Background
The aim of this study was to investigate whether strain Doppler echocardiography before reperfusion therapy could quantify ischemic dysfunction and predict viable myocardium in acute myocardial infarction as determined by magnetic resonance imaging.
Methods
Twenty-six patients (mean age, 60 ± 12 years; seven women) with acute myocardial infarctions who underwent acute percutaneous coronary intervention were examined using strain Doppler echocardiography immediately before the procedure. Percutaneous coronary intervention was performed 296 ± 122 min after the onset of pain. Peak left ventricular systolic longitudinal strain and the duration of systolic lengthening were analyzed. Magnetic resonance imaging was performed 11 ± 5 months after therapy. Scarring exceeding 50% of the segment area was considered nonviable.
Results
Peak systolic strain fell gradually (becoming less negative) from normal segments to segments with transmural infarction ( P < .0001), and the duration of systolic lengthening increased ( P < .0001). Myocardial scarring was closely correlated with peak systolic strain ( R = 0.76, P < .00001) and the duration of systolic lengthening ( R = 0.88, P < .00001). There was a significant correlation between the degree of scarring and time to percutaneous coronary intervention ( R = 0.40, P = .045). In segments with systolic lengthening, the improvement in strain after remodeling was significantly higher (5.5 ± 5.1%) than in segments with duration of systolic lengthening > 67% of systole (2.2 ± 3.7%) ( P < .001). Receiver operating characteristic curve analyses showed that duration of systolic lengthening > 67.3% could identify nonviable myocardium (sensitivity, 90%; specificity, 94%).
Conclusions
In patients with acute myocardial infarctions in the anterior wall, strain measurements can identify myocardium with nontransmural scarring. The duration of systolic lengthening is a novel, easily implemented variable that may identify ischemic but viable myocardium. Myocardial infarctions in other left ventricular regions should be investigated in future studies.
The accurate assessment of myocardial function is an important clinical issue in patients with acute myocardial infarctions (AMIs). Revascularization of dysfunctional but still viable myocardium may be of prognostic importance. Approximately 40% of regions showing Q waves or dysfunction in chronic myocardial infarction are not irreversibly damaged, and myocardial function in those regions may be improved by revascularization. Patients with ST-segment elevation myocardial infarctions (STEMIs) and with chest pain should receive reperfusion therapy according to present guidelines. However, patients with AMIs are a heterogeneous group, in which up to one third of those with non–ST-segment elevation myocardial infarctions have occluded culprit arteries, while some patients with STEMIs have no occlusions. Thus, the correct identification of viable tissue in patients with ischemic heart failure could be of great clinical importance.
In a clinical setting, a range of different imaging modalities can be useful in assessing viability. However, none of these methods is suitable in the acute setting, and there is currently no established method to detect viable myocardium during ongoing ischemia. Electrocardiography is currently the only method for the detection of transmural injury in AMI. Q waves, however, should be used with care to distinguish transmural from subendocardial infarcts.
A bedside method to evaluate viability during acute ischemia would be clinically valuable for patient triage, particularly at medical centers without percutaneous coronary intervention (PCI) facilities and for patients without ST-segment elevation or those with infarctions accompanied by atypical clinical features.
The quantification of regional myocardial deformation using strain Doppler echocardiography is feasible as a bedside method in the acute setting. Previous studies have demonstra-ted that strain Doppler echocar-diography can detect myocardial scarring shortly after PCI and after left ventricular (LV) remodeling. Furthermore, strain Doppler echocardiography has improved sensitivity compared with visual assessment of LV function in acute ischemia and dobutamine stress echocardiography for viability assessment. The magnitudes of systolic strain and postsystolic shortening have been introduced as markers of acute ischemia but so far have not proven useful for identifying viable myocardium.
We hypothesized that myocardial strain analyses performed before reperfusion therapy can predict tissue viability in patients with AMIs. Our goal was to analyze the association between passive myocardial lengthening compared with shortening during systole. The nonviable myocardium was expected to remain in a lengthened state during systole for a longer time period compared with active myocardium. The aim of this study was therefore to investigate whether a greater extent of passive myocardial properties indicates a lesser degree of viability.
Methods
Patient Population
To test our hypothesis, we wished to recruit a homogenous group of patients. Twenty-six consecutive patients (19 men, seven women; mean age, 60 ± 12 years) with first acute AMIs in the anterior LV wall who were admitted for PCI treatment were therefore prospectively enrolled. All patients had typical chest pain and sustained ST-segment elevation on electrocardiography. Patients were excluded if they had histories of myocardial infarction or coronary artery disease, and patients with significant valve disease or arrhythmia were not included. Twenty patients (77%) underwent primary PCI, and six (23%) underwent rescue procedures by PCI.
Coronary angiography showed total ( n = 20) or subtotal ( n = 6) occlusion of the left anterior descending coronary artery (LAD), and all patients underwent successful revascularization therapy. All had single-vessel disease, apart from two patients (7.7%) with two-vessel disease. After angioplasty, none of the patients had significant residual stenosis (>50%) in the LAD. Only bare-metal stents were used. The study was approved by the regional committee for medical research ethics. All patients gave written informed consent.
Echocardiography
Examinations were performed using a digital ultrasonographic system (Vivid 7; GE Vingmed Ultrasound AS, Horten, Norway). Patients were examined immediately before reperfusion by PCI, during preparations for the procedure. Care was taken that the echocardiographic study did not delay PCI. A typical echocardiographic examination lasted <5 min. All patients underwent PCI regardless of echocardiographic findings. Doppler tissue imaging recordings of the left ventricle were obtained from the apical long-axis, four-chamber, and two-chamber views (mean frame rate, 117 ± 26 frames/sec); the region of interest (sample size) was 6 × 12 mm. New echocardiographic examinations conducted made after PCI and at the time of magnetic resonance imaging (MRI). The echocardiographic recordings were analyzed using dedicated software (EchoPAC; GE Vingmed Ultrasound AS).
LV ejection fraction was assessed using Simpson’s method from grayscale digital recordings. Peak systolic strain, systolic lengthening (if present), and postsystolic strain index were assessed ( Figure 1 A ). The duration of systole was defined from peak R on electrocardiography to aortic valve closure. Ischemic myocardial segments with dyskinesia were assessed by the ratio of systolic lengthening to total shortening (L-S ratio), as described previously. The different strain variables were measured in the basal part of each LV segment. Furthermore, we introduced a new myocardial viability index, based on the time duration of passive myocardial lengthening as a percentage of total systolic duration. The duration of systolic lengthening was measured as the time interval from end-diastole (peak R in the QRS complex) to the return to zero strain and was reported as a percentage of systolic duration.

All strain values were related to the extent of delayed enhancement by MRI ( Figure 1 A) in the corresponding 16 segments (or 12 segments when only four-chamber and two-chamber views were available).
MRI
MRI was performed using a 1.5-T scanner (Magnetom Vision Plus; Siemens Healthcare, Erlangen, Germany) and a phased-array body coil. No patient had clinical evidence of recurrent coronary events in the period between the initial admission and the MRI study (11 ± 5 months after the AMI). Breath-hold cine images with a time resolution of 35 msec were acquired. Approximately 10 to 20 min after intravenous injection of 0.1 mmol/kg gadopentetate dimeglumine, late enhancement images were obtained from the long-axis views, followed by multiple short-axis slices covering the entire left ventricle. A breath-hold segmented magnetization-prepared turbo gradient-echo sequence was used. The basal and midventricular short-axis slices were each divided into six segments ( Figure 1 B), and the apical short-axis slice was divided into four segments.
In each of the LV segments, the area of the entire myocardium was manually drawn ( Figure 2 ). Infarct was defined as areas with pixel intensities > 2 standard deviations higher than the mean pixel intensity in normal myocardium in the same slice. In each segment, the infarct size was calculated as a percentage of the total segment area. Transmural infarction was defined as 50% to 100% contrast extent of the total segment area, and infarct extent < 50% was defined as viable myocardium. Normal myocardium was defined as tissue with no delayed enhancement. Total infarct size was reported as a percentage of LV myocardial volume and in units of grams of infarcted myocardium.

Statistical Methods
Data are expressed as mean ± SD. The strain measurements were compared with infarct size estimated by MRI using regression analyses. We used one-way repeated-measures analysis of variance with Bonferroni’s correction for comparisons between groups.
Receiver operating characteristic curves were constructed, and areas under the curves were measured to determine cutoff values for optimal sensitivity and specificity with regard to strain and timing. The receiver operating characteristic analyses were set to identify infarcts of ≥50% transmurality of LV segments.
For reproducibility, we randomly selected 10 patients, and two independent observers analyzed strain and the duration of lengthening in each segment. Reproducibility was calculated by determining the coefficient of variation and intraclass correlation (α value). A positive predictive value was calculated to identify nonviable segments. SPSS version 15.0 for Windows (SPSS, Inc., Chicago, IL) was used for all statistical analyses. P values < .05 were considered statistically significant.
Results
Clinical characteristics of the patients are shown in Table 1 . The number of normal segments was 162, and nontransmural infarction was present in 134 segments and transmural infarction in 60 segments. Sixteen percent of all segments were discarded because of low image quality. During follow-up, none of the patients had recurrent angina, but one patient underwent a new PCI procedure because of significant stenoses in nonculprit arteries, found during the acute angiographic study. Thrombolysis In Myocardial Infarction flow grade was reported after angioplasty and was ≥2 in all but two patients with flow grades of 1. The echocardiographic analyses were conducted during acute ischemia, in the PCI laboratory and in the supine position. This resulted in reduced sonographic “circumstances,” primarily in the anterior and posterior wall. At least two segments could be analyzed in the myocardium supplied by the LAD in all patients. Feasibility in all LV segments perfused by the LAD was 87%. Two patients had coronary events during follow-up. They did not have myocardial infarcts but were referred for PCI because of stable angina pectoris.
Variable | Value |
---|---|
Age (y) | 60 ± 12 |
Women/men | 7/19 |
Systolic blood pressure at PCI (mm Hg) | 135 ± 28 |
Diastolic blood pressure at PCI (mm Hg) | 81 ± 15 |
History of diabetes | 1 |
History of hypertension | 7 |
History of smoking | 14 |
History of hypercholesterolemia | 2 |
Time to PCI (min) | 296 ± 122 |
LV ejection fraction (%) | |
Echocardiography | 45 ± 8 |
MRI | 48 ± 13 |
Infarct size by MRI (% of LV volume) | 20 ± 12 |
Infarct size by MRI (g) | 33 ± 24 |
Creatine kinase-MB mass (μg/L) | 331 ± 132 |
Troponin I (μg/L) | 97 ± 84 |
Coronary events during follow-up | 2 events in 2 patients |
Myocardial strain was measured after thrombolysis and before PCI in all patients. Strain was not measured before thrombolytic therapy. The mean time to primary PCI was 289 ± 131 min in those with primary PCI and 330 ± 71 min ( P = .10) in those with primary thrombolysis. The mean scar was 20.8 ± 12.% and 15.5 ± 6.6%, respectively ( P = .19). There was a weak but nonsignificant relationship between time to PCI and the duration of systolic lengthening (R= 0.37, P = .064). Tables 2 and 3 summarize the different strain measurements. Peak systolic strain decreased gradually (becoming less negative) from the normal segments to segments with nontransmural infarction to segments with transmural infarction; however, L-S ratio, the duration of systolic lengthening, and postsystolic shortening increased. The differences among the three groups of segments with transmural injury were highly significant with regard to strain parameters ( P < .0001). The extent of the segmental myocardial scar was closely correlated with peak systolic strain ( R = 0.76, P < .0001), L-S ratio ( R = 0.74, P < .0001), and postsystolic strain ( R = 0.44, P < .0001). The best correlation, however, was found with the duration of systolic lengthening ( R = 0.88, P < .0001) ( Figure 3 ). There was a significant correlation between the degree of scarring and time to PCI ( R = 0.40, P = .045) and between the duration of systolic lengthening and improvement of peak negative strain ( R = 0.44, P < .0001). In segments with short durations of early lengthening (<67% of systole), the improvement in peak negative strain after remodeling was significantly higher (5.5 ± 5.1%) than in segments with durations of systolic lengthening >67% of systole (2.2 ± 3.7%) ( P < .001).
Parameter | 0% | 1%–49% (nontransmural) | 50%–100% (transmural) |
---|---|---|---|
Peak systolic strain (%) | −15.7 ± 5.7 ∗ | −5.7 ± 8.1 ∗ | 4.0 ± 4.7 ∗ |
Post systolic shortening (%) | −0.9 ± 1.9 ∗ | −3.2 ± 4.0 ∗ | −5.7 ± 4.8 ∗ |
L-S ratio | 0.01 ± 0.03 ∗ | 0.18 ± 0.28 ∗ | 0.58 ± 0.30 ∗ |
Duration of systolic lengthening (%) | 1 ± 5 ∗ | 27 ± 33 ∗ | 93 ± 20 ∗ |
0% | 1%–49% (nontransmural) | 50%–100% (transmural) | |||||||
---|---|---|---|---|---|---|---|---|---|
Before PCI | After PCI | Time of MRI | Before PCI | After PCI | Time of MRI | Before PCI | After PCI | Time of MRI | |
Duration of systolic lengthening (%) | 1 ± 5 ∗ | 2 ± 9 † | 0 ± 1 ‡ | 27 ± 33 ∗ | 31 ± 32 † | 5 ± 15 ‡ | 93 ± 20 ∗ | 80 ± 31 † | 22 ± 37 ‡ |
∗ P < .0001, † P < .0001, and ‡ P < .0001 between each group.

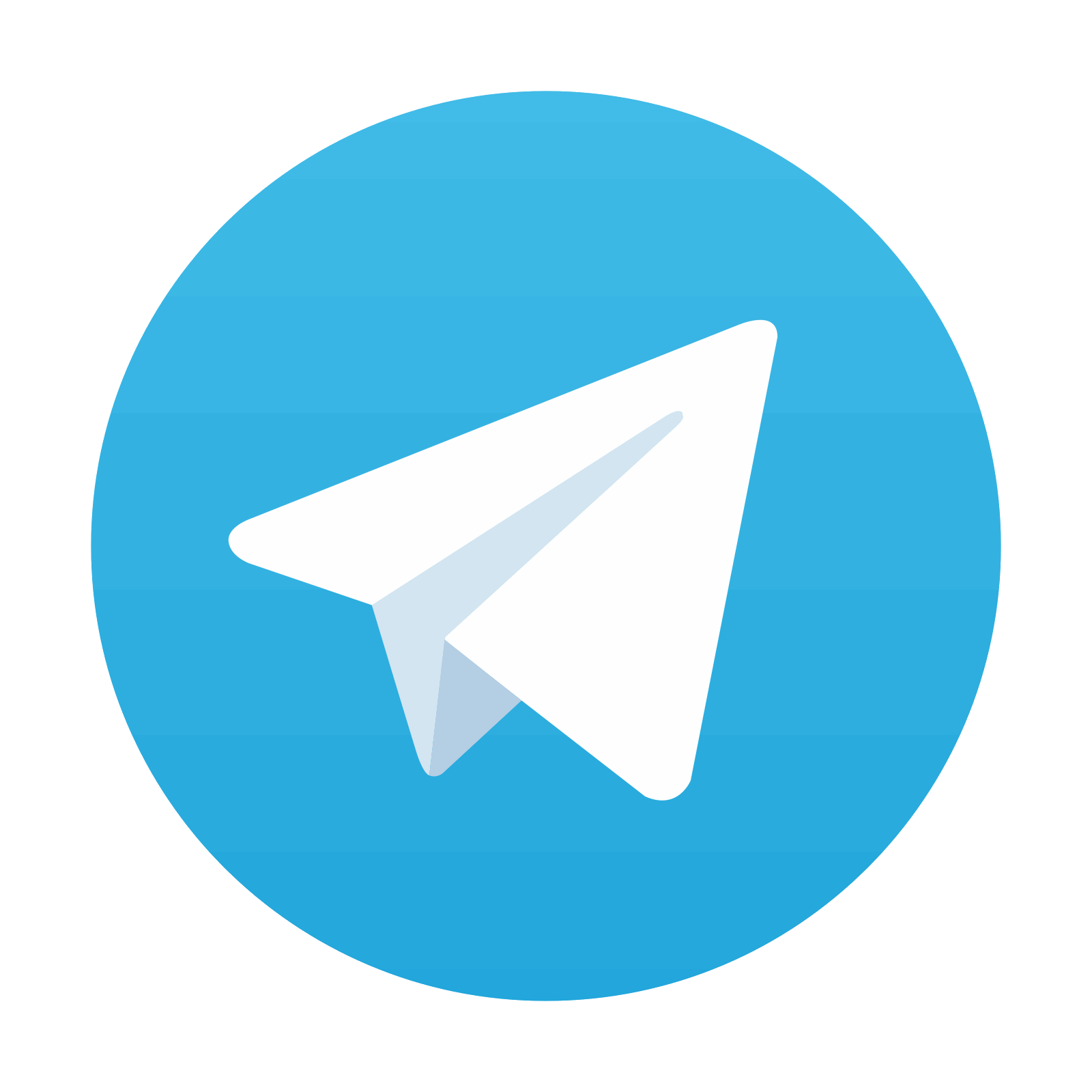
Stay updated, free articles. Join our Telegram channel

Full access? Get Clinical Tree
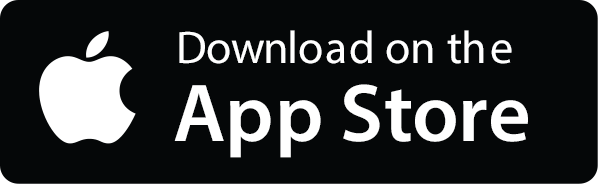
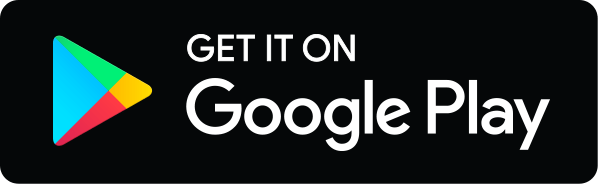
