evaluation of those patients having sustained significant injury. From the standpoint of vascular trauma, contrast enhanced CT angiography can reliably confirm and characterize clinically evident problems (e.g., occluded extremity vessel with ischemia) and can also detect subclinical injuries (e.g., asymptotic or minor TAI).
Magnetic resonance imaging (MRI) and magnetic resonance angiography (MRA) may be used in some centers as alternatives to CT and CTA, with some potential advantages from avoiding CT artifacts and radiation exposure. However, MRI is not as readily available and has slower image acquisition; and there are more contraindications to its use, including the presence of metallic implants. Also of practical importance, there are many pieces of medical equipment that may not be compatible with use in the presence of a strong magnetic field.
Indications
The indications for CTA for evaluation of the injured patient are broad. Any patient with a known or suspected vascular injury may be a candidate for CTA, though CTA may not be needed if there is sufficient information from clinical assessment (i.e., hard signs of vascular injury). Additionally, other noninvasive imaging modalities may suffice to make the correct diagnosis of vascular injury and may allow for appropriate management.
Indications for CTA of the head and neck after blunt or penetrating trauma include unexplained or incongruous central or lateralizing neurological deficit. This modality is also indicated for complex facial or mandible fractures, penetrating injuries to the neck (zones I, II, and III), cervical spine or spinal cord injuries, and thoracic injuries. CTA for suspected arterial injuries of the neck without initial indications for immediate operation allows characterization of lesions such as partial or complete occlusion, pseudoaneurysm, intimal flap, dissection, and arteriovenous fistula. With the same examination, CT provides information about the cervical soft tissues, the aerodigestive tract, the spinal canal, and the spinal cord. In cases of penetrating injuries, the bullet or fragment trajectories and the locations of fragments may be assessed ( Fig. 6-6 ).

The most common indication for thoracic CTA for trauma is evaluation of known or suspected TAI, usually in the setting of high-energy deceleration injuries. CTA of the chest is also useful in the setting of penetrating trauma with possible great vessel injury. Chest x-ray findings that suggest TAI or other vascular injury include a widened mediastinum, an apical cap, and a displacement of the trachea, left main bronchus, or nasogastric tube. However, a normal chest x-ray does not exclude TAI. Blunt TAI has been characterized based on findings on CTA as follows: type I, intimal flap; type II, intramural hematoma; type III, pseudoaneurysm; and type IV, aortic disruption. This grading scheme separates those patients who may be managed conservatively (type I) from those with more-severe injuries (types II, III, and IV) that require operative or endovascular treatment ( Fig. 6-7 ).

CT scanning of the abdomen and pelvis is an established modality for the evaluation of patients after blunt trauma. Standard imaging protocols are often employed, but contrast-enhanced CTA provides additional information for the evaluation of suspected vascular injuries that may not have been clinically apparent or that lead to an immediate operation. For example, the finding of a blunt abdominal aortic dissection or of a visceral branch on CTA may not have been associated with positive physical examination findings or clinical symptoms. Most patients with truncal vascular injuries have associated spine or spinal cord injury or injury to the viscera or solid organs.
The indications for CTA for the evaluation of extremity trauma are similar to those for conventional arteriography. However, the availability and diagnostic accuracy of CTA has made it the imaging modality of choice in most centers. Adding extremity CTA to an already-planned scan of the chest, abdomen, and pelvis adds little time or contrast but does provide detailed information that may be particularly useful in the polytrauma patient ( Fig. 6-8 ). Studies of CTA in the evaluation of extremity vascular trauma have demonstrated high rates of sensitivity and specificity (90% to 100%).

Preparation
Reliable intravenous access is needed for contrast administration. As previously stated, limiting the volume of iodinated contrast agents (by limiting multiple diagnostic imaging procedures) and avoiding hypovolemia are recommended to reduce CIN risk.
Pitfalls and Danger Points
Risks associated with CTA are similar to conventional arteriography, but without the risks of arterial catheterization. CTA hazards include the following:
- •
Contrast induced nephropathy (CIN)
- •
Anaphylactic reaction to contrast
- •
Contrast extravasation outside of the vein
- •
Ionizing radiation
- •
Venous injuries may be missed if single-phase acquisition is performed.
- •
Diagnostic (interpretation) errors
- •
Imaging limitations due to artifact
- •
Movement of the patient from a treatment area to the CT imaging suite
From a population-based perspective, the risk of radiation exposure from diagnostic CT scans is considerable. However, on an individual basis, radiation-associated risks are low and usually not major considerations in the context of a potentially life- or limb-threatening vascular injury. The most practical way to keep radiation exposures as low as reasonably achievable in trauma care is to perform only those studies needed for patient management. Of note, overall radiation dose may be decreased with the initial performance of a quality CTA, as its high diagnostic yield may obviate the need for other radiographic studies. Technology advances (detector design, image-processing systems) have decreased radiation dose and other procedure-specific changes (adjustments in tube current [mA], tube potential [kVp], gantry rotation time, helical pitch) can further limit exposure. Other pitfalls of CTA include sources of artifact that can degrade imagine quality or one’s ability to interpret the image.
In evaluation of the trauma patient, motion can also degrade image quality, creating black or white bands, dark spots, loss of resolution, or anatomic distortion. Strategies to reduce motion artifact include fast scanning, gating (e.g., to reduce motion artifact from the cardiac cycle), tube alignment, corrective reconstruction, and postprocessing techniques. The presence of high-density foreign materials can also be problematic. Metal can create streak artifacts by causing the detectors to operate in a nonlinear response region, and even small fragments can create a star-pattern artifact. Patient body habitus also affects image quality with more image distortion occurring in larger patients.
Performance of a CT scan relies on geometric precision and measurement quality. Inaccurate geometry, inaccurate alignment of the x-ray tube with the detectors, or incorrect data can produce artifacts and blurring that limit spatial resolution. Detector calibration errors and balance can also occur detracting from image quality. Artifacts caused by equipment malfunction can be eliminated by repair or preventive maintenance.
Beam attenuation is proportional to the average attenuation coefficient in each volume element (voxel). Resolution may be degraded when tissues with different absorption densities are in the same voxel. Partial-volume effects are minimized by the use of thin sections or “cuts” and by the selection of a section that lies in the center of the object of interest for attenuation measurements. Beam-hardening artifacts result from preferential absorption of low-energy photons from the x-ray beam. The effect may be pronounced in areas of high attenuation, such as bone.
Specific to CTA, inadequate vascular opacification due to poor delivery of contrast or circulatory delays in patients in shock may render CT angiography (arterial or venous) nondiagnostic. Timing delays may be most problematic for CTA of distal extremity vessels or for more central venous structures.
Finally, as experienced clinicians are aware, transporting critically ill or injured patients to an imaging suite is associated with risks. Transport of patients form the resuscitation, OR, or intensive care unit may require suspension of specific therapies and may initiate a transition of care. Additionally, movement of a seriously injured patient to the CT scan area and bed may result in interruption of cardiovascular monitoring and may increase the risk of displacement of lines or tubes.
Strategy
CT scanning has become nearly ubiquitous in the management of the severely injured patient, with demonstrated efficacy for detection of occult injuries and characterization of known injuries. Standard imaging protocols detect most vascular injuries, but dedicated CT angiographic studies are often needed to better characterize some patterns of vascular trauma, especially those to medium and smaller-sized vessels.
Technique
CT angiography is performed in targeted regions with IV contrast infusion. A typical contrast bolus volume is 100 mL with an infusion rate of 4 mL/s. The imaging delay is typically estimated, but most systems will time the arterial phase acquisition with bolus tracking, starting when the contrast arrives at a preselected region of interest. A technologist performs CT scans, typically with predefined protocols. The technologist positions the patient, administers contrast materials, prepares and operates the CT scan equipment, then sends image data in Digital Imaging and Communications in Medicine (DICOM) format to the picture archiving and communication system (PACS).
Conventional CT displays show the density of the imaged tissue (the degree to which x-rays are attenuated) in gray scale. CT densities are measured in Hounsfield units (HU), which range from −1024 to +3071. As the human eye can discern only 30 to 40 gray scale levels, the image display can be varied to include HU ranges across a small or broad window, centered on a particular level of interest. Modern MDCT scanners have isotropic resolution, with all three dimensions of the individual image volumes (voxels) being the same (X = Y = Z). Because of this, the CT dataset can be considered a three dimensional (3-D) representation of the image volume scanned, and these data can be displayed in several ways. Postprocessing of the volumetric imaging data from CTA can greatly facilitate image interpretation.
Some postprocessing may be done automatically, but technologists, radiologists, and other clinicians are able to manipulate the dataset to yield the views and projections of specific diagnostic interest. Postprocessing techniques can create 2-D or 3-D images. The use of dual energy levels (kVp) during imaging can facilitate removal of bone from images or can help distinguish calcium from contrast-enhanced blood. The selection of the thickness of the imaging slice through the imaged volume can be selected (though it cannot be thinner than the collimation width used for imaging). Thin-slice reconstructions have better edge definition, better high-contrast resolution, and fewer partial-volume artifacts at the cost of greater noise and poorer low-contrast resolution.
Two-dimensional CTA postprocessing techniques include multiplanar reformatting (MPR) of images, as well as curved reformatting. MPR displays volumetric data in orthogonal planes (axial, sagittal, coronal), as well as in oblique planes selected and manipulated by the user. Samples through the volume dataset can be thin slices or thick slabs. Curved reformats (CR) are used to view vessels over their entire course, which facilitates evaluation of segment patency or stenosis.
Three-dimensional postprocessing includes maximum intensity projections (MIP) and surface shaded volume rendering (VR). With MIP displays, the highest attenuation along the line projected through the image is brought forward. MIP effectively displays structures with high HU, such as contrast-filled vessels. VR images are helpful for understanding complex structural relationships, and many surgeons prefer this view for operative planning. No additional information is provided by VR. In fact, some information may be lost as vessels without sufficient contrast may not be displayed. Smaller imaging increments (with overlap of adjacent slice acquisition) provide for better 3-D rendering.
CTA signs of arterial trauma in the extremities include extravasation of contrast (i.e., pseudoaneurysm), narrowing (i.e., stenosis), loss of opacification (i.e., occlusion), and rapid venous contrast (i.e., arteriovenous fistula).
Postexamination Care
There are few specific concerns after CTA, although hypovolemia should be avoided. Urine output and renal function should be monitored.
Complications
CT is safe, is noninvasive, and is associated with few direct risks of complications. Complications of CT are primarily those associated with contrast administration (extravasation, renal failure, allergic-type reactions). Other risks associated with CT CTA are those associated with errors in image interpretation.
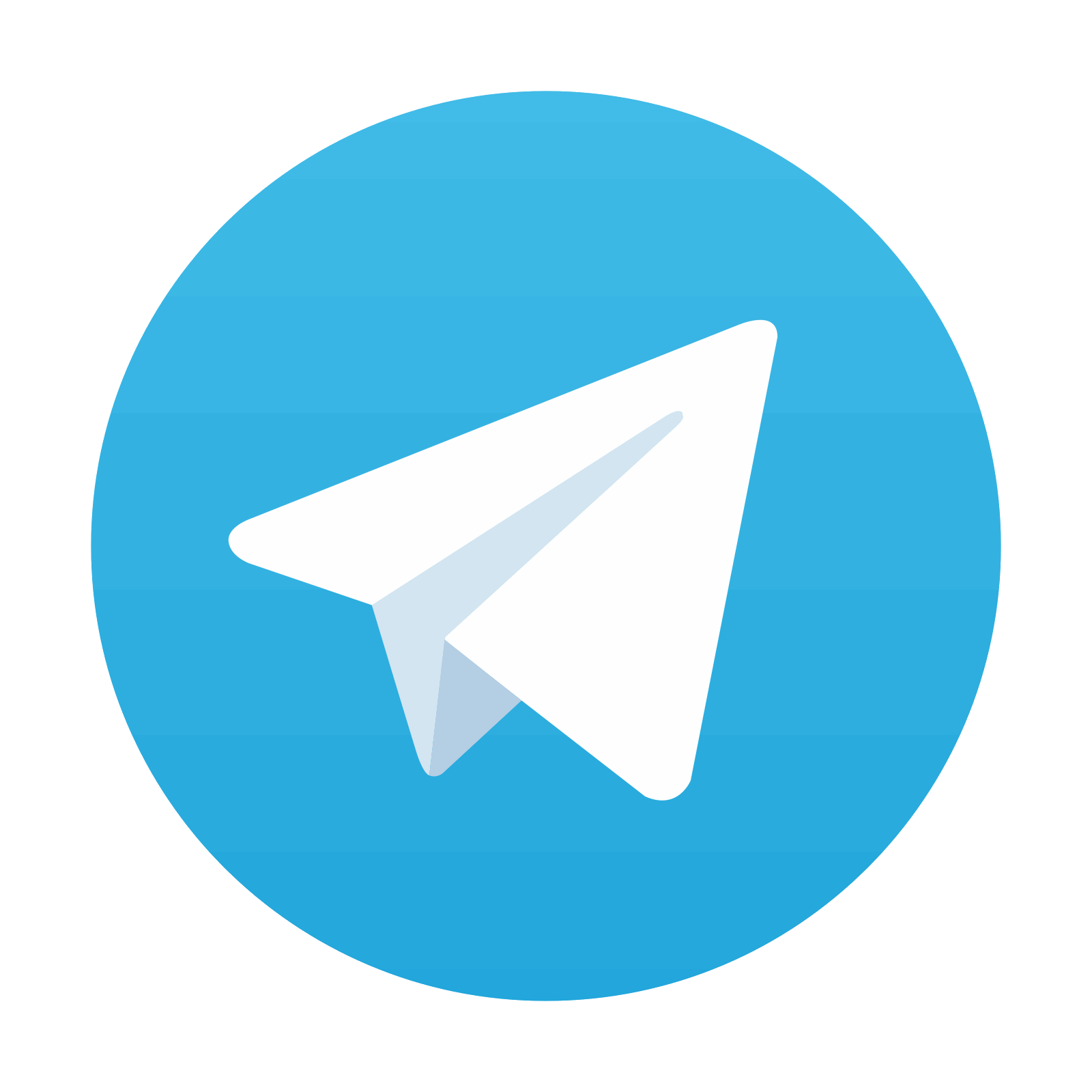
Stay updated, free articles. Join our Telegram channel

Full access? Get Clinical Tree
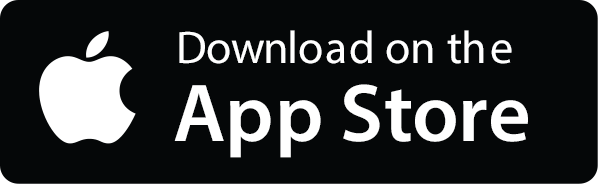
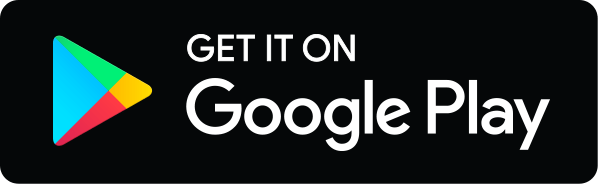