Abstract
Idiopathic ventricular tachycardia (VT) comprises 10% of all patients referred for evaluation of VT. Diagnostic features of idiopathic VT include (1) structurally normal heart and (2) QRS morphology consistent with site of origin from typical locations of idiopathic VAs (in particular, the ventricular outflow region). The echocardiogram is normal in most patients.
Idiopathic VT comprises multiple discrete subtypes that are best differentiated by their mechanism, QRS morphology, and site of origin. Most forms of focal idiopathic VTs are adenosine-sensitive and are thought to be caused by triggered activity. A prototype of an idiopathic focal VT is outflow tract VT.
Given the generally benign long-term prognosis, no pharmacological or invasive therapy is recommended for idiopathic VT in asymptomatic patients with normal left ventricular function. Pharmacological therapy can be considered in symptomatic patients. Beta-blockers, verapamil, and diltiazem are the drugs of choice, but they have limited efficacy. Catheter ablation offers cure rates of over 90% and is the treatment of choice for significantly symptomatic patients in whom drug therapy is unsuccessful, not tolerated, or not preferred. Additionally, we offer catheter ablation to patients with “malignant” forms of idiopathic VT. Catheter ablation is also recommended for patients with frequent premature ventricular complexes (PVCs) or nonsustained VT when they are presumed to be contributing to a cardiomyopathy (with suspected PVC-induced cardiomyopathy) or worsening of preexisting left ventricular dysfunction, even in otherwise asymptomatic patients.
Keywords
idiopathic ventricular tachycardia, outflow tract tachycardia, focal ventricular tachycardia, premature ventricular complexes, triggered activity
Outline
Pathophysiology, 816
Mechanism of Focal Idiopathic Ventricular Tachycardia, 818
Mechanism of Premature Ventricular Complex-Induced Cardiomyopathy, 818
Anatomical Considerations, 818
Right Ventricular Outflow Tract, 818
Pulmonary Root, 820
Left Ventricular Outflow Tract, 820
Aortic Root, 820
Aortomitral Continuity, 822
Left Ventricular Summit, 822
Left Ventricular Papillary Muscles, 822
Cardiac Crux, 823
Epidemiology and Natural History, 823
Clinical Presentation, 823
Initial Evaluation, 824
Electrocardiography, 824
Ambulatory Cardiac Monitoring, 824
Exercise Electrocardiography, 824
Cardiac Magnetic Resonance, 824
Differential Diagnosis, 825
Arrythmogenic Right Ventricular Cardiomyopathy, 825
Dilated Cardiomyopathy, 825
Idiopathic Ventricular Fibrillation, 826
Other Arrhythmia Mechanisms, 826
Principles of Management, 826
Electrocardiographic Localization of Focal Ventricular Tachycardia, 827
Electrophysiological Testing, 836
Induction of Tachycardia, 836
Tachycardia Features, 837
Diagnostic Maneuvers During Tachycardia, 837
Mapping, 837
Activation Mapping, 837
Pace Mapping, 840
Electroanatomic Mapping, 841
Noncontact Mapping, 842
Basket Catheter Mapping, 843
Ablation, 843
Target of Ablation, 843
Ablation Technique, 843
Endpoints of Ablation, 854
Outcome, 854
Cryoablation, 854
Pathophysiology
Ventricular tachycardia (VT) is usually associated with structural heart disease—most commonly coronary artery disease and cardiomyopathy. However, about 10% of patients who present with VT have no obvious structural heart disease (idiopathic VT). Absence of structural heart disease is usually suggested if the electrocardiogram (ECG) (except in Brugada syndrome and long QT syndrome), echocardiogram, and coronary arteriogram collectively are normal.
Nevertheless, cardiac magnetic resonance (CMR) may demonstrate mild structural abnormalities and subtle areas of diminished wall motion in some patients with idiopathic VT, even if all other test results are normal. In addition, focal dysautonomia in the form of localized sympathetic denervation has been reported in patients with VT and no other obvious structural heart disease. However, there is no conclusive evidence that such structural abnormalities are causally related to idiopathic ventricular arrhythmias (VAs). Of note, idiopathic VAs can also occur in patients with apparent structural heart disease, in whom the structural cardiac abnormalities are not related to the VAs. Furthermore, frequent or incessant idiopathic VAs can be a cause of cardiomyopathy.
Several distinct types of idiopathic VTs have been recognized and classified with respect to the chamber of origin of the VT (right ventricle [RV] vs. left ventricle [LV]), VT morphology (left bundle branch block [LBBB] vs. right bundle branch block [RBBB] pattern), response to exercise testing, response to pharmacological agents (adenosine-sensitive versus verapamil-sensitive vs. propranolol-sensitive VT), and behavior of VT (repetitive salvos vs. sustained).
A prototype of an idiopathic focal VAs is outflow tract (OT) VAs. Approximately 90% of OT VAs are caused by one of two phenotypic forms of adenosine-sensitive VT. Repetitive monomorphic VT is the most common form (60% to 90%) and is characterized by frequent isolated monomorphic premature ventricular complexes (PVCs), couplets, or salvos of nonsustained VT, interrupted by brief periods of normal sinus rhythm (NSR; Fig. 23.1 ). This form of VT usually occurs at rest or following a period of exercise, and typically decreases during exercise, but can be incessant. On the other hand, paroxysmal exercise-induced VT is characterized by sustained episodes of VT precipitated by exercise or emotional stress, separated by long intervals of NSR with infrequent PVCs ( Fig. 23.2 ). Evidence has suggested that both types represent polar ends of the spectrum of idiopathic VT caused by triggered activity, and there is considerable overlap between the two types. Furthermore, this subtype classification, although useful, is not necessarily precise and depends on the means and duration of rhythm recordings. Patients are typically categorized based on their presenting or index arrhythmia. Prolonged telemetry and long-term ambulatory ECG recordings have demonstrated that most patients with one subtype of OT VAs show evidence for at least one other subtype with an identical morphology. Almost all patients with nonsustained VT have high-density repetitive runs and frequent PVCs. In patients who present with repetitive PVCs, nonsustained VT can also be observed in approximately 70%; however, only 20% of these patients develop runs of more than five beats.


Mechanism of Focal Idiopathic Ventricular Tachycardia
Idiopathic VT comprises multiple discrete subtypes that are best differentiated by their mechanism, QRS morphology, and site of origin. Most forms of focal idiopathic VAs are adenosine-sensitive and are thought to be caused by catecholamine-induced, cyclic adenosine monophosphate (cAMP)–mediated delayed afterdepolarizations (DADs) and triggered activity. Several features of idiopathic VAs support triggered activity as the underlying mechanism ( see Chapter 3 ). Heart rate acceleration facilitates VT initiation. This can be achieved by catecholamine infusion or rapid pacing from either the ventricle or the atrium. In addition, termination of the VT is dependent on direct blockade of the dihydropyridine receptor by calcium channel blockers or by agents or maneuvers that lower cAMP levels (e.g., by activation of the M 2 muscarinic receptor with edrophonium or vagal maneuvers, inhibition of the beta-adrenergic receptor with beta-blockers, or activation of the A 1 adenosine receptor with adenosine). Furthermore, a direct relationship exists between the coupling interval of the initiating ventricular extrastimulus (VES) or ventricular pacing cycle length (CL) and the coupling interval of the first VT beat. In addition, VT initiation is CL-dependent; pacing cycle lengths (PCLs) longer or shorter than a critical CL window fail to induce VT. This critical window can shift with changing autonomic tone. Notably, a small proportion (11%) of VAs are insensitive to adenosine.
A subset of idiopathic focal VAs arises from the Purkinje system in either ventricle. Focal Purkinje VTs (classified as “propranolol-sensitive automatic VTs”) are typically provoked by exercise and catecholamines and are suppressed by beta-blockers (but not verapamil). Furthermore, programmed electrical stimulation fails to induce or terminate those arrhythmias. Purkinje VTs are transiently suppressed by adenosine and with overdrive pacing. These characteristics suggest abnormal automaticity as the underlying mechanism of focal Purkinje VTs, in contrast to the reentrant verapamil-sensitive fascicular VT. Purkinje VAs can manifest as monomorphic VT or PVCs, or as an accelerated idioventricular rhythm that competes with and can be suppressed by NSR.
Other subtypes of idiopathic VAs include verapamil-sensitive, reentrant fascicular VT ( see Chapter 24 ), and idiopathic polymorphic VT and ventricular fibrillation (VF; see Chapter 31 ). Those arrhythmias are discussed in elsewhere in this book. In this chapter, focal idiopathic monomorphic PVCs and VT are collectively referred to as “idiopathic ventricular arrhythmias (VAs).”
Mechanism of Premature Ventricular Complex-Induced Cardiomyopathy
Frequent idiopathic nonsustained VT or PVCs can precipitate a reversible form of dilated cardiomyopathy. The mechanisms of how frequent PVCs cause LV systolic dysfunction have yet to be elucidated. Potential mechanisms include (1) dyssynchronous ventricular contraction caused by abnormal ventricular activation during PVCs (similar to LBBB or ventricular pacing); (2) alterations in intracellular calcium handling and membrane ionic currents (caused by short PVC coupling intervals and post-extrasystolic potentiation of contractility); (3) abnormal ventricular filling (due to the postextrasystolic pause); (4) myocardial and peripheral vascular autonomic dysregulation; and (5) alterations in heart rate dynamics and hemodynamic parameters. Although PVC-induced cardiomyopathy was originally thought to be a type of tachycardia-induced cardiomyopathy, tachycardia is an unlikely mechanism, since the overall heart rates in patients with frequent PVCs have remained within the normal range due to the usual compensatory pause following the PVC. Interpolated PVCs can increase the overall heart rate.
The most prominent predictor of cardiomyopathy in patients with frequent PVCs appears to be the daily burden of PVCs. However, the PVC burden necessary to induce LV dysfunction is not yet clearly defined. Although a PVC burden as low as 4% to 10% can be associated with cardiomyopathy, a PVC burden of more than 13% to 24% appears be more likely to cause PVC-induced cardiomyopathy.
Nonetheless, it is well recognized that considerable variability exists in susceptibility to PVC-related cardiomyopathy, even among patients with high PVC burden. Multiple other factors were linked to the development of PVC-induced cardiomyopathy, including (1) lack of symptoms (with the consequent delay of diagnosis and treatment); (2) broader PVC-QRS duration (>140 or >153 milliseconds, likely due to more pronounced mechanical dyssynchrony); (3) longer PVC coupling interval (due to more pronounced LV dyssynchrony); (4) percent PVC interpolation; (5) non-OT site of origin of PVCs (in particular papillary muscle and epicardial foci); (6) presence of retrograde P waves following PVCs (likely related to worsened hemodynamics caused by simultaneous atrial and ventricular contraction); and (7) constant PVC burden throughout the day and night (i.e., less circadian fluctuation in PVC frequency). However, it is important to recognize that there is inconsistent evidence as to whether these factors represent independent predictors of PVC-induced cardiomyopathy. In fact, different studies arrived at different conclusions regarding the association of the different factors with developing LV dysfunction. Furthermore, a significant overlap in these parameters usually exists between groups with and without PVC-induced cardiomyopathy, limiting their prognostic utility.
Anatomical Considerations
Most idiopathic VAs originate from the OT region of either ventricle (most frequently from the right ventricular outflow tract [RVOT]). Other variants of OT VAs (with similar underlying electrophysiology [EP] mechanism) include VAs arising from the aortic sinuses of Valsalva, pulmonary artery, mitral or tricuspid inflow tracts, papillary muscles, and epicardial foci in close proximity to the coronary venous system (in the LV summit or cardiac crux). The thorough knowledge of the unique and complex anatomical relationships of the OTs is of utmost importance for analyzing the ECG and mapping findings during OT VAs, as well as for safe catheter maneuvering and ablation.
Right Ventricular Outflow Tract
The RVOT is the tube-like portion of the RV cavity above the supraventricular crest, and is defined superiorly by the pulmonic valve and inferiorly by the RV inflow tract and the top of the tricuspid annulus (the region of the His bundle [HB] and proximal right bundle branch). The RVOT passes cephalad in a posterior and slightly leftward direction. The lateral aspect of the RVOT region is the RV free wall. The medial aspect is formed by the anterior interventricular septum at the base of the RVOT and RV musculature opposite to the anterior left ventricular outflow tract (LVOT; as a cephalad continuation of the interventricular septum) and the root of the aorta (immediately adjacent to the right aortic sinus of Valsalva) at the region just inferior to the pulmonic valve ( eFig. 23.1 ).

Although the medial aspect of the RVOT is frequently referred to as the “septal” wall, it should be noted that the OT per se is not part of the interventricular septum, and the septum is a component only of the most proximal (inferior) part of the RVOT at the branch point of the septomarginal trabeculation. Above this area, the RVOT curves to pass anterior and cephalad to the LVOT, and, therefore, any perforation in the “septal” part is more likely to go outside the heart than into the LV ( Fig. 23.3 ).

From the coronal view above the pulmonic valve, the RVOT region is seen wrapping around the LVOT and the root of the aorta and extending leftward (see eFig. 23.1 ). Whereas the inflow portion of the RV lies to the right and anterior to the inflow portion of the LV, the RVOT courses anterior to the LVOT such that the distal RVOT and pulmonic valve are located to the left of the aortic valve and the distal LVOT. The pulmonic valve is typically placed approximately 1 to 2 cm cephalad and to the left of the aortic valve and offset 90 degrees from the aortic valve in the horizontal plane. Hence the supravalvular portion of the aorta lies in immediate proximity to the portions of the pulmonic valve. Immediately anterior to the aortic valve is the posterior muscular infundibular portion of the RVOT.
The top of the RVOT can be convex or crescent-shaped, with the posteromedial region directed rightward and the anterolateral region directed leftward. The anteromedial aspect of the RVOT actually is located in close proximity to the LV epicardium, adjacent to the anterior interventricular vein and in proximity to the left anterior descending coronary artery. The aortic sinuses of Valsalva sit squarely within the crescent-shaped posterior region of the RVOT and are inferior to the pulmonic valve (see eFig. 23.1 ). The most posterior aspect of the RVOT is adjacent to the region of the right sinus of Valsalva, and the posteromedial (leftward) surface is adjacent to the anterior margin of the right sinus of Valsalva or the medial aspect of the left sinus of Valsalva.
The thickness of the RVOT wall is variable, ranging from approximately 3 to 6 mm, and is thinnest in the rightward, anterior, and subpulmonic valve portions, and thickest in the posterior infundibular part that is adherent to the anterior LVOT as a cephalad continuation of the interventricular septum. Of note, despite the absence of epicardial connections, subendocardial fibers connect the RVOT and LVOT across the infundibular septum.
Pulmonary Root
The pulmonic and aortic valves are not at the same level (see eFig. 23.1 ). The pulmonic valve, the most superiorly situated of the cardiac valves, lies at the level corresponding to the third left costal cartilage at its junction with the sternum. The transverse plane of the aortic valve slopes inferiorly, away from the plane of the pulmonic valve, such that the orifice of the aortic valve faces rightward at an angle of at least 45 degrees from the median plane.
Because of its anterior and leftward location, only the posterior and rightward parts of the pulmonary artery have important relations with other cardiac structures. Of the three cusps of the pulmonic valve, the septal (right) pulmonic cusp lies at variable distances from and sometimes adjacent to the distal portions of the right atrial (RA) appendage. The left pulmonic cusp, being the most superficial, lies immediately beneath the pericardium and has no other cardiac structures related to it. The posterior pulmonary cusp overlies in the proximal portion of the left main coronary artery and the distal portions in the LA appendage. The supravalvular portion of the aorta lies close to and in some cases adjacent to the junction and surrounding parts of the right and posterior pulmonic cusps.
The pulmonary sinuses are not as prominent as the aortic sinuses. Nonetheless, owing to the semilunar configuration of the valvular leaflets, the hinge line of each leaflet crosses the ventriculo-arterial junction at two points. Consequently, there are always small segments of the infundibular myocardium at the nadirs of the three sinuses. Between adjacent sinuses, the wall comprises small triangles of fibrous tissue that become incorporated into the RV when the valve closes. On the epicardial aspect, the ventriculo-arterial junction is not always a sharply defined line. Sleeves of ventricular myocardium extend above the semilunar valves for a variable distance (a few millimeters and up to more than 2 cm).
The subpulmonary infundibulum is an entirely muscular funnel that supports in uniform fashion the leaflets of the pulmonic valve. As a result, all three pulmonary sinuses are continuous with myocardium of the RVOT. The muscular fibers typically extend circumferentially around the pulmonic valve between and above all three cusps just above the annulus; more distally the extension is patchy and generally asymmetrical. The presence of fibrosis and fatty tissue among these muscular sleeves may create a possible substrate for VAs. In studies using pulmonary arteriography or intracardiac echocardiography (ICE) to accurately identify the location of the pulmonic valve, up to 50% of “RVOT” arrhythmia foci were actually localized beyond the pulmonic valve.
Left Ventricular Outflow Tract
Unlike the RV, the inflow and outflow regions of the LV are at an acute angle to one another. Although commonly referred to as the LVOT, there is no tubular muscular “tract” in the LV outflow region. The LVOT consists of both muscular and fibrous portions. This contrasts with the RVOT, which is composed entirely of myocardium. The central location of the aortic valve places the LVOT between the mitral valve and the ventricular septum. The curvature of the ventricular septum forms the anterosuperior wall of the LVOT, and this continues smoothly into the LV wall. The major portion of the septal component is primarily muscular, but also includes the membranous portion of the ventricular septum. Adjoining the membranous septum is the fibrous half of the LVOT that makes up the posterior wall. This is formed by the area of fibrous continuity between the aortic valve and the anterior leaflet of the mitral valve. The mitral leaflet hangs like a curtain between the inflow and OTs of the LV. The extremities of the fibrous continuity are the left and right fibrous trigones, the right trigone forming the central fibrous body. The LVOT passes underneath the RVOT in a rightward and cephalad direction pointing toward the right shoulder (see Fig. 23.3 ).
The atrioventricular node (AVN), located in the wall of the right atrium at the apex of the triangle of Koch, is relatively distant from the aortic root. As the conduction axis penetrates through the central fibrous body, however, it is positioned at the base of the interleaflet triangle between the noncoronary and right aortic sinuses ( eFig. 23.2 ).

Aortic Root
The aortic root is the portion of the LV outlet that supports the leaflets of the aortic valve and represents the interface between the LV and the ascending aorta. The aortic root extends from the sinotubular junction in the aorta to the plane defined by the bases of the aortic valve leaflets attaching to the crown shaped aortic annulus. Within these boundaries, the aortic root is composed of the aortic valve leaflets, the sinuses of Valsalva, and the interleaflet triangles ( eFig. 23.3 ).



Sinotubular Junction
The sinotubular junction marks the junction of the sinuses and the aorta proper. On the innermost aspect of the aortic wall, the sinotubular junction forms a slightly raised ridge of thickened aortic wall (known as the sinotubular ridge or supra-aortic ridge) at the upper margin of each sinus.
Aortic Valve
The aortic valve is normally composed of three symmetrical, semilunar-shaped leaflets (cusps). Each aortic cusp has (1) a functional hinge point where it attaches to the aortic root, (2) a body of the semilunar valve, and (3) a coaptation surface of the leaflet with a thickened central nodule (nodule of Arantius). The hinge points of the aortic leaflets attach to the fibrous skeleton within the aortic root in a semilunar fashion, instead of the traditional sense of a ring-like, annular valvular attachment. Therefore the hinge line of the attachment of the aortic valvular leaflets actually forms a crown-like structure (the annulus fibrosus) with the tips right at the sinotubular junction and the nadirs within the LVOT (see eFig. 23.3 ). The apices of the leaflets attaching to the annulus fibrosus attach to the sinotubular junction. The bases of the aortic leaflets attach to the annulus at or below the anatomical ventriculo-arterial junction. The semilunar hinge lines of adjacent leaflets meet at the level of the sinotubular junction, forming the commissures.
Aortic Sinuses
Corresponding with each of the three valvular leaflets, the aortic root is expanded forming the three aortic “sinuses of Valsalva.” The aortic sinuses of Valsalva are defined inferiorly by the attachments of the aortic valve leaflets and superiorly by the sinotubular junction (see eFig. 23.3 ). Three equally spaced sites of minimal tethering within the aortic root mark the junctions of the sinuses of Valsalva. Each sinus is associated with a leaflet of the aortic valve, whereas the junctions between the adjacent sinuses are aligned with the commissures between the aortic valve leaflets. Two of the aortic sinuses give rise to the left and right coronary arteries, and these sinuses are termed right and left coronary sinuses (CS), respectively (see eFig. 23.2 ). Usually no vessels arise from the third sinus, which is then termed the noncoronary aortic sinus. In anatomical descriptions, however, the aortic sinuses (and cusps) are named anterior (for right coronary), left posterior (for left coronary), and right posterior (for noncoronary). In attitudinal orientation, however, the sinuses are in anterior, left posterolateral, and right posterolateral positions, respectively (see eFig. 23.1 ).
Interleaflet Triangles
Because of the semilunar nature of the attachments of the valvular leaflets, there are three triangular extensions of the LVOT that reach to the level of the sinotubular junction. Therefore, when considered as a whole, the aortic root is divided by the semilunar attachment of the aortic leaflets (i.e., leaflet annulus) into supravalvular and subvalvular components. The supravalvular components, the aortic sinuses, are primarily aortic in structure, but contain structures of ventricular origin at their base. The supporting subvalvular parts (the interleaflet triangles) are primarily ventricular ( eFig. 23.4 ). However, these triangles are formed not of ventricular myocardium but of the thinned fibrous walls of the aorta between the expanded sinuses of Valsalva.

Ventriculo-Aortic Junction
Unlike the tricuspid and pulmonic valves that occupy opposite ends of the banana-shaped RV, the aortic and mitral valvular orifices are fitted alongside each other (with no muscle in between) within the elliptical ostium of the LV. As a result, the leaflets of the aortic valve are attached only in part to the muscular walls of the LV. About half of the circumference of the lower part of the aortic root is connected to the muscular ventricular septum, with the remaining half in fibrous continuity with the anterior leaflet of the mitral valve.
The “anatomical” ventriculo-aortic junction is a circular locus within the aortic root, marking the interface between the ventricular structures and the fibro-elastic walls of the aortic sinuses. This discrete ring, however, is markedly discordant with the morphology of the semilunar attachment of the leaflets of the aortic valve (the annulus fibrosus) that form the “hemodynamic” junction between the LV and the aorta. Indeed, ventriculo-aortic junction is crossed at several points by the hinge lines of the valvular leaflets. As a result, a crescent of ventricular musculature is incorporated at the base of each of the right and left aortic sinuses. In contrast, there is no muscular crescent at the base of the noncoronary sinus, since this sinus has exclusively fibrous walls, the basal part beneath the anatomical ventriculo-aortic junction being part of the aortomitral continuity (see eFig. 23.4 ).
Furthermore, sleeves (isolated strands) of ventricular myocardium extend beyond the aortic valve attachments for variable distances (analogous to atrial myocardial extensions in the pulmonary veins [PVs]). Myocardial extensions can be seen in the aortic wall, in the valve leaflet itself, or in the intercuspal region. The myocardial extensions within the aortic sinuses are mostly asymmetrical and do not exceed more than a few millimeters above the basal valvar attachment. Whereas the right sinus of Valsalva and the anterior portions of the left sinus of Valsalva frequently exhibit these myocardial sleeves (given their anatomical contact with ventricular myocardium), the posterior portions of the left sinus and the entire noncoronary sinus of Valsalva, particularly in relation to the aortomitral continuity, are exclusively fibrous and do not come in direct contact with the LV myocardium. The noncoronary cusp at its junction with the right sinus of Valsalva may have sleeves of ventricular myocardium, and at present, it is not clearly known whether myocardial extensions into the noncoronary sinus represent atrial or ventricular myocardium.
Approximately 10% to 15% of idiopathic VAs require ablation within the aortic root. The left sinus of Valsalva is the most common source, followed by the right sinus, and then the left-right sinus junction. The substrate of this VT likely originates from the strands of ventricular myocardium present at the bases of those sinuses. In contrast, the base of the noncoronary sinus of Valsalva is composed of fibrous tissue and, thus, is an extremely rare site of origin of VT (7% of the idiopathic aortic root VAs). However, the nature of the actual substrate being ablated has not been clearly defined. For example, the ablation electrode placed in the depth of the right sinus of Valsalva may be mapping and ablating a focus arising from the supravalvular extension into the cusp, LVOT myocardium, or a deeper posterior RVOT myocardium.
Anatomical Relationships
The aortic valve is the cardiac centerpiece; it lies in contact or continuity with all four cardiac chambers and shares important proximate relationships with each of the other cardiac valves ( see Fig. 9.1 ). It comes into contact with the RA, LA, interatrial septum, RVOT, mitral valve (aortomitral continuity), pulmonic valve, tricuspid valve, and conduction system (see eFig. 23.1 ). The aortic valve plane tilts rightward (at about 30 to 45 degrees) in the horizontal plane as it is joined to the LV ostium. This positions the noncoronary sinus of Valsalva as the most inferior of the aortic sinuses, the left sinus of Valsalva as the most superior, and the right sinus of Valsalva as the most anterior. The degree of tilt is determined by the axis of the LV and can vary substantially among different individuals. As the LV axis flattens horizontally, the right and noncoronary sinuses are displaced inferiorly relative to the left sinus. The aortic root is inferior, posterior, and somewhat rightward compared with the RVOT. The posterior subpulmonic valve portions of the RVOT are immediately anterior and more proximally continuous with the anterior myocardial subaortic LVOT and more distally to the right sinus ( eFig. 23.5 ). The lateral and more distal portions of the left sinus of Valsalva lie immediately subjacent to the peripulmonic valve portions of the RVOT.

The triangle between the noncoronary and left sinuses is part of the aortomitral fibrous continuity. The triangle between the noncoronary and the right sinuses is directly continuous with the membranous part of the ventricular septum. The triangle between the left and right sinuses is the least extensive of the three, and abuts the muscular sub-pulmonary infundibulum ( see Fig. 9.1 ).
Left Aortic Sinus of Valsalva
The left sinus of Valsalva lies to the left of the right sinus and is related to the posterior wall of the RVOT ( see Fig. 9.1 ). The anterior part of the left sinus and the commissure between the right and left sinuses lie just posterior to the distal RVOT and are close but caudal to the posterior pulmonic valve annulus. The rest of the left sinus (more leftward and posteriorly) lies in fibrous continuity with the anterior leaflet of the mitral valve (namely, the aortomitral continuity). Adjacent to this site lies the peripulmonic valve myocardium and, more laterally, the posterior lobe of the LA appendage (when present). Rightward tilting of the aortic root causes the left sinus to be situated higher than the right and noncoronary sinuses. The right sinus of Valsalva and anterior part of the left sinus are connected with the ventricular musculature at their bases because the semilunar leaflets are hinged superiorly to the aortic wall but inferiorly to muscle.
Right Aortic Sinus of Valsalva
The right aortic sinus is the most anterior sinus relative to the sternum, and it lies immediately posterior to the relatively thick posterior infundibular portion of the RVOT ( see Fig. 9.1 ). Caudally, there is continuity with the anterior LVOT, and at the level of valve insertion there is either physical continuity or very close proximity between the myocardium that extends above this sinus, the LVOT, and the posterior RVOT (see eFig. 23.1 ). The posterior part of the right sinus of Valsalva is adjacent to the central fibrous body, which carries within it the penetrating portion of the HB. Anteriorly, the right sinus is related to the bifurcating AV bundle and the origin of the left bundle branch (see eFig. 23.2 ). The right sinus does not have a direct relationship with either atrium, but lateral to the commissure with the noncoronary sinus lies the RA appendage, trunk of the right coronary artery, and a variable amount of fat ( eFig. 23.6 ).

Noncoronary Aortic Sinus of Valsalva
The noncoronary sinus of Valsalva is the most posterior, inferior, and medial of the aortic cusps. The noncoronary sinus lies immediately anterior to the interatrial septum and superior to the central fibrous body and has the RA and LA as the posterior right and left relations, respectively (see eFigs. 23.2 and 23.6 ). Because of this relationship to the atria, some atrial tachycardias (ATs) arising from the roof of the interatrial septum can be ablated only within the noncoronary sinus. For most of its anatomical course, the noncoronary sinus is attached to the anterior leaflet of the mitral valve from its most leftward extent in continuity with the left sinus commissure. Only the most anterior and rightward extension of the noncoronary sinus comes into contact with the interventricular septum near its junction with the right sinus of Valsalva. The commissure between the noncoronary and the right sinuses is located immediately adjacent to the commissure of the anterior and septal leaflets of the tricuspid valve ( see Fig. 9.1 ). The joining of these commissures forms the membranous portion of the interventricular septum and is the location of the penetrating HB. The compact AVN itself is located more posteriorly and inferiorly to this commissure. The membranous septum is crossed on its right side by the hinge of the tricuspid valve, which divides the septum into atrioventricular and interventricular components. The membranous septum and anterior mitral leaflet almost completely separate the noncoronary sinus from the LV myocardium. Because of this limited relationship to the ventricle, VAs originating from the noncoronary sinus are very rare (7% of the idiopathic aortic root VAs).
Aortomitral Continuity
The cardiac skeleton consists of four rings of dense connective tissue that surround the AV canals (mitral and tricuspid) and extends to the origins of the aorta and the pulmonary trunk. The aortic valve occupies the central position with the other valve rings attached to it or nearby (pulmonic). The right fibrous trigone is formed by the triangular formation between the aortic valve and the medial parts of the tricuspid and mitral valves, and it represents the largest thickening and strongest portion of the cardiac skeleton. Together with the membranous septum, the right fibrous trigone constitutes the central fibrous body ( see Fig. 9.1 ).
Another junction between the mitral annulus and aortic valvular ring occurs at the left fibrous trigone, anchoring the anteromedial aspect of the mitral annuls to the base of the left aortic sinus of Valsalva. The left fibrous trigone is less substantial than the right fibrous trigone. Between the left and right fibrous trigones, a rigid and broad fibrous curtain (often referred to as the aortic curtain) extends across the anterior leaflet of the mitral valve and supports the aortic valve leaflets. The aortomitral continuity refers to a fibrous continuity of the medial part of the noncoronary and left sinuses and the anterior mitral leaflet.
The membranous interventricular septum is an inferior extension of the central fibrous body that attaches to the muscular interventricular septum. The membranous septum is crossed on its right aspect by the attachment of the tricuspid valve, dividing the septum into atrioventricular and interventricular components. As a result, the membranous septum separates the LVOT from both the RV and RA; the line of attachment of the tricuspid annulus determines the line of division between the RV and RA. The penetrating HB runs through the portion of the membranous interventricular septum at the commissure where the right sinus of Valsalva meets the noncoronary sinus ( see Fig. 9.1 ).
Although the aortomitral continuity is a fibrous structure, catheter ablation in this region can successfully eradicate VAs arising in this vicinity. The exact origin of arrhythmias ablated from this fibrous region is still uncertain.
Left Ventricular Summit
The LV summit refers to the most superior portion of the LV epicardium, which lies on the basal portion of the LV ostium next to the septum and the anterior mitral annulus, abutting on the left aortic sinus of Valsalva superiorly, laterally, and anteriorly.
The LV summit represents the triangular region in the epicardial LVOT with its apex at the bifurcation of the left main coronary artery (between the left anterior descending and the circumflex coronary arteries) and its base formed by an arc connecting the first septal perforator branch of the left anterior descending coronary artery and the circumflex coronary artery ( eFig. 23.7 ). The left main coronary artery arises cranial to the left sinus of Valsalva (about 15 to 20 mm above the nadir of the cusp). The aortomitral continuity and LV summit face each other, with the superior end of the LV muscle between them.

The great cardiac vein bisects the LV summit into superior (closer to the apex of the summit) and inferior regions. Approximately 12% of VAs from the LV are estimated to occur from the LV summit. While the inferior portion of the summit is usually accessible to epicardial catheter ablation, successful ablation in the superior region is frequently limited by the close proximity of the coronary arteries and the thick layer of epicardial fat that overlies the proximal portion of these vessels.
Left Ventricular Papillary Muscles
The anterolateral (or anterior) and posteromedial (or posterior) LV papillary muscles are thick, finger-like processes attached to the ventricular wall at one end and to the mitral leaflets by multiple tendons (chordae tendineae) on the other end ( Fig. 23.4 ). The anterior papillary muscle originates from the middle to apical region of the LV ventral wall, lies on the anterolateral free wall, and provides chordae to the anterolateral half of the anterior and posterior mitral leaflets. The posterior papillary muscle arises from the inferoseptal wall of the LV, lies between the LV septum and posterior free wall, and provides chordae to the posteromedial half of both leaflets. The anterior papillary muscle typically has a single head, while the posterior papillary muscle often has two heads. Focal VAs generally originate from the base of the papillary muscle.

The peripheral Purkinje network extends to the surface of the papillary muscles. Free-running false tendons traverse the ventricular chamber and reattach at the free wall myocardium, projecting predominantly toward the papillary muscles. The left anterior fascicle crosses the anterobasal LV region toward the anterior papillary muscle and terminates in the Purkinje system of the anterolateral LV wall. The left posterior fascicle fans out extensively toward the posterior papillary muscle and terminates in the Purkinje system of the posteroinferior LV wall. The close relationship that exists between the Purkinje fiber and papillary muscles has caused some difficulties in distinguishing arrhythmias from the two structures. Furthermore, VAs originating from the body of the papillary muscle can exhibit multiple QRS morphologies caused by variable exit sites from an intramural focus or its attachment to false chordae.
Cardiac Crux
The cardiac crux (crux cordis, from Latin “crux” meaning “cross”) is the posteroseptal region (the diaphragmatic aspect) of the heart where the atrioventricular, interventricular, and interatrial grooves form a roughly cross-shaped intersection. This four-sided pyramidal space resents the confluence of all four cardiac chambers and the CS in their nearest proximity. The “basal” crux area lies in proximity to the ostium of middle cardiac vein, whereas the “apical” crux area lies near the posterior interventricular artery, more inferior and epicardial as compared with the basal crux area. Hence the apical crux is often not accessible from the proximal CS or the middle cardiac vein, and a transcutaneous subxiphoid epicardial approach is typically required.
Epidemiology and Natural History
Idiopathic VT comprises 10% of all patients referred for evaluation of VT. Idiopathic VAs are not evenly distributed, but have a predilection for originating from certain anatomical regions of the ventricles and aortic and pulmonary roots. Approximately 60% to 80% of idiopathic VAs arise from the ventricular outflow regions, including myocardium around the aortic and pulmonic cusps, as well as the summit of the LV. Although the RVOT has been the most common site of origin of OT VAs, a significant proportion (20% to 50%) arise from the LVOT and adjacent structures.
Age at presentation is usually 30 to 50 years (range, 6 to 80 years). Women are more commonly affected, although LVOT VAs are more frequently observed in men. The clinical course is benign and the prognosis is excellent. Sudden cardiac death is rare. Spontaneous remission of the VT occurs in 5% to 20%. However, very frequent idiopathic nonsustained VT or PVCs can precipitate a potentially reversible form of LV systolic dysfunction (observed in about 5% to 7% of patients with a PVC burden >10%). The relationship between the LV dysfunction and VT may not be initially recognized, and those patients can present with heart failure symptoms and become diagnosed with idiopathic dilated cardiomyopathy, and may even undergo prophylactic implantation of a defibrillator, which unfortunately often results in the delivery of inappropriate shocks triggered by frequent nonsustained episodes of idiopathic VT. Rarely, monomorphic focal PVCs can trigger idiopathic polymorphic VT or VF in patients with no apparent structural heart disease.
Clinical Presentation
When present, symptoms are mild in many of patients, but they can be disabling in some individuals. Most symptomatic patients present with palpitations, 50% develop lightheadedness, and a minority (<10%) present with syncope. Symptoms can be attributed to the PVC, the subsequent compensatory pause, the hypercontractile sinus beat following the pause, or a combination of all. A sensation that the heart has “stopped” or pulsation in the head or neck can be noted.
Not infrequently, initial presentation can be related to effort intolerance due to fatigue and dyspnea, as a result of decreased effective cardiac function. Some patients can be completely asymptomatic and present with ectopy detected incidentally by an irregular pulse or on cardiac recordings obtained for other clinical reasons. Other patients present because of “low pulse rates,” which is related to ineffective ventricular contraction during PVCs with insufficient pressure to allow opening of the aortic valve.
Most commonly, patients present with frequent PVCs or short runs of repetitive nonsustained VT. Less frequently, paroxysmal sustained VT is observed. Idiopathic VAs are usually provoked by emotional or physical stress and stimulants such as caffeine. Not infrequently, however, VAs become quiescent during exercise and arise only during the recovery phase or during rest. In females, the burden of arrhythmia tends to increase during periods of hormonal variations (premenstrual and perimenopausal).
Frequent idiopathic nonsustained VT or PVCs can precipitate a reversible form of LV systolic dysfunction, and present with heart failure symptoms. Such arrhythmias may also exacerbate preexisting LV dysfunction and is a cause of loss of effective biventricular pacing in heart failure patients treated with cardiac resynchronization therapy. At the first clinical encounter, patients often present with both PVCs and LV dysfunction. In this setting, it can be difficult to determine the onset of frequent PVCs relative to the onset of LV dysfunction and whether the cardiomyopathy is PVC-induced or PVC-worsened.
Initial Evaluation
The diagnosis of idiopathic VT is one of exclusion; structural heart disease, cardiomyopathy, and coronary artery disease should be excluded, usually by cardiac stress testing and echocardiography. Coronary angiography may be warranted, especially in those with LV systolic dysfunction and coronary risk factors.
Diagnostic features of idiopathic VAs include (1) structurally normal heart; and (2) QRS morphology consistent with site of origin from typical locations of idiopathic VAs (in particular, the RVOT or LVOT). The echocardiogram is normal in most patients. Slight RV enlargement is observed rarely.
Electrocardiography
The surface ECG during NSR is usually normal. Up to 10% of patients have complete or incomplete RBBB. Other ECG abnormalities may be observed when idiopathic VAs coexist with structural heart disease.
Idiopathic focal VAs are characterized by frequent monomorphic PVCs, couplets, or salvos of nonsustained VT, interrupted by brief periods of NSR (see Fig. 23.1 ). Paroxysmal exercise-induced VT is characterized by sustained episodes of VT precipitated by exercise or emotional stress (see Fig. 23.2 ). The tachycardia rate is frequently rapid (CL <300 milliseconds), but can be highly variable. A single morphology for the VT or PVCs is characteristic. QRS morphology during VAs on the surface ECG is discussed later in this chapter.
Ambulatory Cardiac Monitoring
Holter monitors can assess the burden of VAs and their correlation to symptoms. Cardiac event monitors are more appropriate when symptoms occur less frequently.
Several characteristics of idiopathic VAs can be observed on ambulatory cardiac monitoring. Idiopathic VAs typically occur at a critical range of heart rates (CL dependence). The coupling interval of the first PVC is relatively long (approximately 60% of the baseline sinus CL). A positive correlation exists between the sinus rate preceding the VT and the VT duration. In addition, the VT occurs in clusters, and is most prevalent on waking and during the morning and later afternoon hours. Catecholamines increase during waking hours and nocturnal decrease in catecholamine levels, and predominance of vagal tone might account for the differences in the circadian PVC burden. The largest change in PVC burden is observed in the transition from the sleeping hours to the morning waking hours. Notably, the lack of circadian fluctuation in PVCs frequency has been correlated with increased risk of PVC-induced cardiomyopathy.
Idiopathic focal VAs are very sensitive to autonomic influences, resulting in poor day-to-day reproducibility. Therefore a single 24-hour recording may not reflect the true PVC burden. When frequent PVCs are strongly suspected, it may be necessary to obtain extended Holter recordings of 48 to 72 hours or several 24-hour Holter recordings.
Exercise Electrocardiography
Exercise stress testing is recommended for evaluation of patients with exercise-related symptoms, but may not be clinically helpful in those in whom the arrhythmias have already been documented on ambulatory recordings.
Exercise testing can reproduce patients’ clinical VT 25% to 50% of the time. Exercise-provoked VT usually manifests as nonsustained or, less often, sustained VT. In those with isolated PVCs, the frequency of PVCs tends to increase during exercise. The VT can be initiated either during the exercise test or during the recovery period. Both scenarios likely represent examples of the dependence of VT on a critical window of heart rates for induction. This window can be narrow and only transiently present during exercise, resulting in induction of VT only during recovery. In some patients with repetitive monomorphic VT, the VT can become suppressed during exercise. The response of VAs to exercise can be helpful in planning the strategy for arrhythmia induction during an ablation procedure.
Catecholaminergic polymorphic ventricular tachycardia (CPVT), also exercise dependent, can be distinguished by the alternating QRS axis with 180-degree rotation on a beat-to-beat basis (bidirectional VT) or polymorphic VT, which can degenerate into VF.
Cardiac Magnetic Resonance
CMR is of value when structural heart disease is suspected despite a normal echocardiogram. CMR can help exclude arrhythmogenic right ventricular cardiomyopathy (ARVC), amyloidosis, and sarcoidosis, among other diseases. CMR can also evaluate the presence and extent of myocardial fibrosis in patients with LV dysfunction.
It is important to recognize that CMR can reveal concealed myocardial structural abnormalities involving the RV and the LV among patients with apparently idiopathic VAs, including subepicardial or midmyocardial foci of fibrosis, acute inflammation, and focal fatty infiltration. Of note, the morphology of VAs appears to be related to the presence of such abnormalities on CMR. In contrast to the infrequent abnormalities noted among patients with VAs of LBBB pattern, CMR detected myocardial structural abnormalities (mainly involving the LV inferior and lateral wall) in a sizable proportion (41%) of patients with unexplained VAs with RBBB configurations (consistent with an LV origin). Whether these abnormalities are causally related to the observed VAs is uncertain; however, the presence of myocardial structural abnormalities on CMR seems to predict spontaneous sustained arrhythmias among patients presenting with apparently idiopathic VAs of LV origin.
Differential Diagnosis
It is important to differentiate idiopathic VT from other potentially malignant forms of VT that may also arise from the OT region, including VT in ARVC, CPVT, Brugada syndrome, and idiopathic polymorphic VT and VF. A Brugada ECG pattern, findings of reduced LV or RV function, polymorphic VT, multiple monomorphic VT morphologies, a history of recurrent syncope, or a family history of sudden cardiac death mandate further detailed evaluation.
Arrythmogenic Right Ventricular Cardiomyopathy
RVOT VAs should, in particular, be distinguished from ARVC, a disorder with a more serious clinical outcome ( see Chapter 29 ). Although RVOT VAs are associated with a benign prognosis with no familial basis, it can be extremely difficult to distinguish from the concealed phase of ARVC, in which typical ECG and imaging abnormalities are absent. The VT in ARVC also affects young adults, is commonly catecholamine-facilitated, and can originate from the RVOT. The distinction between the two entities has important prognostic and therapeutic implications.
Electrocardiogram During Sinus Rhythm
Although the VT in ARVC can have morphological features similar to RVOT VAs (LBBB with inferior axis), several ECG criteria during VT can help distinguish between the two arrhythmias. Longer QRS duration in lead I (≥120 milliseconds), late precordial transition (at lead V 5 or V 6 ), and the presence of notching in the QRS in multiple leads all favor ARVC over idiopathic RVOT VAs. In addition, the presence of an LBBB morphology with a superior axis is very unlikely in idiopathic RVOT VAs. Also, VAs in ARVC patients generally have lower QRS amplitude and more fragmentation, but these are qualitative differences and not always present.
In ARVC, the resting 12-lead ECG in NSR typically shows inverted T waves in the right precordial leads. When present, RV conduction delay with an epsilon wave (best seen in leads V 1 and V 2 ) is helpful in the diagnosis of ARVC. The resting surface ECG in NSR is typically normal in patients with idiopathic VT, with no epsilon wave, QRS widening or fragmentation, or other markers of delayed activation of the RV. Patients with idiopathic VT also have a normal signal-averaged ECG in NSR.
Nevertheless, the ECG can also be normal in up to 40% to 50% of patients with ARVC at presentation, but in almost no patient 6 years after initial diagnosis. Furthermore, approximately 10% of patients with idiopathic VT can have complete or incomplete RBBB during NSR.
Electrocardiogram During Ventricular Tachycardia
In general, patients with idiopathic VT typically present with a single-morphology VT. The presence of multiple VT morphologies and RV sites of origin of the VT that are inconsistent with idiopathic RVOT VT (e.g., LBBB with superior axis) should prompt the consideration of ARVC. Nonetheless, it should be recognized that patients with several idiopathic VT morphologies have been reported, and patients with ARVC can initially present with only one VT morphology consistent with an RVOT origin.
During VTs with LBBB and inferior axis, certain features of the QRS complex favor ARVC over RVOT VT, including (1) late precordial transition (at V 5 or later); (2) QRS notching in at least two leads (in particular, leads I and aVL); and (3) QRS duration in lead I longer than 120 to 125 milliseconds. The most specific finding was late precordial transition (100% specificity at lead V 6 and 90% specificity at lead V 5 or later), and the most sensitive was duration of QRS in lead I exceeding 120 milliseconds, followed by the presence of any notching on the QRS complex. QRS notching and the longer QRS duration in ARVC reflect the abnormal myocardial substrate underlying the VT, which is lacking in idiopathic RVOT VT.
Invasive Electrophysiology Testing
An invasive EP study is usually not necessary to establish a diagnosis, although it can help exclude other forms of tachyarrhythmias in selected patients. The response of VT during EP testing can be helpful in distinguishing idiopathic RVOT VAs from ARVC. Reentry is the mechanism of VT in most ARVC patients, whereas RVOT VT almost always displays features of triggered activity. The repetitive initiation of these VAs by programmed ventricular stimulation suggests a reentrant mechanism, and is much more common in ARVC than idiopathic OT VAs (93% vs. 3%). In addition, recording fractionated diastolic electrograms during VT or NSR at the site of origin of VT or other RV sites is very rare with idiopathic RVOT VAs, but is typical for ARVC. As noted, the induction of VAs with different QRS morphologies is common in ARVC (observed in 73% of patients in one report) and is rare with RVOT VT. Furthermore, the VT in ARVC does not terminate with adenosine.
In addition, electroanatomic voltage mapping can help distinguish early or concealed ARVC from idiopathic VT by detecting RV electroanatomical scars that correlate with the histopathological features pathognomonic of ARVC.
Isoproterenol Testing
An arrhythmogenic response to high-dose intravenous (IV) isoproterenol (45 µg/min for 3 minutes, regardless of heart rate) can potentially help diagnose ARVC, particularly in the early stages of the disease. The occurrence of polymorphic VAs (PVCs and sustained and nonsustained VT) with predominant LBBB morphology (with features that are not typical for an RVOT origin) during isoproterenol testing (during isoproterenol infusion or within 10 minutes after the cessation of the infusion) is highly suggestive of ARVC (sensitivity, 91%; negative predictive value, 99%). In contrast, a sustained monomorphic RVOT VT response during isoproterenol testing is considered a negative response.
Cardiac Imaging
Patients with idiopathic VT have normal imaging studies (echocardiography, CMR, contrast ventriculography) of RV size and function. The presence of RV dilation or aneurysm is consistent with ARVC.
Dilated Cardiomyopathy
It is important to recognize that, in some patients, idiopathic VAs can coexist with structural heart disease, including cardiomyopathy, in which setting the VAs may not be related to the cardiac disease. On the other hand, very frequent idiopathic nonsustained VT or PVCs can precipitate dilated cardiomyopathy that can improve or completely resolve after elimination of the VAs. Therefore in patients who present with a dilated cardiomyopathy of unclear etiology and who have frequent PVCs or nonsustained VT, it is important to assess the contribution of VAs to LV systolic dysfunction. Failure to recognize a causal relationship between the arrhythmia and LV dysfunction can have important consequences. These patients can be misdiagnosed with “idiopathic” dilated cardiomyopathy and may not be offered therapeutic measures to reduce the PVC burden (e.g., catheter ablation), which can significantly impact LV systolic function and long-term outcome.
A reversible form of dilated cardiomyopathy precipitated by idiopathic VAs should be suspected when frequent PVCs or nonsustained VT are observed on a 24-hour Holter recording, especially when the QRS morphology is monomorphic and is suggestive of origin from typical locations of idiopathic VAs.
The diagnosis of PVC-induced cardiomyopathy is one of exclusion and often retrospective based on recovery of LV function after elimination of the arrhythmia, typically by catheter ablation. Although pharmacological suppression of the PVCs, such as by amiodarone therapy, may help evaluate the relationship between the arrhythmia and cardiomyopathy, the usefulness of this approach and duration of therapy required have not been defined.
Predictors of reversibility of LV systolic dysfunction in patients with PVC-induced cardiomyopathy include the absence of myocardial scar (on CMR), a large PVC burden baseline, and effective long-lasting elimination of PVCs. Although no single cutoff value of PVC burden completely discriminates reversible from irreversible LV dysfunction, a burden of ≥13% at baseline was shown to predict recovery of left ventricular ejection fraction (LVEF) following ablation of PVCs. Of note, early improvement in LVEF (at 1-week follow-up) post PVC ablation was shown to predict complete recovery of LV systolic function. One report suggested that patients with PVC QRS duration of 170 milliseconds or longer are unlikely to normalize their LV function after ablation of the PVCs, suggesting that PVC QRS duration can be a marker of the presence and severity of underlying structural heart disease.
Given the large magnitude of potential improvement in LV systolic function that could be achieved by elimination of frequent PVCs (regardless of whether the cardiomyopathy is in fact PVC-induced or PVC-worsened), evaluation for the presence of frequent PVCs (by 24- or 48-hour Holter monitoring) needs to be considered in all patients with depressed LVEF. This is particularly important in patients who meet criteria for primary prevention implantable cardioverter-defibrillator (ICD) implantation, as the recovery of LVEF following ablation can potentially remove the ICD indication.
Idiopathic Ventricular Fibrillation
Idiopathic monomorphic focal VAs, although generally considered “benign,” can also trigger polymorphic VT and VF in some patients with idiopathic VF and no apparent structural heart disease ( see Fig. 31.20 ). Hence, for patients presenting with idiopathic VF, it is imperative to pay special attention to recording potential monomorphic PVC “triggers,” since catheter ablation of those foci can potentially prevent further episodes of VF or reduce the burden of arrhythmias. Also, patients with polymorphic VT and VF should be carefully evaluated for underlying channelopathies.
Even in patients with no prior history of VF, it is also particularly important to distinguish the “malignant” from “benign” forms of idiopathic monomorphic VAs because the malignant form often leads to unexpected sudden cardiac death. Identification of patients presenting with idiopathic focal VAs carrying some risk for VF remains challenging. Malignant VAs should be suspected in patients with known idiopathic focal VAs who present with syncope. Patients with idiopathic VF frequently (57%) experience syncopal episodes before presenting with cardiac arrest.
Several ECG parameters have been suggested as markers of malignant OT VAs. However, the diagnostic value of these ECG parameters is inconsistent and their clinical utility is limited. Although idiopathic VF and polymorphic VT can arise from triggers located in the RVOT and LVOT, PVCs arising from the Purkinje network, RV moderator band, papillary muscles, and apical cardiac crux, due to unclear mechanism, appear to be particularly prone to induce sustained VT and VF, leading to syncope or cardiac arrest. Therefore VAs with QRS morphology suggestive of those sites of origin should be carefully scrutinized.
The prognostic implications of the coupling intervals of clinical PVCs have been debated. Although no absolute coupling interval cutoff identifies potentially “malignant” PVCs, shorter coupling interval to the preceding QRS complex, and a shorter CL during monomorphic VT were found to predict the coexistence of VF or polymorphic VT in patients with idiopathic RVOT VT/PVCs; however, significant overlap exists. In contrast, one report showed that the prematurity index (defined as the ratio of the coupling interval of the first VT beat or isolated PVC to the preceding R-R interval of the sinus cycle just before the VT or isolated PVC), but not the coupling interval, was the only independent predictor for polymorphic VT. A more recent report found that the second coupling interval of nonsustained VT was significantly shorter in malignant OT VT than in benign OT VT. A second coupling interval of nonsustained VT of less than 317 milliseconds could predict a malignant OT VT with modest diagnostic accuracy (sensitivity 58%, specificity 87.5%). However, prospective validation of these criteria is yet to be tested in a larger population.
Other Arrhythmia Mechanisms
Idiopathic OT VT should be differentiated from other forms of VT with an LBBB pattern, including bundle branch reentrant VT ( see Chapter 26 ), reentrant VT following surgical repair of congenital heart disease ( see Chapter 30 ), and postinfarction VT originating from the LV septum ( see Chapter 22 ). In addition, antidromic AVRT using an atriofascicular BT also presents with wide complex tachycardia with an LBBB pattern ( see Chapter 18 ). Often the diagnosis of these VTs can be established without difficulty, given the frequently associated structural heart disease. Nevertheless, idiopathic focal VT also can occur in patients with unrelated structural heart disease, and the distinction of those “ablatable” VTs has important prognostic and therapeutic implications.
Principles of Management
Acute Management
Acute termination of most forms of idiopathic VT can be achieved by vagal maneuvers or IV administration of adenosine. IV verapamil is an alternative, provided the patient has adequate blood pressure and has a previously established diagnosis of a VT that is sensitive to verapamil. Hemodynamic instability warrants emergency cardioversion.
Chronic Management
Conservative Management
Given the generally benign long-term prognosis, reassurance and counseling, with no pharmacological therapy, are the preferred treatment for patients with idiopathic VAs who are asymptomatic or only mildly symptomatic and have normal LV function. Annual follow-up with ambulatory ECG monitoring and echocardiography is recommended in those with frequent PVCs (>10,000 PVCs per 24 hours) to monitor for possible development of cardiomyopathy, which should prompt the initiation of therapy.
Importantly, many patients with frequent PVCs do not complain of palpitations and present primarily with occult cardiac symptoms, such as weakness, fatigue, and effort intolerance. Such complaints should not be dismissed as “unrelated,” and those patients should not be labeled “asymptomatic.” Treatment to reduce the burden of PVCs should be considered to alleviate symptoms.
Although frequent PVCs can precipitate LV systolic dysfunction in some patients who are otherwise asymptomatic, there is currently no risk stratification model to reliably identify patients at risk. Therefore prophylactic PVC elimination for the sole purpose of preventing PVC-induced cardiomyopathy is not recommended.
Pharmacological Therapy
Medical therapy can be considered in symptomatic patients. Beta-blockers, verapamil, and diltiazem are the first-line treatment. However, these medications have a modest efficacy rate (only 10% to 15% of patients achieve ≥90% PVC suppression), and they may not be well tolerated by the generally young patient population. Although class I and III antiarrhythmic drugs (sotalol, flecainide, mexiletine, propafenone, amiodarone) are more effective in reducing the burden of PVCs, they are not optimal as first-line therapy, given the potential for proarrhythmic risk and a greater side-effect profile.
Catheter Ablation
Catheter ablation is the treatment of choice for significantly symptomatic patients in whom long-term drug therapy is unsuccessful, not tolerated, or not desired. In addition, catheter ablation should be strongly considered in patients with “malignant” forms of idiopathic VAs, such as those with short-coupled PVCs and syncope or cardiac arrest, or in whom the PVCs were found to trigger polymorphic VT or VF.
Catheter ablation is also recommended for patients with frequent PVCs or nonsustained VT when they are presumed to be contributing to a cardiomyopathy or worsening of preexisting LV dysfunction, even in otherwise asymptomatic patients. Effective elimination of PVCs (reduction of PVC burden is by >80% or to <5000 PVCs per 24 hours) with ablation has been shown to improve LVEF, ventricular dimensions, mitral regurgitation, and functional status. A recent meta-analysis estimated a mean improvement of 12% in LVEF after PVC ablation, which was observed in the whole population of patients with frequent PVC and LV dysfunction. The magnitude of improvement is superior to that achieved by other heart failure treatments. Although improvement in LVEF after PVC ablation was initially described in patients with suspected PVC-induced cardiomyopathy, recent studies showed a comparable benefit in patients with previously diagnosed cardiomyopathy and therefore considered to have a “PVC-worsened” cardiomyopathy. Importantly, the recurrence of PVCs (after initially successful ablation and subsequent normalization of LVEF) can again result in a decline of LVEF. Therefore long-term follow-up and reassessment of the PVC burden are recommended in these patients, particularly those whose PVCs were asymptomatic or pleomorphic.
Radiofrequency (RF) ablation now has cure rates of over 90%, which makes it a preferable option, given the young age of most patients with idiopathic VAs. Late recurrence (few months after an acutely successful ablation) of frequent PVCs (from the same area where the original predominant PVCs originated or from a different focus) can be observed in approximately 15% of the patients. Risk of recurrent PVCs after ablation is higher in patients who originally had multiple PVC morphologies, likely suggesting that nondominant PVCs can become dominant PVCs over time. Also, the risk of recurrence seems to be higher in patients in whom the PVCs originated from the papillary muscles.
Implantable Cardioverter-Defibrillator
ICD implantation is recommended in patients with prior cardiac arrest. ICD therapy also should be considered for patients with “malignant” idiopathic VAs that are found to trigger polymorphic VT or VF, especially those presenting with syncope and in whom the PVC trigger cannot be completely eliminated by catheter ablation.
Importantly, for patients presenting with frequent PVCs and LV systolic dysfunction (PVC-induced or PVC-worsened cardiomyopathy) who have a primary prevention indication for ICD implantation, it is advisable to consider therapeutic measures to reduce the PVC burden (such as catheter ablation) before implanting a prophylactic ICD. Elimination of PVCs with ablation has been shown to improve LVEF within a few months in the majority of these patients (especially those with PVC burden of ≥13% at baseline) such that the patients no longer qualify for an ICD. Prophylactic ICD implantation in these patients unfortunately often results in delivery of inappropriate shocks triggered by frequent nonsustained episodes of idiopathic VT. Therefore withholding the ICD and reevaluating within the first 6 months of ablation seems to be an appropriate and safe strategy.
Electrocardiographic Localization of Focal Ventricular Tachycardia
Of all idiopathic VAs, approximately 70% arise from the ventricular OTs, more commonly in the RVOT. Up to 20% to 50% originate in the LV. For LV VAs, the aortic root (the right and left aortic sinuses of Valsalva or the commissure between the two sinuses) is the most common site of origin (70%). The LV summit accounts for 12%, while the LV ostium (septal para-Hisian, aortomitral continuity, and the mitral annulus) accounts for about 5% to 10%. Other less frequent sites of origin include the LV and RV papillary muscles, the moderator band, and cardiac crux ( Table 23.1 ).
Sites of Origin | Prevalence | Mechanism | ECG Findings |
---|---|---|---|
| 70%–80% of idiopathic VT | DAD-related triggered activity | LBBB inferior axis
|
LVOT, aortic cusps, AMC | 16% of outflow tract VT | DAD-related triggered activity | LBBB inferior axis
|
| 10%–15% of idiopathic VT | Macroreentry | Relatively narrow QRS
|
Focal Purkinje VT (Propanolol-sensitive automatic VT) | Rare | Enhanced automaticity | Relatively narrow QRS.
|
| 2.4%–5.2% of idiopathic VT | Enhanced automaticity vs. DAD-related triggered activity | APM: RBBB (q in V1), inferior axis
|
Moderator band | 2.5% of idiopathic VT | Enhanced automaticity vs. DAD-related triggered activity | LBBB with precordial transition later than V 4 or later than sinus rhythm
|
| 8% of idiopathic VT | Enhanced automaticity vs. DAD-related triggered activity | LBBB
|
Mitral annulus | 5% of idiopathic VT | Enhanced automaticity vs. DAD-related triggered activity | RBBB
|
| 1.8%–9.2% of idiopathic VT | Enhanced automaticity vs. DAD-related triggered activity | LBBB, early transition
|
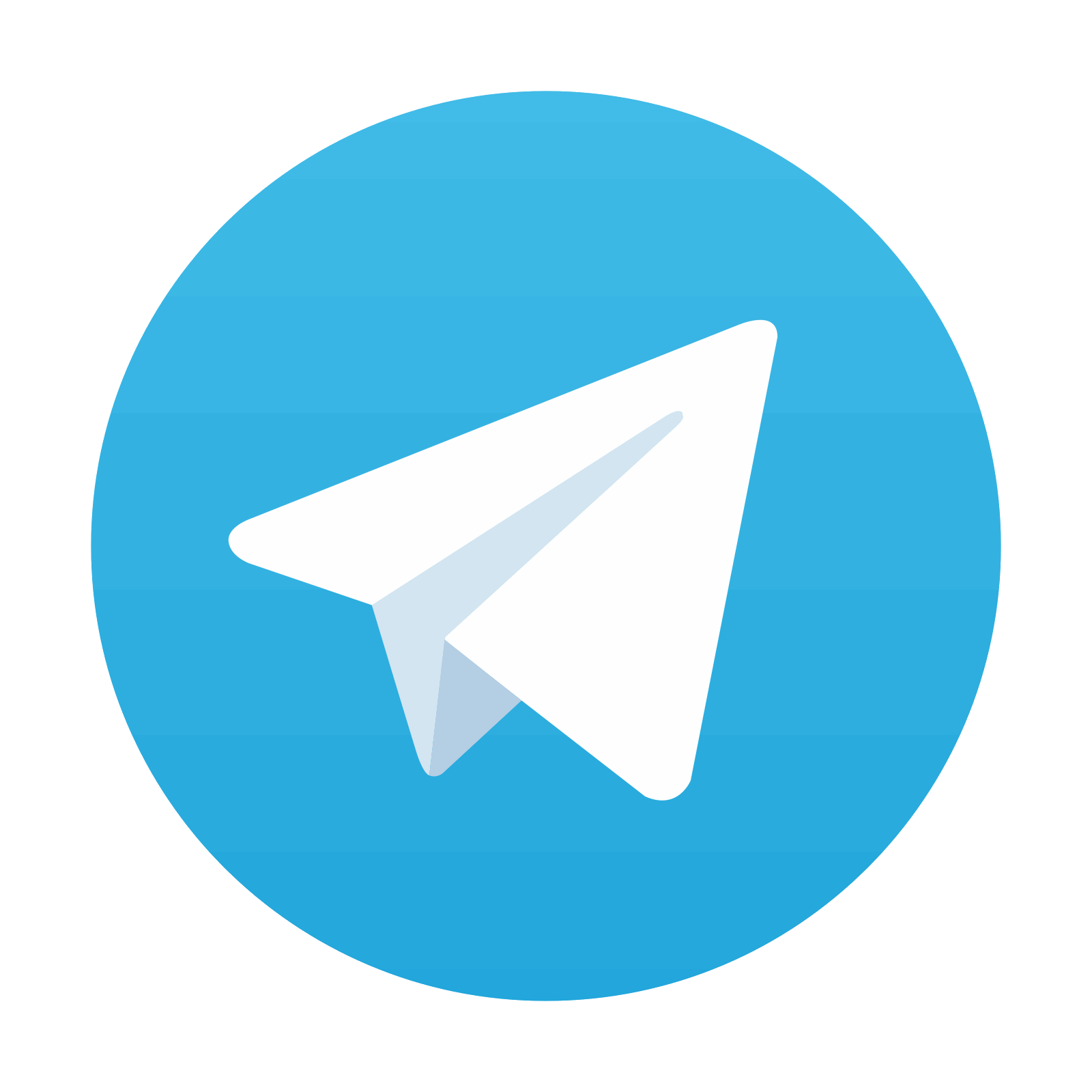
Stay updated, free articles. Join our Telegram channel

Full access? Get Clinical Tree
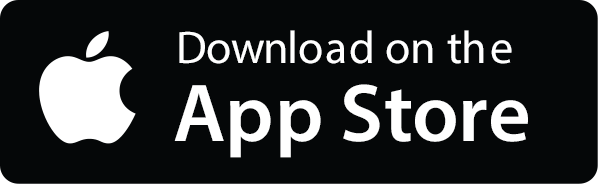
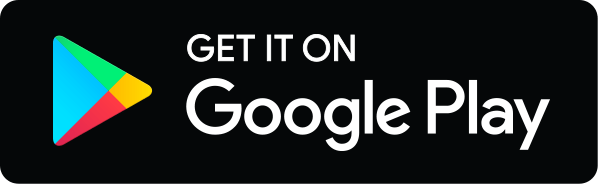
