Fig. 9.1
Layout of post-processing of a contrast-enhanced CT with synchronised axial, 3-D rendering imaging, sagittal and coronal multi-planar reconstructions (clockwise)
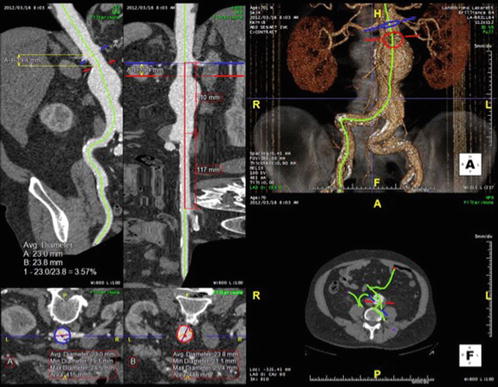
Fig. 9.2
Post-processing of a contrast-enhanced CT scan of a patient with an aortic aneurysm. A centre line of flow is obtained based on the contrast attenuation within the lumen of the aorta (upper right). The workstation provides reconstructions orthogonal to the centre line of flow (bottom left) and allows length measurements along the centre line (upper left)
9.7 Postoperative Imaging
As mentioned before, there has been a trend for replacing CT by ultrasound in the follow-up after standard EVAR of AAA. However, CT is still the golden standard for EVAR postoperative controls, especially in thoracic and more complex EVAR. Postoperative CT aims at measuring aneurysm diameter and identifying endoleaks, migrations, kinks and material fatigue. It should include plain, arterial phase and delayed scans to allow the diagnosis type II endoleaks (from collaterals such as the lumbar arteries) that could otherwise remain unnoticed [44]. Even during follow-up, low-dose and dual-energy CT can be used to reduce radiation, while maintaining the diagnostic outcome [36, 45–48]. Dual-energy CT is also able to produce virtual non-contrast imaging which avoids one scan [49]. Post-processing workstations are also very useful in the follow-up after EVAR allowing the assessment of stent-graft integrity and possible migration [50]. Of particular interest is the fly-through application with virtual angioscopy that provides detailed assessment of the different branches and possible compression or kinking of stents [51]. Moreover, workstations have different methods of measuring the aneurysm size besides the axial diameter. Orthogonal diameters and aneurysm volume seem to increase accuracy in the identification of changes in aneurysm size [11, 52, 53] but demand time-consuming post-processing.
9.8 Magnetic Resonance Imaging (MR)
MR is still used less frequently than CT in the study of aortic aneurysms due to its cost, lower availability and longer time required for scanning. It is however a method widely used for the PAD since it is less susceptible to calcium artefacts and allows imaging of long segments without the same dependency on the varying perfusion as CT (Fig. 9.3) [54, 55]. MR uses magnetic fields and takes advantage of the different relaxation times of the different tissues. Gadolinium contrast agents are commonly used to shorten the T1 (longitudinal) relaxation time and thereby enhance the contrast and shorten the examination time [56]. This contrast agent is also nephrotoxic, but the doses needed for MR angiography are usually below the toxicity levels [57]. On the contrary, gadolinium-based contrast agents have been associated with nephrogenic systemic fibrosis (NSF) in patients with renal insufficiency, and they should be avoided in patients with advanced renal insufficiency [58]. The risks seem nevertheless to be small and should be weighed against the clinical indication for the MR [59]. Unenhanced MR is an upcoming imaging modality that has shown promising results in EVAR planning and diagnosis of PAD [60–64]. This technique may become important in the future for patients with renal insufficiency.
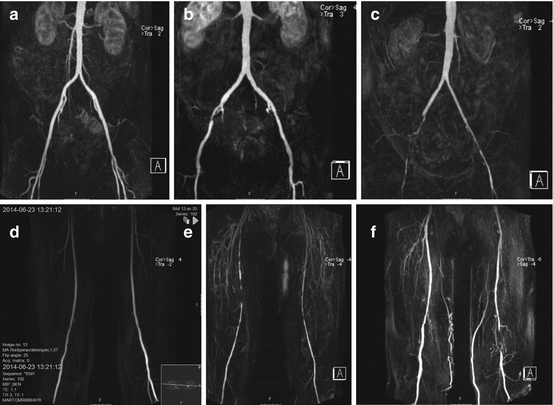
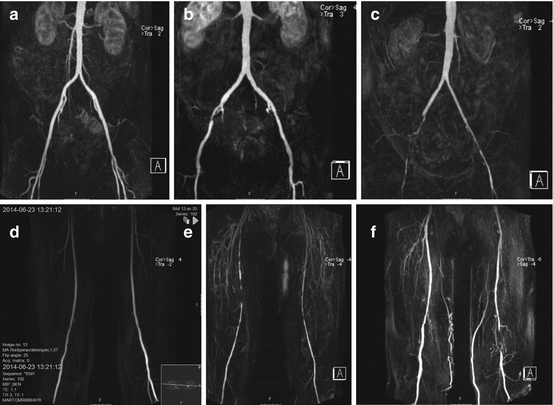
Fig. 9.3
Anterior projections of maximal intensity projections (MIP) of MR angiography for the diagnostic workup of PAD. (a) Aorto-iliac arteries without significant stenosis. (b) Right-sided external iliac stenosis as opposed to the rest of the aorto-iliac segment that is open without significant stenosis. (c) Occluded external iliac artery and significant stenosis in the common femoral artery on the right side. On the left side, there is a distal stenosis of the external iliac artery. (d) Femoro-popliteal arteries without significant stenosis bilaterally. (e) Bilateral multi-segmental stenosis of the superficial femoral arteries. (f) Short occlusion of the left distal superficial femoral artery as opposed the remaining femoro-popliteal arteries
MR angiography images are most commonly analysed as MIP since they are similar to the conventional digital subtraction angiography, i.e. they depict only the lumen. They are nevertheless sensitive to reconstruction artefacts and the raw images should therefore be available. Moreover, raw images enable the identification of the presence of aneurysms and dissections and the measurement of the arterial diameters. Raw images may also identify pathology surrounding the aorta such as inflammatory reactions. MR angiography images can also be post-processed in a similar way as described for CT, enabling detailed morphological analysis and planning of EVAR [65]. MR has also good diagnostic accuracy in the follow-up after EVAR, especially for the identification of type II endoleaks [66–68], in particular when blood-pool contrast agents are used [69, 70]. However, MR use is limited when endografts made of stainless steel or having ferromagnetic markers are used since these cause signal void [71]. Dynamic MR has also shown promising diagnostic possibilities in aortic aneurysms [39, 72–75] and dissections, including assessment of flow dynamics and its implications in future aneurysmatic dilatation of the aorta [76, 77] and false lumen thrombosis after endovascular treatment of aortic dissections [78]. Another interesting application of MR imaging is the identification of areas of increased biological activity in the aneurysm wall by the use of ultrasmall superparamagnetic iron oxide contrast agents (USPIO) that are taken up by macrophages [79–82]. These applications together with the possibility of performing MR-guided endovascular procedures can lead to an increased role of MR aortic imaging in the future [83, 84].
9.9 Digital Subtraction Angiography (DSA)
Angiography is the golden standard for the assessment of PAD but is inappropriate for the evaluation of aneurysms since it outlines only the arterial lumen and is insensitive to the aneurysm diameter, especially if the aneurysm is partially affected by thrombosis or the wall is not heavily calcified. DSA was used in the past to complete the preoperative diagnosis of aortic aneurysms concerning the concomitant presence of PAD in the access vessels and aortic branches, to exclude anatomic variations before open surgical repair or to assist in length measurement before EVAR. This has become obsolete with the advent of 3-D dedicated workstations with rapid and detailed volume rendering of CT and MR imaging [85, 86].
Angiography is therefore currently used during endovascular therapeutic procedures. DSA usually requires iodine intra-arterial contrast. This is nephrotoxic as discussed above and modern angiographic equipment allow radiation adjustments in order to be able to limit the amount of contrast used [87]. Moreover, carbon dioxide can also be used as a contrast medium but has limited application when imaging arteries with dorsal origin and should be avoided above the diaphragm [88, 89].
9.10 Rotation Angiography, Cone Beam CT and Fusion
Modern angiographic equipment with flat digital panels has usually the capability of obtaining high-definition volume-rendering imaging. This may be a digitally subtracted rotational angiography that can be used as overlay reference into the fluoroscopy but has limited diagnostic advantages when compared to the high-definition non-invasive methods described above. The other volume-rendering imaging that can be used is a cone beam CT (CB-CT), which has high spatial resolution, but the acquisition volume is limited by the size of the flat detector and centred in its iso-centre. For these reasons, CB-CT is mainly used intraoperatively and has shown good preliminary results in preoperative anatomic evaluation [90–92] and assessment of the final result [92].
Another technical advancement available in modern angiographic systems is the fusion of the preoperative volume-rendering imaging (usually CT) with the intraoperative fluoroscopy. This is achieved by matching the position of the bony structures on the preoperative CT and intraoperatively. The angiographic software can then use the preoperative CT angiography as an overlay reference in the fluoroscopy screen (Fig. 9.4). The initial results of the application of this technique in EVAR are very promising [93–95], and other applications are being developed with similar outcome [96]. Attention should nevertheless be paid to the potential deviation of the anatomy by the stiff wires and introducers in the aorta and movements related to breathing, heart cycle and posture [97]. There is very recent data on the possibility of gating preoperative MR data, which may allow the overcoming movement deviations [98].
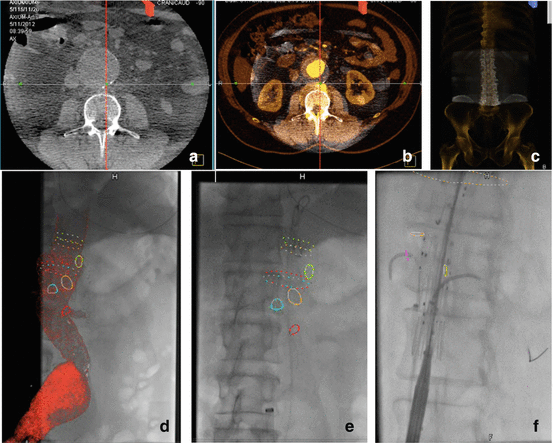
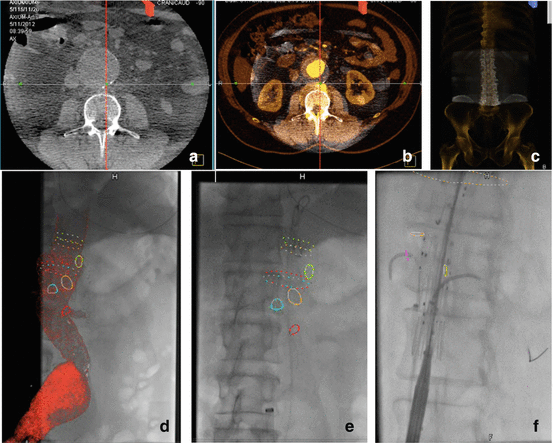
Fig. 9.4
Overlay fusion: (a) rudimentary cone beam CT without contrast obtained with the intraoperative angiographic equipment. (b) Superimposed cone beam CT (whitish shadow within the small circle) on the preoperative high-definition contrast-enhanced CT scan. (c) Superimposed bony reconstructions of the intra- and preoperative CT scans. There is a slight misalignment of the bony structures that will be corrected by the registration process. (d) Transparent 3-D reconstruction of the aortic lumen from the preoperative CT scan superimposed in the intraoperative fluoroscopy. The visceral arteries and the positions of interest in the aorta have been marked with colour rings. (e) Live overlay of the preoperative 3-D information on the intraoperative fluoroscopy. The 3-D volume has been taken away leaving only the rings marking the positions of interest from the preoperative CT (visceral arteries). (f) Example of catheterisation of the renal arteries using only the fusion rings as landmarks and without the need of intraoperative iodine contrast
9.11 Integrated Positron Emission Tomography and Computed Tomography (PET-CT) or Magnetic (MR-PET)
Positron emission tomography (PET) uses fluorine-18 fluorodeoxyglucose (FDG) that is taken up by metabolically active cells such as macrophages in the arterial wall [96, 97] emitting positron and gamma rays upon disintegration that are detected by the camera. CT (and more recently even MR) scanners can be integrated with PET providing, therefore, combined imaging with morphological and functional information. The experiences with PET-CT in pre- and postoperative imaging of aortic aneurysms have been contradictory, and further studies are needed [99–106]. More recent animal studies have shown promising results with the use of PET tracers targeting other components such as integrin, but the value is also awaiting more studies [107].
9.12 Ageing of Arteries: The Role of Arterial Stiffness
Arterial ageing is a process that spans from normal ageing [108] to pathological ageing and the changes described related to atherosclerosis.
In recent years, the interest has increased in arterial stiffness and the underlying arteriosclerosis, as a precursor to the more well-known and well-studied atherosclerosis, with its pathology influenced by genetics, high LDL-cholesterol levels, smoking, hypertension, inflammation and overt type 2 diabetes [109]. In many cases, it is believed that early life programming may cause a susceptibility for this increased tendency for arterial stiffening as well as other aspects of vascular tree, for example, the development of capillaries and the microcirculation. As this process is also related to ageing, it has been proposed that a process of early vascular ageing (EVA) is an early sign of arteriosclerosis (in the media) but linked also to early changes in the endothelial function (intima), haemodynamic changes and the influence of abnormal glucose metabolism and increased inflammation [110–112]. The difference between the concept of arterial ageing and EVA is that the latter also encompasses the smaller arteriolae and the microcirculation, based on the crosstalk between the macro- and microcirculation [112]. EVA is now being extensively studied in different population-based cohorts, both in Europe and in Latin America, but still no general definition has been agreed upon. One way to define EVA could be to use the outliers according to the normal range of c-f PWV, i.e. above the two standard deviations (SD) of the normal distribution of c-f PWV in the European reference group [113]. Another way to describe EVA is based on statistical methods when arterial stiffness (c-f PWV), a central aspect of EVA, is used as the dependent variable in multiple regression analyses and a number of risk markers are used as independent variables, based on data from population-based studies. As the influence of haemodynamic changes and sympathetic nervous system (SNS) stimulation on the arterial tone is substantial, the data are normally adjusted for mean arterial pressure (MAP) and heart rate (HR), the latter as a marker of SNS activity. Such investigations in a population-based study in Malmo, Sweden, have revealed that markers of glucose metabolism and dyslipidaemia (elevated triglycerides, low HDL-cholesterol levels), as well as waist circumference (a marker of active abdominal fat tissue with inflammatory action), are significantly associated with arterial stiffness, but not LDL cholesterol, smoking or cystatin C, a marker of impaired renal function [114]. The findings thus point to different clusters of cardiovascular risk factors involved in development of arteriosclerosis and atherosclerosis, respectively.
Still there is a need to better define EVA in different age groups but also in relation to gender and ethnicity, as well as based on genetic studies for improved classification [115]. Some would argue that EVA is just a construct to cover one example of target organ damage (arterial stiffness) in subjects at high cardiovascular or metabolic risk, and primarily influenced by haemodynamic changes and blood pressure levels. However, the modern genetics of hypertension and blood pressure regulation, based on a global study, could not show any marker on chromosome 13 [116], but exactly on this chromosome, a genetic locus (for the COL4A1 gene, involved in collagen metabolism) was found for arterial stiffness in a study from Sardinia, Italy, with independent replication in another American cohort [117]. This shows that even if arterial stiffness (and EVA) is strongly influenced by the blood pressure load (MAP), HR and SNS activity, there could even exist some other important components (collagen protein synthesis, structure) and vascular risk factors (hyperglycaemia, dyslipidaemia, inflammation) independent of blood pressure regulation. If true, this opens up new possibilities to target these mechanisms of protein/collagen synthesis with new drugs to reduce arterial stiffness.
So far it has been shown that a prolonged control of hypertension will reverse early changes and have a long-term beneficial influence on arterial stiffness with decreasing c-f PWV levels over time, beyond the blood pressure control itself [118]. However, an ongoing randomised controlled study in France (SPARTE) aims to compare a treatment strategy for reduction of arterial stiffness (c-f PWV) by different means, including drugs that specifically influence the renin-angiotensin system, and another treatment strategy (control) to go for implementation of control of the conventional risk factors including blood pressure, as suggested in guidelines [119]. SPARTE is supposed to continue for still a number of years until a sufficient number of cardiovascular endpoints have accumulated to show potential differences in outcomes between the treatment arms. Recruitment is ongoing.
As increased c-f PWV has been documented to be an independent risk marker for future cardiovascular events and total mortality in recent meta-analyses [120, 121], there is a need to target it with multiple risk factor control, aiming for c-f PWV <10 m/s that is the current threshold for increased risk [122].
In summary, the knowledge about morphological changes (imaging) and physiological changes (imaging and haemodynamics) in the arterial wall will make it possible to better understand the double process of arteriosclerosis and atherosclerosis leading to cardiovascular disease manifestations. Current medical and surgical therapies will be expanded in the future for better control of these pathological processes and even for the control of arterial ageing.
References
1.
Hodgson R, McWilliams RG, Simpson A, Gould DA, Brennan JA, Gilling-Smith GL, et al. Migration versus apparent migration: importance of errors due to positioning variation in plain radiographic follow-up of aortic stent-grafts. J Endovasc Ther. 2003;10(5):902–10.PubMed
2.
Murphy M, Hodgson R, Harris PL, McWilliams RG, Hartley DE, Lawrence-Brown MM. Plain radiographic surveillance of abdominal aortic stent-grafts: the Liverpool/Perth protocol. J Endovasc Ther. 2003;10(5):911–2.PubMed
3.
Wilmink AB, Forshaw M, Quick CR, Hubbard CS, Day NE. Accuracy of serial screening for abdominal aortic aneurysms by ultrasound. J Med Screen. 2002;9(3):125–7.PubMed
4.
Wilmink AB, Hubbard CS, Quick CR. Quality of the measurement of the infrarenal aortic diameter by ultrasound. J Med Screen. 1997;4(1):49–53.PubMed
5.
Lindholt JS, Vammen S, Juul S, Henneberg EW, Fasting H. The validity of ultrasonographic scanning as screening method for abdominal aortic aneurysm. Eur J Vasc Endovasc Surg. 1999;17(6):472–5.PubMed
6.
Beales L, Wolstenhulme S, Evans JA, West R, Scott DJ. Reproducibility of ultrasound measurement of the abdominal aorta. Br J Surg. 2011;98(11):1517–25.PubMed
7.
Grondal N, Bramsen MB, Thomsen MD, Rasmussen CB, Lindholt JS. The cardiac cycle is a major contributor to variability in size measurements of abdominal aortic aneurysms by ultrasound. Eur J Vasc Endovasc Surg. 2012;43(1):30–3.PubMed
8.
Hartshorne TC, McCollum CN, Earnshaw JJ, Morris J, Nasim A. Ultrasound measurement of aortic diameter in a national screening programme. Eur J Vasc Endovasc Surg. 2011;42(2):195–9.PubMed
9.
Sprouse I, Richard L, Meier I, George H, LeSar CJ, DeMasi RJ, Sood J, Parent FN, et al. Comparison of abdominal aortic aneurysm diameter measurements obtained with ultrasound and computed tomography: is there a difference? J Vasc Surg. 2003;38(3):466–71.PubMed
10.
Lederle FA, Wilson SE, Johnson GR, Reinke DB, Littooy FN, Acher CW, et al. Variability in measurement of abdominal aortic aneurysms. Abdominal Aortic Aneurysm Detection and Management Veterans Administration Cooperative Study Group. J Vasc Surg. 1995;21(6):945–52.PubMed
11.
Manning BJ, Kristmundsson T, Sonesson B, Resch T. Abdominal aortic aneurysm diameter: a comparison of ultrasound measurements with those from standard and three-dimensional computed tomography reconstruction. J Vasc Surg. 2009;50(2):263–8.PubMed
12.
Moll FL, Powell JT, Fraedrich G, Verzini F, Haulon S, Waltham M, et al. Management of abdominal aortic aneurysms clinical practice guidelines of the European society for vascular surgery. Eur J Vasc Endovasc Surg. 2011;41 Suppl 1:S1–58.PubMed
13.
Dias NV, Riva L, Ivancev K, Resch T, Sonesson B, Malina M. Is there a benefit of frequent CT follow-up after EVAR? Eur J Vasc Endovasc Surg. 2009;37(4):425–30.PubMed
14.
Perini P, Sediri I, Midulla M, Delsart P, Gautier C, Haulon S. Contrast-enhanced ultrasound vs. CT angiography in fenestrated EVAR surveillance: a single-center comparison. J Endovasc Ther. 2012;19(5):648–55.PubMed
15.
Perini P, Sediri I, Midulla M, Delsart P, Mouton S, Gautier C, et al. Single-centre prospective comparison between contrast-enhanced ultrasound and computed tomography angiography after EVAR. Eur J Vasc Endovasc Surg. 2011;42(6):797–802.PubMed
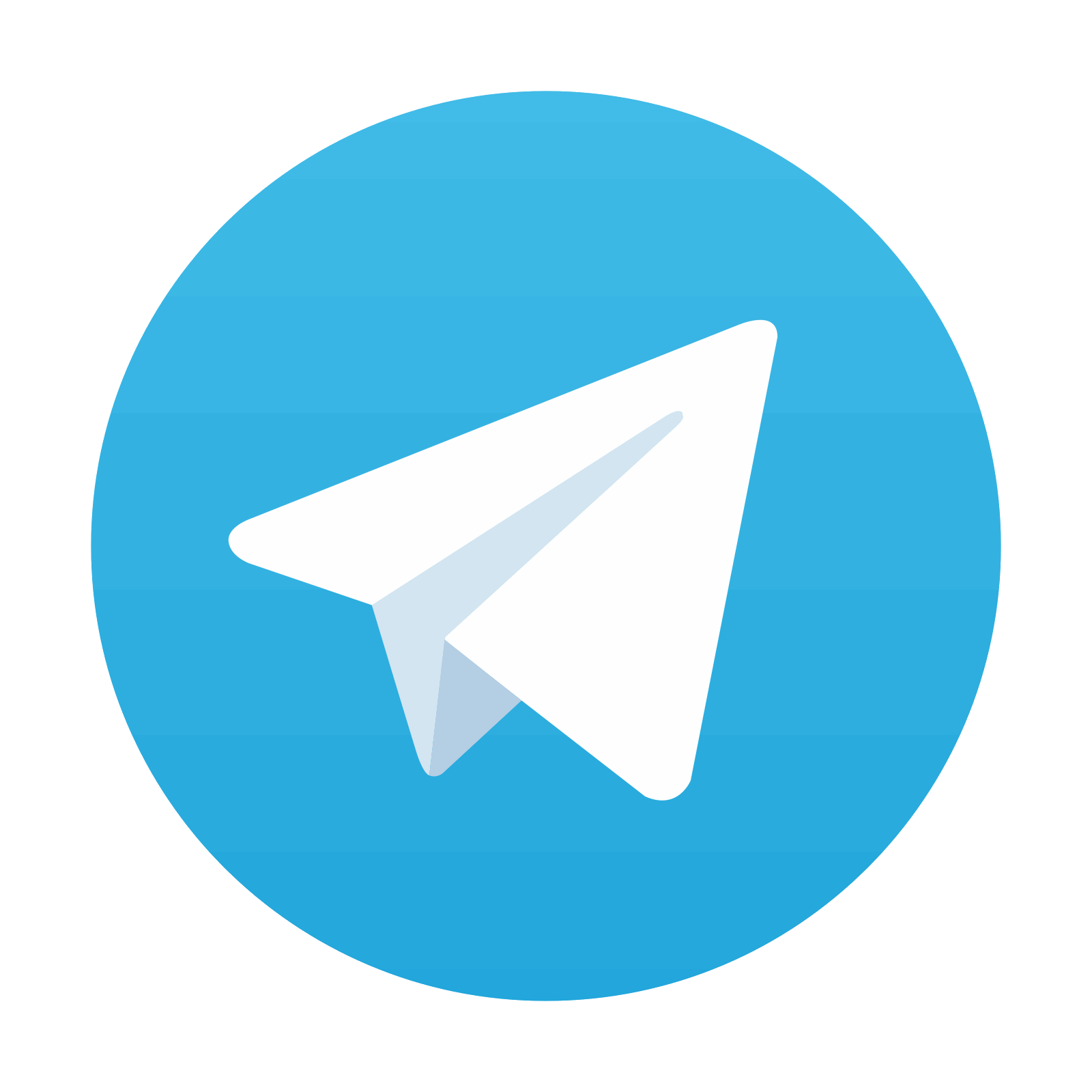
Stay updated, free articles. Join our Telegram channel

Full access? Get Clinical Tree
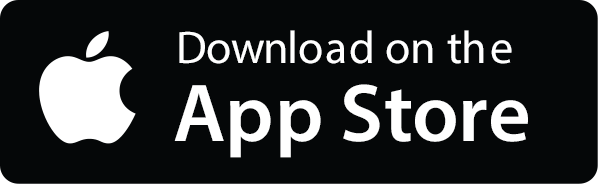
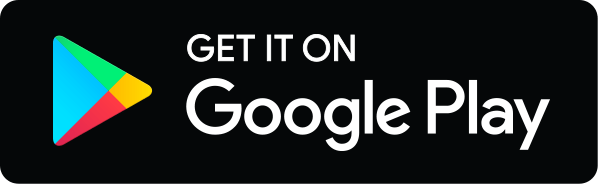