Women
Men
Normal
Mildly abnormal
Moderately abnormal
Severely abnormal
Normal
Mildly abnormal
Moderately abnormal
Severely abnormal
Mass (g)
66–150
151–171
172–182
≥ 183
96–200
201–227
228–254
≥255
Mass/BSA (g/m2)
44–88
89–100
101–112
≥113
50–102
103–116
117–130
≥131
EDV/BSA (ml/m2)
35–75
76–86
87–96
≥97
35–75
76–86
87–96
≥97
ESV/BSA (ml/m2)
12–30
31–36
37–42
≥43
12–30
31–36
37–42
≥43
EDD (cm)
3.9–5.3
5.4–5.7
5.8–6.1
≥6.2
4.2–5.9
6.0–6.3
6.4–6.8
≥6.9
EF (%)
≥55
45–54
30–44
<30
≥55
45–54
30–44
<30
Evaluation of the diastolic function is also fundamental in the diagnosis of the type of HF— mainly in patients with symptoms and preserved LVEF. A variety of echocardiographic techniques can determine the left atrial pressure and left ventricular end-diastolic pressure, but none of them alone is sufficiently accurate or reproducible to make a definitive diagnosis of LV diastolic dysfunction [2]. We can have good prognostic information from those measures [13], but the association of the relevant 2D–Echo and Doppler data are recommended for the diagnostic evaluation. Depending on the association of findings, the function could be graded according to severity in “abnormal relaxation,” “pseudonormal,” and “restrictive.” Mild diastolic dysfunction—“abnormal relaxation”—can be detected via decrease in early diastolic flow velocity and greater reliance on atrial contraction to fill the LV. Moderate diastolic dysfunction—“pseudonormal”—reflects an increasing left atrial pressure at the onset of diastole and an increase in early diastolic flow velocity to a level near that of normal filing. Severe diastolic dysfunction—“restrictive filling”—occurs when left atrial pressure is further elevated, with quick early diastolic flow and rapid equalization between LV and LA pressures in early diastole [14]. This characterization can be done based in the information from the mitral inflow Doppler, Tissue Doppler of mitral annular motion and the pulmonary venous flow [15] and it is related to adverse outcomes [16].
The cardiac dyssynchrony assessment is other potential use of echocardiography. From clinical and experimental studies, we can subdivide dyssynchrony into three levels: atrioventricular, interventricular and intra-(left) ventricular. Of those, the last one seems to be the best to predict cardiac resynchronization therapy (CRT) response [17]. The most commonly used techniques include M-mode echocardiography, TDI, strain imaging, and the 3D–Echo. Several small and single-center studies show good correlations between markers of dyssynchrony and response to CRT, but the largest prospective and multicenter study designed for this evaluation—the PROSPECT (Predictors of Response to CRT)—has shown modest results [17, 18]. In this sense, the guidelines for CRT treatment indication do not include any dyssynchrony evaluation by imaging. Nevertheless, recent improvements in the acquisition and analysis of the echo images contributed to better reproducibility of the parameters, so perhaps new studies can cast more light in this issue.
Some new echocardiographic techniques like the 3D–Echo and the “speckle tracking” (ST) are opening new horizons in cardiac non-invasive evaluation. Development of new matrix array transducers that acquire full volume data in real time allow the 3D–echo to get rid of off-line image reconstructions, and therefore made this modality more suitable for the clinical use (Fig. 3.1). The great advantage is the calculation of volumes and diameters of the heart without geometric assumptions. Some studies have shown that 3D–Echo is highly accurate and reproducible for assessing LV volumes and mass when compared to cardiac MRI [19, 20]. The ST is also one of the most promising new techniques, because it can measure the global and regional strains without a dependence of angle acquisition, different of what happens with the TDI. This modality relies on algorithms that can identify multiple unique patterns of echocardiographic pixel intensity and then automatically track them along the cardiac cycle, providing their movement in plane (strain) and by the time (strain rate) (Fig. 3.2). Several small studies have shown correlations with strain parameters by ST and tissue alteration, such as fibrosis, and with markers of subtle myocardial disease [21]. For both 3D–Echo and ST, however, we still need information from randomized trials and epidemiologic studies.



Fig. 3.1
Example of a 3D echocardiography acquisition. With full volume data acquisition in real time, it is possible to obtain cines of various planes at the same time. (a) 4 chamber view; (b) 2 chamber view; (c–e) short axis views. It is remarkable to notice the ability to observe more than one plane at the same time

Fig. 3.2
Representation of the strain parameters obtained from “speckle tracking” technique by echocardiography. (a) Longitudinal strain demonstrates the shortening of the wall segment as the base moves towards the base in systole; (b) Circumferential strain reflects the reduction in circumference of the heart in systole (This is the most stable strain parameter); (c) Radial strain reflects the thickening of a wall segment in systole; (d) Twist is the difference in degrees between apical and basal rotation. In the inferior left there is an example of a radial strain acquisition with the curves
Cardiac Magnetic Resonance Imaging
Cardiac Magnetic Resonance (CMR) is considered one of the most useful techniques in the evaluation of suspected HF. It can provide all the anatomical and functional parameters that echocardiography can provide—with additional high spatial resolution and tissue characterization. Furthermore, CMR is regarded as the gold standard with respect to accuracy and reproducibility of volumes, mass, and wall motion [2]. Its ability to have good image quality in most exams makes it the exam of choice for functional evaluation when 2D–Echo is non-diagnostic.
A general CMR exam comprises a complete segmentation of the heart, with a vertical and horizontal long axis of the LV (2 and 3 chamber views), a stack of 8–12 slices in the short axis and the left ventricle outflow track (3 chamber view) (Fig. 3.3).


Fig. 3.3
General cardiac magnetic resonance (CMR) imaging segmentation. A CMR exam comprehends a complete segmentation of the heart, with a vertical and horizontal long axis of the LV (2 and 3 chamber views), a stack of 8–12 slices in the short axis (here with an example of basal, mid and apex) and the left ventricle outflow track (3 chamber view)
All the volumes and LV mass are usually calculated using the modified Simpson’s rule in the short axis stack, with the big advantage of the absence of geometrical assumptions for those calculations (Table 3.2). This fact is especially important given the chamber dilatation and remodeling that often accompany HF. Nowadays, the most commonly used sequence for cine imaging is a balanced steady-state free precession (SSFP) with retrospective ECG gating [22], which has good spatial resolution and contrast between the ventricular wall, the blood and adjacent structures. The disadvantage of this sequence is the necessity of repeatedly breath-holds, which can be difficult to achieve in HF patients. Because of that, the use of real time acquisition of cine imaging is becoming more available. This sequence provides diagnostic images (with lower spatial resolution when compared to SSFP) acquired in 3–4 s, with no breath-hold required [23, 24], and can be considered a good option in patients who cannot perform this maneuver. Completing the function assessment, phase-contrast images can be obtained for the measurement of valve flow, intra-cardiac flow, and even myocardial tissue motion [24]. This sequence relies on phase shifting related to the motion (or flow) of the blood (or myocardium) when compared to the stationary structures, which provides a detailed pixel map where intensity is directly related to the velocity and the sign (positive or negative) indicates the direction (Fig. 3.4). Myocardial tagging is a powerful tool to evaluate the global and regional myocardial function with detailed and comprehensive examination of intra-myocardial motion and deformation; therefore, it is the reference technique for evaluating the tissue strains [25]. Besides the good sensibility to the detection of subtle abnormalities, the role of the tagging sequences in regular clinical use remains uncertain [24].

Table 3.2
Reference values for left and right ventricular function and dimension by cardiac MRI in adults [42]
Women | Men | |||
---|---|---|---|---|
<35 years | ≥35 years | <35 years | ≥35 years | |
Left ventricle | ||||
Mass (g) | 52–132 | 54–130 | 89–173 | 74–166 |
Mass/BSA (g/m2) | 35–71 | 34–70 | 47–87 | 42–78 |
EDV/BSA (ml/m2) | 62–98 | 51–95 | 68–112 | 53–97 |
ESV/BSA (ml/m2) | 13–37 | 11–35 | 16–44 | 10–34 |
EF (%) | 57–81 | 57–81 | 57–77 | 59–83 |
Right ventricle | ||||
EDV/BSA (ml/m2) | 67–111 | 42–118 | 74–134 | 67–111 |
ESV/BSA (ml/m2) | 25–45 | 6–54 | 26–62 | 20–48 |
EF (%) | 55–67 | 50–78 | 47–67 | 49–73 |

Fig. 3.4
Flow quantification of the ascending aorta with phase contrast imaging by Cardiac magnetic resonance imaging. In this example, the ROI (red) is placed in the ascending aorta and can observed in the ECG-gated cine phase contrast magnitude (left) and phase (right) images during early diastole. On phase images, the bright signal intensity corresponds to cephalad flow and the black signal to caudal flow. A plot of mean flow through the cardiac cycle in the ascending aorta can be then generated
In addition to the function analysis, tissue characterization has a key role in the evaluation of HF patient not only for the diagnosis, but also because of the prognostic information related to its findings. In this sense, several T1- and T2-weighted sequences with or without gadolinium use can be performed to provide information about the composition and perfusion of the myocardial tissue. T2- and T2*-weighted images are generally performed without contrast to bring information about the intrinsic tissue composition, such as water and iron content, and when associated with other techniques can even provide information about the lipid content [27–29]. T1-weighted images are generally used in association with contrast to show its dynamics since the gadolinium makes the T1 times shorter. Late gadolinium enhancement and perfusion images rely on this effect to generate the images. The association of those findings makes the evaluation even more powerful.
Stress perfusion imaging with CMR is an accurate exam to investigate coronary arterial disease. It can be performed using pharmacologic or even physical stress, but the first modality is far more common due the convenience. Dipyridamole or adenosine are used to generate coronary vasodilatation; the first pass of the contrast shows areas of delayed perfusion (darker) that can be related to coronary narrowing. The Dobutamine stress test relies on wall motion abnormalities, the same as the stress echocardiography. Both MRI tests are robust and highly reproducible for the diagnosis of coronary artery disease (CAD) [30, 31].
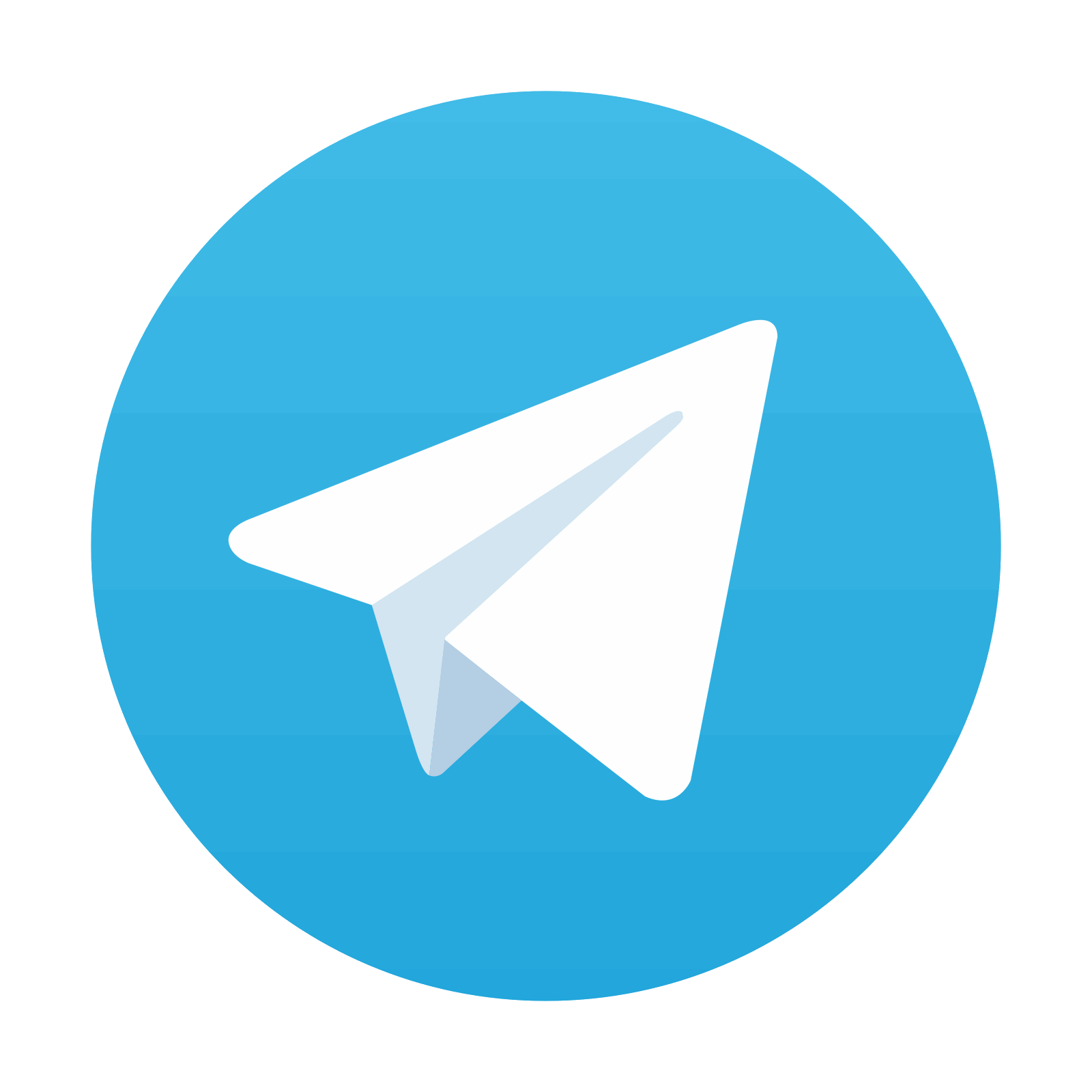
Stay updated, free articles. Join our Telegram channel

Full access? Get Clinical Tree
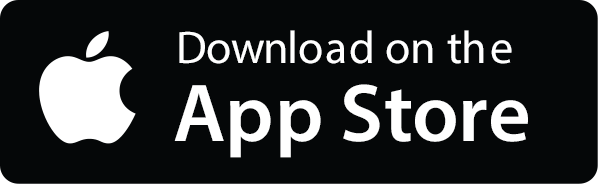
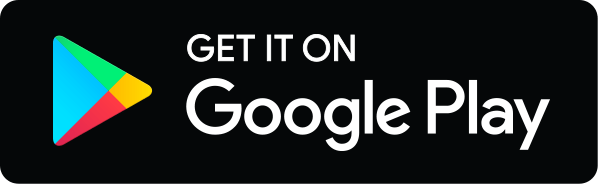