Abstract
Echocardiographic imaging to detect intracardiac thrombi is commonly done to identify source of systemic embolism and for risk assessment to prevent subsequent embolism. Additionally, intracardiac thrombi are occasionally identified incidentally on echocardiograms done for other indications. Several intracardiac structures can mimic thrombi and embolism may be related by nonthrombotic etiologies as well including tumor, vegetations, and atheromata. The following chapter reviews the etiology, prevalence, risk factors, echocardiographic appearance of intracardiac thrombi as well as the role of follow-up and multimodality imaging in the evaluation of a patient with suspected thrombus. Additionally, risk factors and prevention of thromboembolism are reviewed.
Keywords
Intracardiac thrombus, left atrial appendage thrombus, cardiac source of embolus, echocardiographic detection of intracardiac thrombus
Introduction
Intracardiac thrombi represent a subset of cardiac sources of embolism, are common in a variety of cardiac disease states, and account for 15%–20% of the 500,000 annual strokes in the United States. In addition, they are important sources of systemic emboli to other vascular beds. Thrombi may exist in all cardiac chambers and can either originate from an extracardiac source with migration to the heart (i.e., clot-in-transit) or arise de novo in the cardiac chambers (i.e., left atrial appendage [LAA] thrombi). In the following, we will review the appearance and differential diagnosis of thrombus and other sources of emboli and the echocardiographic methods of detecting thrombi.
Intracardiac Sources of Emboli
Similar to venous thromboembolism, arterial and intracardiac thrombi likely arise from disturbances in flow and vessel characteristics, described by Virchow triad in which there are alterations in blood flow (i.e., stasis), endothelial damage, and inherited or acquired variations in prothrombotic blood constituents. Intracardiac sources of embolism may be divided into thrombotic and nonthrombotic etiologies.
Left Ventricle
Thrombus in the left ventricle (LV) typically is as a result of myocardial infarction (MI) and subsequent local akinesis/aneurysm with stasis and exposure of prothrombotic collagen to the blood pool. As a result, thrombus almost invariably occurs in areas of hypokinesis, akinesis, or dyskinesis of the underlying LV wall segments, most commonly in the setting of an apical aneurysm. It may also occur in the setting of underlying nonischemic cardiomyopathy with reduced global ejection fraction; specific cardiomyopathies (e.g., noncompaction, Chagas, and Loeffler endocarditis) appear more predisposed to thrombus formation, presumably due to local stasis and hypercoagulability. Echocardiography is useful in detecting LV thrombi directly, as well as identifying risk factors for thrombus formation, including wall motion abnormalities and spontaneous echo contrast (SEC). There are caveats: visualization of the endocardial border and therefore clear resolution of thrombus from surrounding trabeculae may be difficult in patients with poor acoustic windows, without the use of echocardiographic contrast. Transesophageal echocardiography (TEE), despite its high resolution, may foreshorten the ventricular apex, and thus transthoracic echocardiography (TTE) is actually preferred for the identification of apical thrombi. Occasionally, even with appropriate contrast opacification, other imaging modalities such as computed tomography (CT) and cardiac magnetic resonance imaging (CMR) may be required to identify thrombus with higher fidelity.
Left Atrium
The LAA represents the most common location for atrial thrombus formation. Ninety percent of thrombi occur in the setting of atrial fibrillation of atrial flutter as a result of disorganized and ineffective contraction of the LAA causing local stasis ( Fig. 38.1 and ). When the LAA ejection velocity, measured using pulsed-wave Doppler 1 cm into the mouth of the appendage, is less than 0.4 m/s, there is an increased risk of thrombus formation ( Fig. 38.2 ). Rheumatic mitral stenosis may also contribute to left atrial (LA) enlargement, stasis, and an increased risk for LA thrombus formation and thromboembolism despite sinus rhythm. The incidence of LA thrombus with mitral stenosis in sinus rhythm is estimated to be 2.4%–13.5%, with risk factors including age greater than 44 years, LA inferosuperior dimension greater than 6.9 cm, mean mitral gradient greater than 18 mm Hg, and dense SEC. Some consider TEE warranted if any of the aforementioned risk factors are present; the absence of SEC on TEE is highly predictive of thrombus absence. In contrast to the stasis and predisposition to thrombus formation seen in mitral stenosis, significant mitral regurgitation has been associated with a lower incidence of thrombus formation and thromboembolization in individuals with rheumatic mitral valvular disease. Owing to the complex three-dimensional anatomy of the LAA with its one to four lobes, trabeculation, and interindividual variability in morphology, distinguishing thrombus from normal anatomical structures by TEE can be difficult, especially in patients with SEC ( Fig. 38.3 ).




Right Ventricle/Right Atrium
Although the same disease processes involved in formation of LV thrombi occur in the right ventricle (RV), the prevalence and specific predictors of RV thrombi have not been identified to date. Certain cardiomyopathies predominantly involving the RV, most notably arrhythmogenic right ventricular cardiomyopathy (ARVC), have been noted in case series to be associated with RV thrombi and may identify a group at risk of RV thrombus formation. Loeffler endocarditis (also called endomyocardial fibroelastosis) is often associated with both right and left ventricular restrictive physiology and thrombi, typically mural and involving the apices of the heart (see Chapter 24 ). In theory, one would presume that there is a higher risk for thrombus formation for mechanical prostheses in the tricuspid position due to the lower ventricular pressures in the RV versus LV, but supportive data are limited. With the increasing use of right-sided intracardiac devices such as pacemaker and defibrillator leads, whose artificial surfaces are predisposed to thrombus formation, RV thrombi are likely to be of greater importance in the future ( Fig. 38.4 and ). Venous thromboembolism that is visualized in the right atrium (RA) or RV, so called clot-in-transit, is high risk for subsequent pulmonary embolism and may appear “sausage-like” in shape on echocardiography, representing a cast of the originating lower extremity vein ( Fig. 38.5 and
; see also Fig. 35.1 and
). The RA appendage (RAA) is broad based compared with the LA and can be a source of pulmonary embolism in setting of atrial fibrillation or atrial flutter.




Prosthetic Valves
With their thrombogenic artificial surfaces, mechanical valve prostheses are common sources of intracardiac embolism, particularly in the tricuspid and mitral positions where chamber pressures are lower and there may be areas of stagnation. Subtherapeutic anticoagulation is a major risk factor. Because thrombus on echocardiography may appear similar to pannus or vegetation, both of which may also affect prosthetic valves, the clinical history (in particular the acuity of onset of symptoms, and any history of lapse in anticoagulant therapy), laboratory data including the international normalized ratio (INR), and echocardiographic characteristics such as mobility are all paramount to proper identification of prostheses-associated intracardiac masses (see Chapter 31 ). Although the risk of thrombus and embolization is higher in mechanical valves, thromboembolism may occur with bioprosthetic valves as well, particularly in the first several months after implantation. Due to shadowing and reverberations from the carbon/titanium valve occluders or supporting rings, identification of prosthetic valve thrombi usually requires TEE for mitral and tricuspid valve prostheses or a combination of TTE and TEE for aortic and pulmonic prostheses. In some cases, no discrete thrombus is visualized but is suggested by an increase in transvalvular velocities, diminished valve clicks, and clinical deterioration ( Fig. 38.6 ). Furthermore, thrombi may be very small and obscured by prosthetic material. Thus, in the absence of other risk factors for cardiac thromboembolism, a prosthetic valve should be considered to be the likely source of embolism in most cases.

Interatrial and Interventricular Septum
Paradoxical embolism of a venous thrombus or mass to the systemic arterial bed (see ) may occur in the setting of an intracardiac septal defect, either at the atrial or ventricular level, with intermittent or continuous right-to-left flow. Similarly, although a patent foramen ovale (PFO) is present in 25%–30% of the population at autopsy, it is noted with higher prevalence in younger adults with stroke. Particularly in association with an atrial septal aneurysm, it may predispose to a higher risk of paradoxical embolism in patients less than 55 years old. However, current data are conflicting on the benefit of PFO closure to reduce this risk, in part due to the overall low rate of recurrence of stroke in those populations without other identified sources of embolus (i.e., cryptogenic stroke).
Nonthrombotic Causes of Embolism
Nonthrombotic masses may also embolize to the systemic arterial bed and are an important consideration on the differential diagnosis of systemic embolization. Primary intracardiac tumors most commonly myxomas and fibroelastomas, infectious or noninfectious vegetations, and complex arterial atheromas (>4 mm thick or mobile) can all embolize (see Chapter 37 , Chapter 39 ). In addition, intracardiac structures such as mitral annular calcification with overlying thrombus may be a rare source of embolism.
Prevalence of and Risk Factors for Left Ventricular and Atrial Thrombi
Prior MI represents the greatest risk for LV thrombus formation, with the likelihood of thrombus formation related to infarct size and location. Patients with large anterior MIs with anteroapical aneurysms are particularly at risk. Using LV ejection fraction as a surrogate for infarct size post-MI, in the Gruppo Italiano per lo Studio della Sopravvivenza nell’infarto Miocardico (GISSI-3) database, individuals with an left ventricular ejection fraction (LVEF) ≤40% had a higher incidence of LV thrombus (17.8% in the setting of anterior MI, 5.4% at other sites) compared with those with a higher LVEF (9.6% in anterior MI, 1.8% at other sites). Although those with an LVEF less than 20% are at highest risk of LV thrombus formation, Doppler echocardiography can be used to identify abnormal spatial flow patterns, predictive of LV thrombus formation. Specifically, persistence of abnormal flow patterns noted at 24 hours post MI, defined as (1) delay between onset of blood motion at the mitral valve and apical level (best demonstrated by high pulse repetition frequency Doppler), (2) a continuous positive Doppler shift near the lateral wall, or (3) continuous negative Doppler shift during the cardiac cycle near the interventricular septum, identified individuals who developed LV thrombus at 3 months. The abnormal Doppler flow patterns are thought to reflect larger ring-type vortices or smaller local apical rotating flow patterns engendered by areas of dysfunctional ventricle.
Most thrombi develop within a median of 5–6 days after MI, with one series suggesting an incidence of 27% at less than 24 hours, 75% by 1 week, and 96% by 2 weeks. In the era of reperfusion, estimates of prevalence appear to have decreased to 4%–17% incidence in several small series of individuals undergoing reperfusion therapy (with either fibrinolysis or percutaneous coronary intervention [PCI]), compared with the 40% incidence noted previously. In a meta-analysis of six trials and 390 patients with prior anterior MI and LV thrombus, fibrinolysis reduced the odds of LV thrombus formation by 0.48 (95% CI 0.29–0.79), likely by reducing infarct size and accelerating endogenous fibrinolysis. The prevalence of LV thrombus after primary PCI for ST elevation myocardial infarction (STEMI) is similar to that seen with fibrinolysis. In one study of 163 patients, TTE performed within 3–5 days after MI had a prevalence of 10.4% thrombus in anterior MI and 4.3% overall. In another study of 1059 patients receiving primary PCI, risk factors for LV thrombus included reduced LVEF, anterior MI, and use of glycoprotein IIb/IIIa inhibitors. It is likely that the prevalence of LV thrombus after MI has been underestimated as the aforementioned studies did not use echocardiographic contrast, used TTE instead of CMR, which have shown higher rates of detection of LV thrombi, and excluded those with severe heart failure.
The prevalence for LV and LA thrombus may vary according to comorbid and genetic risk, as well as medication compliance. For example, although LAA thrombi are more common in those with atrial fibrillation, this risk varies according to presence and adequacy of anticoagulation and comorbid conditions known to predispose to LV and LA thrombi (e.g., heparin-induced thrombocytopenia and polycythemia vera). Furthermore, prothrombotic tendencies that show a strong familial inheritance but are not yet been localized to single mutations may account for the increased risk of thrombus formation in individuals in whom no etiology for thrombus formation has been identified. In addition, specific disease states, in particular the cardiomyopathies associated with diminished LV systolic function, are associated with a higher LV thrombus risk. In noncompaction cardiomyopathy, developmental failure of compaction of the spongiform myocardium contributes to diminished LV systolic function and large intramyocardial recesses in continuity with the LV chamber. Resulting stasis and thrombus formation are more common ( Fig. 38.7 ; see also ). Thus individuals with noncompaction cardiomyopathy may be at higher risk for systemic embolization. Certain cardiomyopathies associated with apical akinesis or aneurysm (i.e., Chagas disease, hypertrophic cardiomyopathy with apical aneurysm, and Takotsubo cardiomyopathy) are associated with a risk of thrombus formation and embolization (see Chapter 22 ). Furthermore, cardiomyopathies in which there is disturbance of the blood-endocardium barrier (e.g., Loeffler endocarditis, hypereosinophilic syndromes, and ARVC) are also at risk.

Other imaging modalities may be more sensitive than TTE for identification of thrombus and should be considered if further imaging will change the overall treatment plan. In one study, late gadolinium enhancement CMR identified thrombi in 21% of 57 patients with prior MI or ischemic cardiomyopathy versus only 8.8% identified by TTE with the nonvisualized thrombi primarily located at the LV apex. Another retrospective study of 160 patients with remote MI and confirmed LV thrombus at surgery or autopsy, the sensitivity of LV thrombus with CMR was 88%, versus 23% with TTE and 40% with TEE. CT angiography has evolved to become a useful modality, particularly for visualizing abnormalities in aortic mechanical prostheses, which can be difficult to visualize on both TTE and TEE due to acoustic shadowing (see Chapter 48 ).
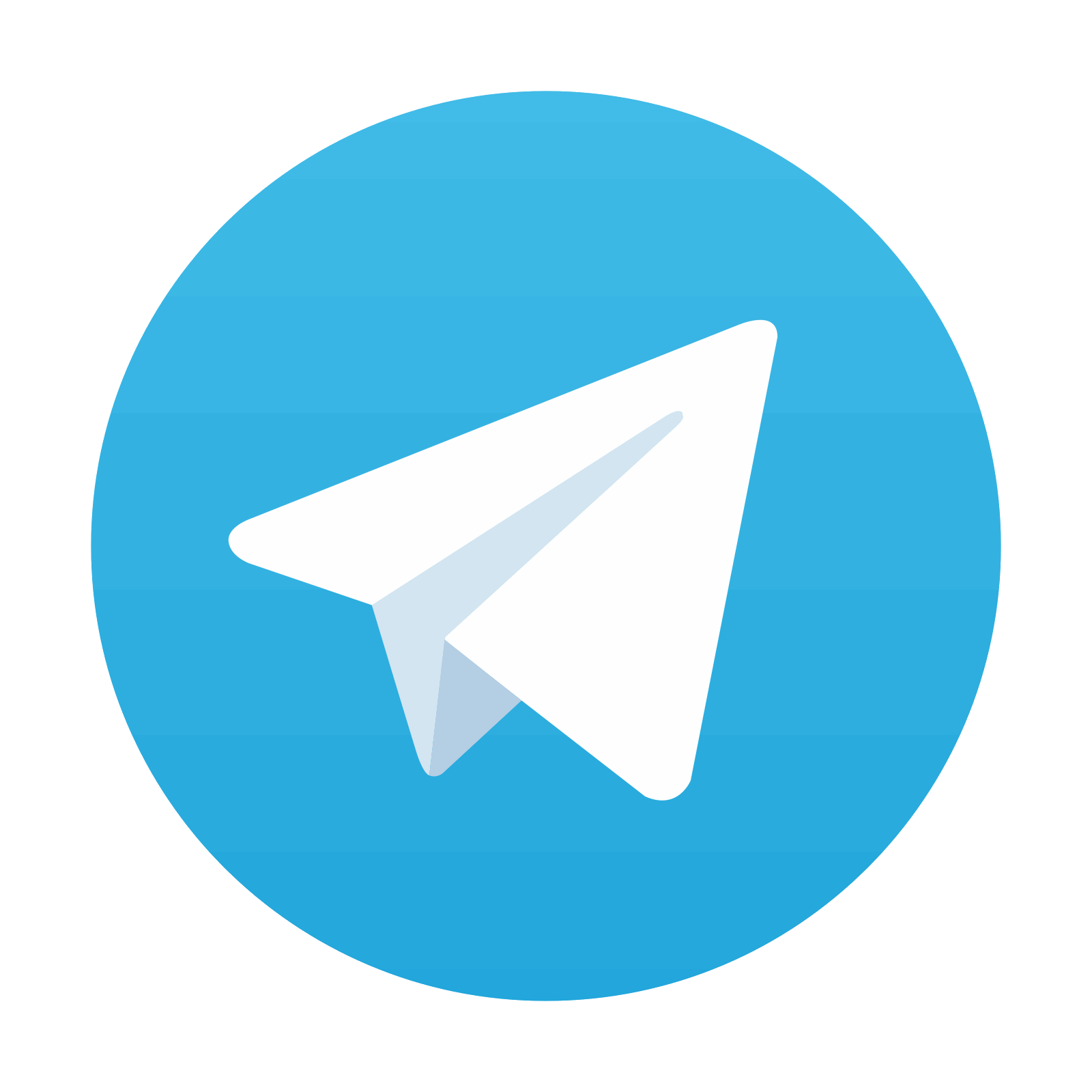
Stay updated, free articles. Join our Telegram channel

Full access? Get Clinical Tree
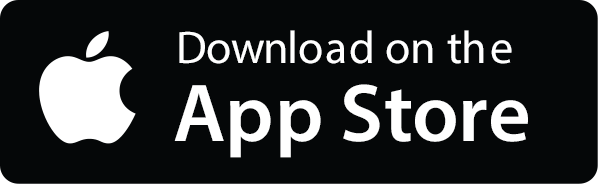
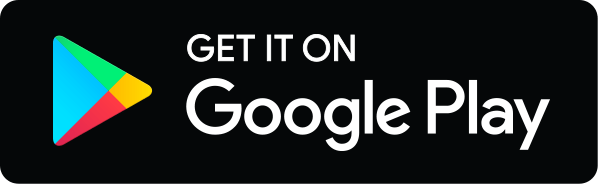