Introduction
The field of transcatheter structural heart interventions has grown tremendously in recent years. Because operators are not able to directly visualize the cardiac anatomy during percutaneous procedures, live imaging is a critical component of safely and successfully performing these procedures. Interventional echocardiography has thus evolved in tandem with the growth of structural heart procedures. Echocardiography has become an important part of preprocedural planning, procedural guidance, and postprocedural assessment. Commonly performed procedures include transcatheter aortic valve replacement (TAVR), transcatheter valve-in-valve implantation or valve-in-ring implantation, closure of paravalvular regurgitation (PVR), edge-to-edge mitral valve repair (TMVR), and left atrial appendage occlusion (LAAO), among others. Intensive interest and ongoing trials are currently evaluating transcatheter options to treat mitral regurgitation (MR) with transcatheter mitral valve replacement (valve in native annulus) or alternative transcatheter mitral valve repair devices, as well as new devices to treat tricuspid regurgitation. These new devices have the potential to provide treatment options for patients deemed to have a high or prohibitive risk for conventional open surgery.
General principles
A number of challenges are unique to interventional echocardiography that are not commonly encountered in other areas of the echocardiography practice. Procedural success relies on the echocardiographer’s ability to track catheters and devices in the heart to ensure safe passage and avoid potential complications. This can be challenging due to movement of catheters related to respiration, cardiac motion, and device manipulation by the operator. Furthermore, the devices themselves commonly cause an element of acoustic shadowing, which can make it more challenging to visualize normal cardiac structures. Finally, devices and catheters often distort normal cardiac anatomy, which can make it challenging to assess the procedural results. When three-dimensional echocardiographic (3DE) guidance is required, blooming and other artifacts specific to 3DE may make it difficult to distinguish between wires and catheters.
Effective communication among the procedural team is a critical component of procedural success and begins when the imager relays important preprocedural findings to the interventionalists. A common language based on fixed spatial anatomic reference landmarks is essential for effective communication. As the procedure begins, it is important that the echocardiographer be familiar with the technical aspects of the procedure being performed, including the different types of catheters/sheaths/wires and dilators used, to anticipate the next steps and potential complications.
Effective procedural imaging incorporates a variety of imaging modes, including 2D, 3D, color Doppler, and spectral Doppler imaging. Frequently, a multiparametric approach is necessary for procedural success. Compared with two-dimensional echocardiography (2DE), 3DE provides a panoramic view of anatomic structures from a single imaging window, which in turn provides contextual anatomic guidance during transcatheter interventions, but the temporal (frame rate) and spatial resolutions are lower.
Transcatheter aortic valve replacement
There is considerable practice variation in utilization of transthoracic vs. transesophageal echocardiography (TEE) for TAVR periprocedural imaging in our current clinical practice, we utilize TTE in the majority of TAVR cases, with TEE used where a good image cannot be attained with TTE and for all transapical TAVRs. To effectively use TTE for TAVR guidance, one must have adequate image quality to definitively assess for PVR and other relevant periprocedural complications. In cases where TTE quality is inadequate, there should ideally be the ability to convert to TEE for more definitive assessment.
Preprocedural echocardiographic assessment
TTE remains the workhorse for diagnosing aortic stenosis and quantifying the severity. Additionally, echocardiography is useful in defining the aortic anatomy (e.g., bicuspid vs. tricuspid), quantifying biventricular systolic function and diastolic function, screening for pulmonary hypertension and preexisting pericardial effusion, and evaluating for coexistent valve disease that could affect patient management. 2DE imaging is also useful to screen for asymmetric or bulky calcification, which can be problematic at the time of valve implantation. This requires the use of orthogonal and off-axis views. Significant asymmetric valve calcification can increase the risk of PVR postprocedure. Calcification that extends into the aortomitral continuity can also be problematic at the time of valve deployment and can increase the risk of subannular rupture. Strategies for choice of valve to best address these anatomic findings are detailed in Chapters 8 and 9 . Frequently, the added depth perspective of 3DE can be helpful to visualize the extent and location of subaortic annular calcification ( Fig. 3.1 ).

At most institutions, cardiac computed tomography (CT) is the primary method to quantify aortic annular size before valve implantation. Because of the irregular and frequently ellipsoid shape of the aortic annulus, a single linear dimension of the aortic annulus may not accurately represent the true annular area or perimeter. 3D imaging modalities are superior in quantifying the aortic annular size and reducing the risk of PVR postprocedure. In experienced hands, 3D TEE using multiplanar reconstruction is equivalent to CT in sizing the aortic annulus. , This method can be utilized in situations where contrast administration is contraindicated, when the CT is equivocal, or when the aortic annulus is on the cusp of two valve sizes ( Fig. 3.2 ). With each method, the aortic annular size is measured at midsystole at the nadir of the aortic valve leaflets. 2DE and 3D TEE can be useful to quantify the sinus of Valsalva and sinotubular junction dimensions, which, if small, also increase the risk of aortic root rupture and coronary artery obstruction.

Patients with coronary artery ostia in close proximity to the aortic annulus (<10 mm) are at increased risk of coronary artery obstruction. Measurement of ostial heights above the annulus at most institutions is done with CT preprocedurally, and this is detailed in later chapters. However, ostial heights can also be measured with TEE. The left main coronary artery is typically seen best with 3D TEE using multiplanar reconstruction. Because of its spatial relationship with the aortic annulus, it is difficult to measure by 2D imaging ( Fig. 3.3 ). The ostium of the right coronary artery, in contrast, is typically best seen anteriorly in a midesophageal long-axis view of the aortic root with a multiplane transducer angle of 120 to 150 degrees (see Fig. 3.3 ). A comprehensive assessment of left ventricular function with attention to regional wall motion is important before the procedure to serve as a baseline. Intraprocedural obstruction of the coronary arteries results in significant changes in left ventricular function with regional abnormalities that fit a coronary distribution.

Additional preprocedural considerations that must be documented include the presence and size of any preexisting pericardial effusion. Acute procedure-related pericardial effusion is rare but can occur most commonly due to laceration of the left ventricular myocardium by a guidewire or the delivery system. Second, the presence and severity of MR. Overly deep implantation of a transcatheter aortic valve can disrupt the normal motion of the anterior mitral leaflet and can result in increased MR. And third, prominent subaortic septal hypertrophy because it can interfere with proper valve positioning and also predisposes to left ventricular outflow tract (LVOT) obstruction after valve implantation.
Postimplantation echocardiographic assessment
The immediate post-TAVR implantation imaging is primarily focused on assessing prosthetic valve function and potential procedure-related complications. These include the presence and severity of PVR, mitral valve dysfunction, aortic root trauma/aortic dissection, abnormal prosthetic leaflet motion, valve thrombosis, and coronary artery obstruction. In patients with suboptimal or nondiagnostic TTE, TEE is needed.
After valve deployment, one of the most important first steps is to evaluate the TAVR valve position and stent expansion. The TAVR valve position is best interrogated in echocardiographic long-axis views ( Fig. 3.4 ). A valve that is implanted too high may risk trauma to the sinotubular junction or valve embolization, and there is increased risk of PVR. A valve that is implanted too low increases the risk of PVR, procedure-related conduction disturbance, disruption of mitral valve function, and overhang of the calcified native aortic valve leaflets, which can interfere with prosthetic valve function. Optimal initial positioning of an Edwards Sapien 3 balloon-expandable valve is “50-50,” with 50% of the valve below the annulus and 50% above it. During balloon expansion, however, the Sapien 3 valve typically shortens from the distal end, and the final positioning is optimally 2 to 4 mm distal to the aortic annulus or 80/20 or 90/10 aortic/ventricular. Normal positioning for the distal edge of the Medtronic CoreValve Evolut self-expanding valve is 3 to 5 mm below the aortic annulus.

Stent expansion is best visualized in short-axis views of the prosthesis. In a true short-axis view of the stent frame, it should appear round. An oval-appearing stent indicates underexpansion, and there is heightened risk of PVR ( Fig. 3.5 ). For current-generation self-expanding valves, the prosthetic leaflets sit supra-annular (superior to the native aortic annulus) and therefore underexpansion of the frame at the annular level does not typically affect prosthetic leaflet function. However, with balloon-expandable valves, the prosthetic leaflets typically sit at the level of the native aortic annulus, and underexpansion at this level can interfere with normal leaflet motion and result in significant transprosthesis regurgitation.

In the absence of acute procedural complications, the next most important assessment is interrogation for PVR, which is challenging and requires an integrated, multiparametric approach. Published guidelines and recommendations largely agree on grading, but differ in recommending a 3-point grading scheme (mild, moderate, severe) or a 6-point grading scheme (none/trace, mild, mild-moderate, moderate, moderate-severe, severe). , At our institution we use a 6-point grading scheme largely in concordance with the noted published guidelines and recommendations.
The circumferential extent of PVR viewed in the short axis is an important component of this assessment. Less than 10% circumference is considered mild, 10% to 20% is considered mild-moderate, 20% to 30% is considered moderate, and >30% is consistent with moderate-severe or severe ( Fig. 3.6 ). It is important to remember that acoustic shadowing from the valve stent and native calcification commonly result in underestimation of PVR when viewed at the level of the native annulus, and care must be taken to interrogate for PVR at the ventricular edge of the stent (at the skirt level) ( Fig. 3.7 ). Because of acoustic shadowing, one must also be cognizant that posterior jets may be underappreciated by TTE from the parasternal window, and anterior jets can potentially be missed with TEE from the midesophageal imaging window. Obtaining “sweeps” through multiple echocardiographic imaging planes can be helpful to be sure occult PVR is not missed. Correlation with invasive hemodynamics (measurement of left ventricular end diastolic pressure [LVEDP] and diastolic aortic pressure) will also guide the procedural team as to whether there is undetected PVR that needs more careful echocardiographic assessment. To avoid acoustic shadowing, it is also critically important that PVR be interrogated from multiple imaging windows, including the parasternal, apical, and subcostal windows, by TTE and both the midesophageal and transgastric views by TEE.


In addition to circumferential extent of PVR, there are a number of other important echocardiographic findings that correlate with the severity of aortic regurgitation (AR). A dense AR continuous wave Doppler signal generally correlates with moderate or greater regurgitation, whereas a short pressure half-time, <200 msec, is thought to generally indicate severe regurgitation, although these parameters lack thorough validation in the TAVR setting ( Fig. 3.8 ). Holodiastolic reversals in the descending thoracic aorta by pulse wave Doppler assessment, particularly when the end-diastolic velocity is >20 cm/sec, also indicate significant AR (see Fig. 3.8 ). Additionally, multiple other qualitative or semiquantitative parameters may indicate significant PVR; a jet path that is visible along the stent frame, or a jet origin diameter >30% the diameter of the LVOT, and a visible flow convergence on the aortic side of the valve are all suggestive of moderate or greater PVR.

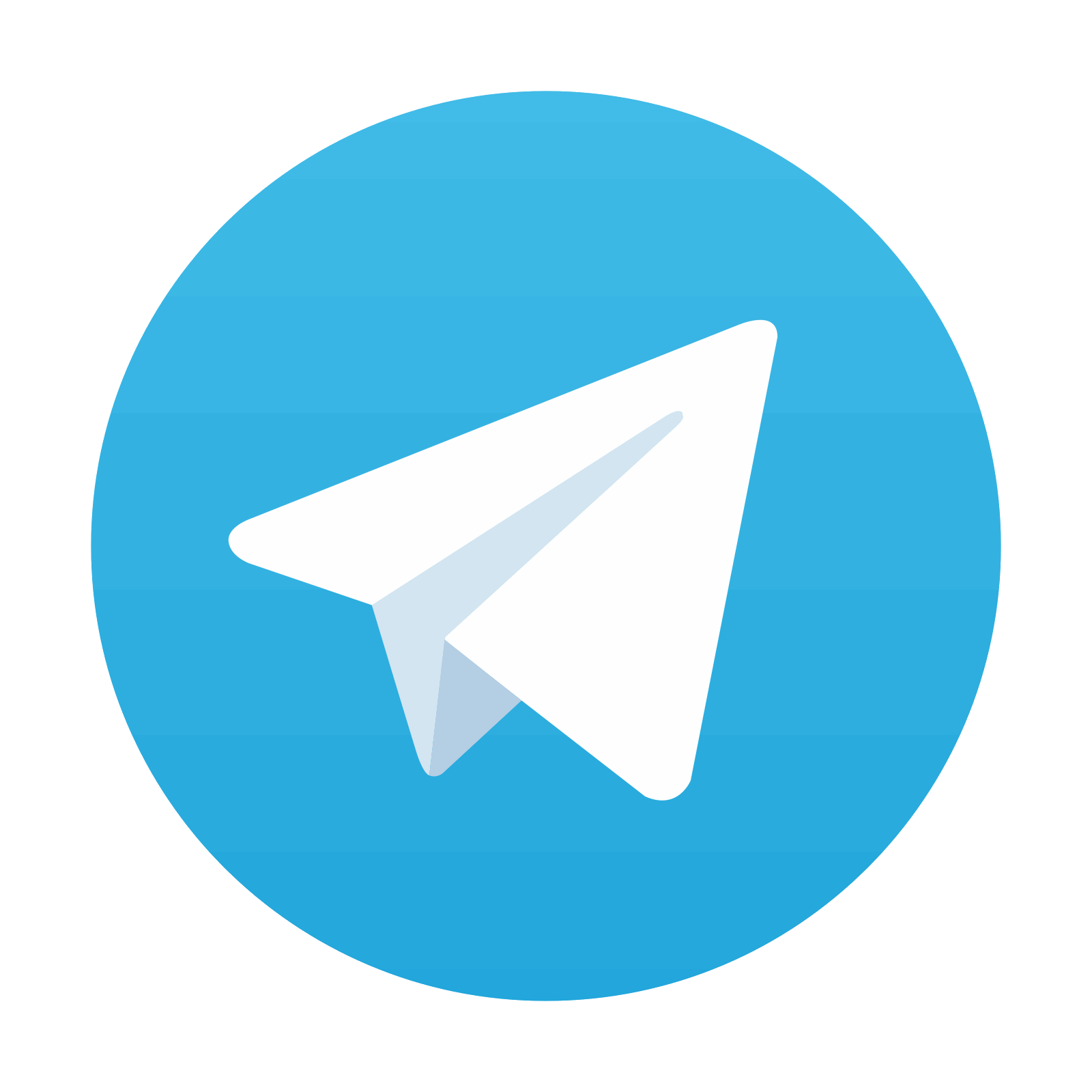
Stay updated, free articles. Join our Telegram channel

Full access? Get Clinical Tree
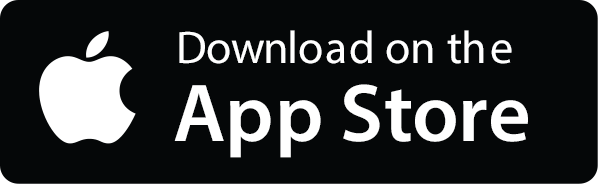
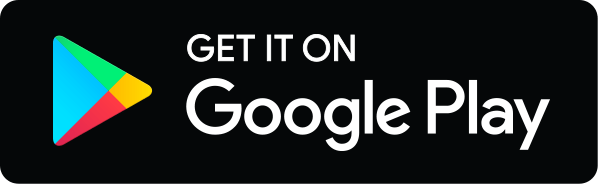
