Fig. 51.1
Mechanisms that regulate CSPC migration (a), differentiation (b), and proliferation (c). CXCR4 C-X-C chemokine receptor type 4, SDF-1 stromal cell-derived factor-1, sVCAM soluble vascular cell adhesion molecules, SCF stem cell factor, NICD Notch1 intracellular domain, RBP-J J kappa-recombining binding protein, TGF-β1 transforming growth factor beta 1, HIF-1α hypoxia-inducible factor 1α, IGF-1 insulin-like growth factor 1 (see text for detailed discussions)
Survival
Survival of stem cells is one of the main barriers to effective cell therapy. The hostile myocardium environment, such as persistent ischemia and inflammation, challenge the survival of all types of cells. It has been observed that within a day of myocardial infarction, 40 % of resident cardiac progenitor cells are depleted [13], emphasizing the importance of enhancing the survival of CSPCs. Lu et al. demonstrated that pretreatment of Sca-1+ cells with IGF-1 can promote cell survival both in vitro and in vivo [40]. The survival rate of the pre-conditioned Sca-1+ cells was 5.5 fold higher than that of the non-preconditioned cells. In addition, Konstantinos et al. reported that transplantation of bone marrow-derived mesenchymal stem cells (MSCs) can stimulate the survival of the host endogenous c-kit+ CSPCs after infarction, and the improvements of CSPC survival lead to amelioration of cardiac function [41].
Homing and Recruitment
When administered intravenously or through coronary artery, the CSPCs need to home to the damaged cardiac tissue, whereas at local tissue, the densely packed cardiac cells, extracellular matrix, and replacement fibrotic tissues impose a significant physical barrier to the cell recruitment. Compelling evidence now suggests that enhancement of CSPC migration can enhance the repair of ischemic heart. Goichberg et al. demonstrated that Ephrin A1, through interacting with EphA2 receptor, promotes the motility of cardiac stem cells in vitro and their migration to the area of damage in vivo [42]. Liang et al. found that upregulation of SDF-1 in the infarcted myocardium induces migration of Sca1+CD31− cardiac SP cells [43]. In addition, the soluble VCAM-1 (sVCAM-1) has also been shown to exert a positive effect on the migration of cardiac stem cells [44]. Short exposure to hypoxia has also been shown to increase the migration of CSPCs [45]. All of these studies suggest the importance of homing and recruitment of the CSPCs in cardiac repair and may provide a therapeutic strategy in the treatment of ischemic heart diseases.
Hypoxic Preconditioning of Cardiac Stem/Progenitor Cells
Hypoxia is the most common pathological state of ischemic diseases including ischemic heart disease, and severe hypoxia causes cardiac cell death. However, accumulating evidence now suggests that hypoxia has a variety of effects on embryonic and adult stem cells, from proliferation and pluripotency maintenance to adaptive stress response, which are largely dependent on the O2 level, time of exposure, and specific tissue microenvironment [46]. Hypoxic preconditioning of stem cells can induce a series of protective responses that adapt these cells to the hostile environment of damaged tissues; therefore, it can be employed as a strategy to enhance the effectiveness of cell therapy.
General Mechanisms of Hypoxic Preconditioning in the Regulation of Stem/Progenitor-Cell Function
It is well established that preconditioning of hearts with mild ischemia/hypoxia stimuli can significantly limit the damage from a subsequent serious cardiac ischemia such as myocardial infarction. However, how hypoxic pre-treatment of stem/progenitor cells affects their functional capacity is incompletely understood, and a number of molecules and signaling pathways have been reported to be involved in the process.
Hypoxia-Inducible Factor-1 (HIF-1)
The HIF-1 transcription factor is known as a master regulator for cells to sense O2 levels in the tissue microenvironment and mount an appropriate cellular response to hypoxia [47]. Its pivotal role in the hypoxic preconditioning is well supported by a large body of literature [48]. Hypoxia results in a reduced degradation of HIF-1a via the proteasome pathway; the accumulated protein induces the expression of a diverse range of target genes, including erythropoietin, vascular endothelial growth factor, and Bcl-2 family members, which augment stem cell function [49, 50] and markedly enhance stem cell survival under severe hypoxia, oxidative stress, or in the inflammatory environment [51–53].
Akt
Akt is a hub of several important survival pathways in many types of cells. Recently, it has been shown to participate in hypoxic preconditioning of stem cells. Phosphorylation (activation) of Akt is significantly increased in adipose-derived stem cells during hypoxic preconditioning, and inhibition of Akt abolishes the protective effect of the hypoxic preconditioning [54]. Similarly, hypoxic treatment of mesenchymal stem cells (MSCs) results in an upregulation of several pro-survival and angiogenic factors, including HIF-1a, VEGF, and the phosphorylated Akt [55, 56]. These data suggest that Akt is critical for the protective effect of hypoxic preconditioning [57].
Mitogen-Activated Protein (MAP) Kinases
It has been shown that the intermittent hypoxia-induced delayed cardioprotection is mediated by p38 MAPK and ERK 1/2 and blocked by p38 MAPK inhibitor [58]. This indicates that the protective effect of hypoxic preconditioning may be, at least partially, dependent on MAPK pathways. In addition, JNK and ERK have been reported to exert an anti-apoptotic effect of ischemic/hypoxic preconditioning on stem cells and regulate cell function by interacting with additional molecular pathways [59]. Furthermore, inhibition of ERK attenuates the beneficial effect of ischemic preconditioning on the pig myocardium [60]. In addition to Akt and MAPKs, protein kinase C (PKC) has also been shown to play a role in ischemic preconditioning in the rat heart [61].
Endothelial Nitric Oxide Synthase (eNOS)
eNOS is an important regulator of stem/progenitor cell biology as well as vascular function. Uemura et al. found that hypoxic treatment significantly increases the level of eNOS in bone marrow stem cells. When injected into the infarcted myocardium in mice, the hypoxic pretreated bone marrow cells exhibit better survival than control non-treated cells [62]. Interestingly, Ii et al. demonstrated that endothelial progenitor cells (EPCs) are recruited to the ischemic pre-conditioned myocardium and exert a protective effect through NOS expression [63].
Iron Channels
Hu et al. demonstrated that hypoxic preconditioning can increase the migratory capacity of bone marrow mesenchymal stem cells via upregulation of Kv2.1 expression and FAK activity [64]. The importance of calcium was also noted by another study in which Yu et al. found that calcium/calmodulin-dependent protein kinase II is critical for the beneficial effect of intermittent hypoxic preconditioning in the ischemic-reperfusion-induced cardiac dysfunction [65]. Thus, iron channels may participate in the hypoxic preconditioning in ischemic heart diseases.
Taken together, these experimental findings provide a general understanding of the mechanisms involved in hypoxic preconditioning of stem/progenitor cells. However, more in-depth investigations are warranted to unravel the molecular details.
Hypoxic Preconditioning of Cardiac Stem/Progenitor Cells (CSPCs)
Compared with other types of stem/progenitor cells, CSPCs seem to be the obvious choice for cell-based cardiac repair. However, the success of this approach is determined in part by the same factors that cause the endogenous cardiac repair system to fail. Within a day of myocardial infarction, 40 % of resident CSPCs are depleted [13], and the barriers imposed by cardiac damage may limit the proliferation and self-renewal of the remaining resident cells, thereby preventing restoration of the progenitor-cell pool. At present, these limitations are often addressed by using ex vivo expansion protocols to generate a sufficient number of cells for transplantation into the ischemic heart. However, only a very small number of transplanted stem/progenitor cells are retained in the ischemic myocardium [66], and poor cell retention is one of the primary barriers to the effectiveness of cell therapy [67]. Thus, techniques that enhance the recruitment and retention of transplanted stem/progenitor cells are crucial to adequately replenish the resident progenitor cell pool and to maximize its regenerative potential. Toward this goal, we have initiated a series of studies investigating the effect of hypoxic preconditioning on the recruitment of intravenously administered cells to ischemic heart tissue and the preservation of heart function in murine models of myocardial infarction.
To enrich potent CPSCs, we selected the hematopoietic-lineage-negative (Lin−) and c-kit-positive (c-kit+) cells (CLK cells) from cardiospheres grown from explants of adult mouse hearts [29]. Because CXCR4 plays a critical role during SDF-1-mediated recruitment of circulating stem/progenitor cells to ischemic myocardium [68, 69], we evaluated the expression of CXCR4 in CLK cells. When cultured under normoxic conditions, CXCR4 expression was rather modest; however, both the number of cells expressing CXCR4 and the amount of CXCR4 protein expressed increased rapidly after just 4 h in hypoxic culture [29]. The elevation in CXCR4 expression was preceded by an increase in the expression of HIF-1α, and transfection of CLK cells with a HIF-1α small-interference RNA (siRNA) diminished hypoxia-induced CXCR4 expression. Furthermore, the hypoxia-induced CXCR4 expression is associated with a markedly increased CLK-cell migration toward SDF-1, and when CXCR4 expression was knocked down by infecting CLK cells with a lentiviral vector encoding CXCR4 shRNA, the hypoxia-induced enhancement of CLK-cell migration was abolished. Collectively, these observations indicate that the migratory activity of CLK cells is increased by hypoxia and that the mechanism of enhancement is dependent on an HIF-1α-mediated increase in CXCR4 expression.
To determine whether hypoxia-induced CLK-cell migration was accompanied by enhanced CLK-cell recruitment to ischemic myocardium, and to assess the differentiation of the recruited CLK cells, CLK cells were transduced with retroviral vector pCL-MFG-LacZ (CLK-LacZ cells). Hypoxic preconditioning was associated with a ∼2.5-fold increase in CLK-cell recruitment to ischemic myocardium as assessed 24 h after CLK cell injection. Importantly, pretreatment of CSPCs with CXCR4 antagonist AMD3100 during the course of hypoxic preconditioning diminished the increase in CLK-cell recruitment, suggesting that the SDF-1/CXCR4 axis plays a pivotal role (Fig. 51.2). Our observation is supported by studies reported by van Oorschot et al., which also demonstrated that short-term hypoxia increased the migratory and invasive capacities of CSPCs [45]. In addition, we assessed CLK cell differentiation at 4 weeks after injection of lacZ-transgenic CLK cells; histological analyses revealed that the lacZ+ cells in the ischemic myocardium co-expressed cardiac troponin I, von Willebrand factor, and smooth muscle actin, indicating that the transplanted CLK cells had differentiated into cardiomyocytes, endothelial cells, and vascular smooth muscle cells, respectively. Therefore, hypoxic preconditioning enhances CLK cell migration, recruitment, and differentiation.
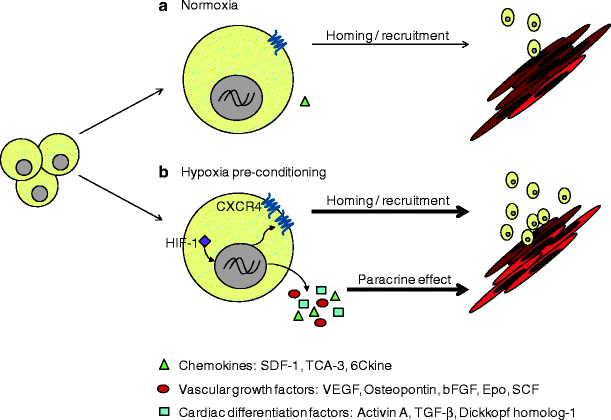
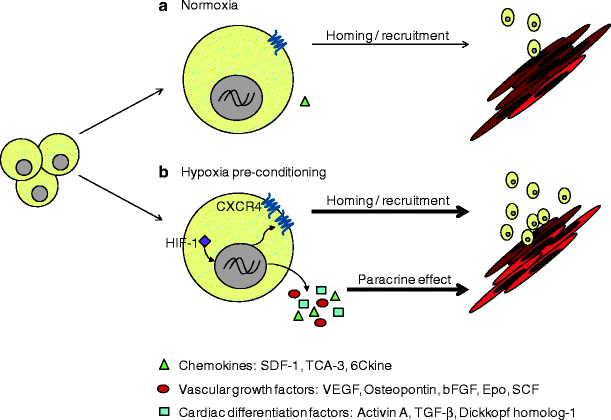
Fig. 51.2
Hypoxic preconditioning of CLK cardiac stem cells enhances repair of the infarcted myocardium. (a) Hypoxic pretreatment of CLK cardiac stem cells upregulates the expression of CXCR4 and SDF-1 through HIF-1 and augments the recruitment of CLK cells to the ischemic myocardium. (b) Hypoxic pretreatment also increases the secretion of paracrine factors that promote chemotaxis, vascular growth, and cardiac differentiation. TCA-3, also named chemokine (C-C motif) ligand 1, CCL1, or I-309; 6Ckine, also named chemokine (C-C motif) ligand 21A, CCL21A, secondary lymphoid-tissue chemokine (SLC), or Exodus-2; Epo, erythropoietin
After observing the dramatic hypoxia-induced increase in CLK-cell recruitment to ischemic myocardium, we investigated whether hypoxic preconditioning enhanced the therapeutic benefit of CLK cells. At week 4, functional analyses indicated that both left ventricular fractional shortening and the ejection fraction were better preserved in mice administered hypoxic-preconditioned CLK cells than in mice treated with normoxia-cultured CLK cells. Histological analyses indicated that peri-infarct capillary density and infarct wall thickness in the left ventricle were significantly greater, and infarct size was significantly smaller, in hearts from mice administered hypoxic-preconditioned cells than from mice treated with normoxia-cultured cells, and hypoxic-preconditioned cells were also associated with lower heart weights and a lower heart-weight:body-weight ratio. Collectively, these results indicate that the therapeutic benefits of CLK-cell administration after myocardial infarction are enhanced by hypoxic preconditioning.
The ischemic myocardium is known to produce an abundance of chemo-active, -attractive, or -repulsive, agents [70, 71], and these chemotactic signals, along with specific cell-matrix and cell-cell interactions, modulate the regenerative capacity of progenitor cells [67]. Accordingly, we performed protein array experiments to compare the levels of more than 300 mouse cytokines released from hypoxia- and normoxia-treated CLK cells into the culture medium. Hypoxic treatment was associated with higher levels of numerous factors, including chemokines (e.g., TCA-3, SDF-1, 6Ckine), vascular growth factors (e.g., VEGF, Osteopontin, bFGF, EPO, SCF), and factors involved in cardiac differentiation (e.g., Activin A, TGF-β, and Dkk-1), which also contribute to the cardiac repair (Fig. 51.2). The increase in SDF-1 secretion by CLK cells is particularly interesting. Recent studies have shown that SDF-1 is cardioprotective and that pretreatment of BM-derived mesenchymal stem cells (MSCs) with SDF-1 enhances cell survival, proliferation, and engraftment, and improves cardiac function after myocardial infarction in animals [72–74]. Our results indicate that SDF-1 is one of the chemokines and growth factors secreted by hypoxia-preconditioned CLK cells, which suggests that the apparent benefit associated with systemically administered hypoxia-preconditioned CLK-cells evolves, at least in part, through paracrine mechanisms.
Outlook
Ischemic heart disease and consequent heart failure remain the leading cause of morbidity and mortality worldwide; traditional therapies can only relieve symptoms and slow the disease progress. In the past decade, stem/progenitor cells have emerged as an optimal strategy of treatment, and CSPCs, in particular, can directly regenerate cardiac tissue by inducing neovasculogenesis and cardiogenesis. At present, the regenerative capacity of CSPCs is rather limited, and their clinical uses are still at infancy. One of the major barriers to the successful CSPC therapy is the hostile environment of the infarcted cardiac tissue, which quickly depletes endogenous CSPCs and limits the expansion and differentiation of the exogenously administered cells.
Recently, hypoxic preconditioning has emerged as a promising strategy to improve CSPC therapy. Studies from our laboratory and others have shown that hypoxic pretreatment of CSPCs significantly increases their migratory capability and survival, and when administered systemically, the hypoxia-preconditioned CSPCs homed to the infarcted myocardium more efficiently than normoxia-cultured cells, which lead to significantly improved cardiac function and reduced infarct size. Hypoxia activates CSPCs through multiple mechanisms in which HIF-1α-mediated SDF-1/CXCR4 upregulation appears to play a critical role.
It is well known that during ischemia, hypoxia stimulates a series of adaptive cellular responses that favor the survival of cardiomyocytes, and transient episodes of ischemia have been shown to protect the ischemic heart. Thus, patients experiencing a cardiovascular event could benefit, at least theoretically, from ischemic preconditioning, but this cardioprotective strategy has yet to be effectively employed clinically. One of the primary barriers limiting the effectiveness of ischemic preconditioning is the need to apply this strategy before the ischemic event occurs rather than after the unanticipated onset of myocardial ischemia experienced by patients who present with acute myocardial infarction or who survive cardiac arrest. Hypoxic preconditioning may be a more viable therapeutic approach, because the cellular components could be obtained and preconditioned with hypoxia in advance for administration after a subsequent ischemic event.
Acknowledgements
This work was supported by NIH grants R01 HL093439 and R01 HL113541 and American Heart Association grant 0430135N (to G.Q.) and the State Scholarship Fund of China (to S.X.).
References
1.
2.
3.
4.
Wehrens XH, Doevendans PA. Cardiac rupture complicating myocardial infarction. Int J Cardiol. 2004;95(2–3):285–92.PubMedCrossRef
< div class='tao-gold-member'>
Only gold members can continue reading. Log In or Register a > to continue
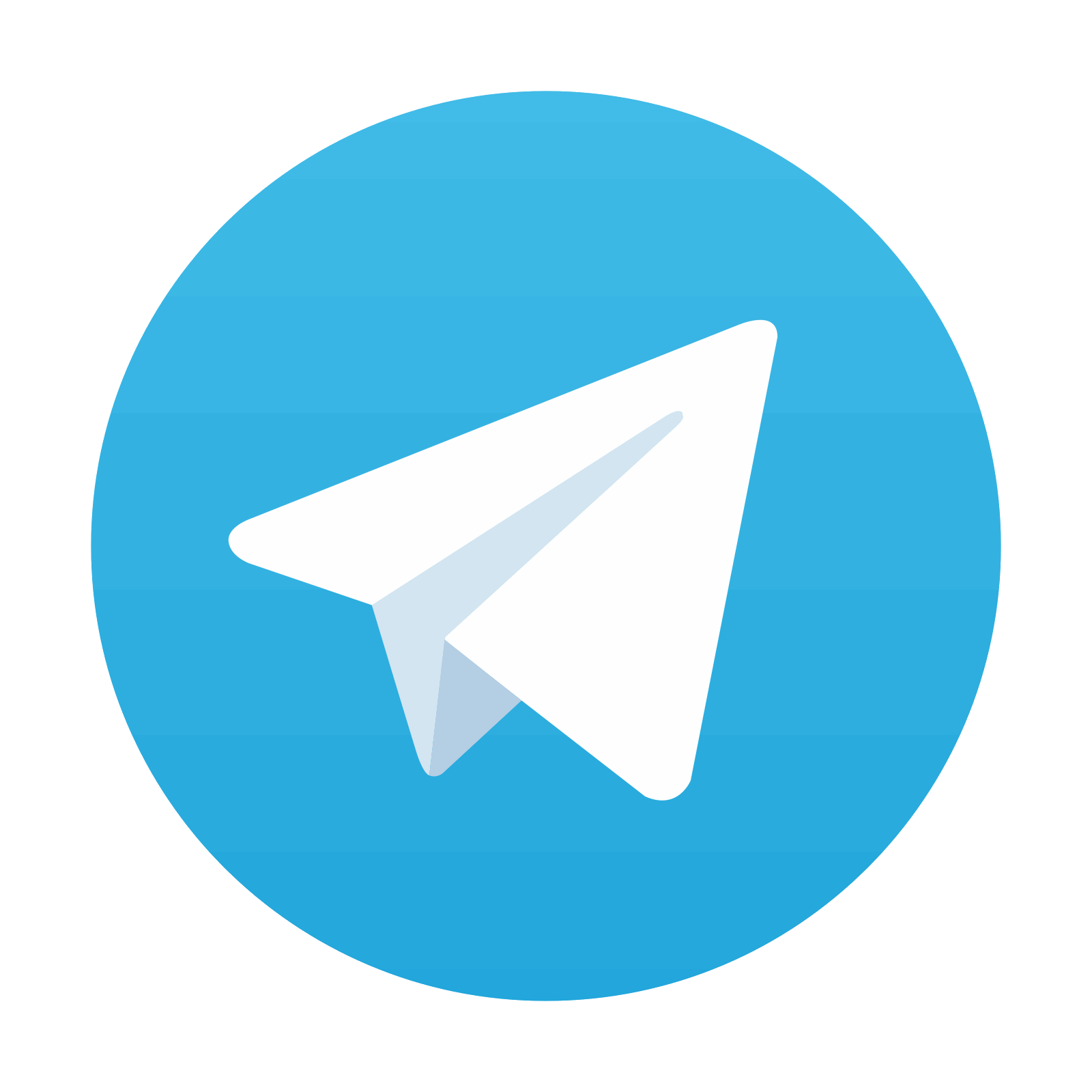
Stay updated, free articles. Join our Telegram channel

Full access? Get Clinical Tree
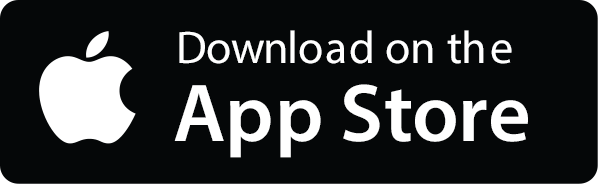
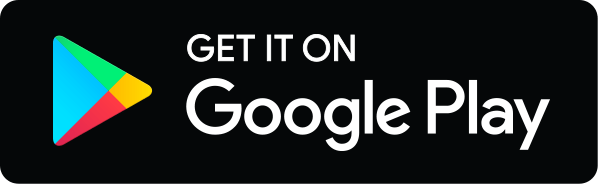