Rather than being a single lesion, the entity usually described as hypoplastic left heart syndrome is a group of conditions with characteristic anatomical, physiological, and clinical properties. The earliest grouping of these features was made by Lev, 1 but he described the syndrome as hypoplasia of the aortic tract. Hypoplasia of the left heart is not always the same as aortic atresia or hypoplasia, since the aortic lesions can exist with a left ventricle of normal size, particularly when the aorta takes its origin from the morphologically right ventricle. As far as we are aware, it was Noonan and Nadas 2 who first used the term hypoplastic left heart syndrome. This is the term now generally accepted for description, despite the concerns that some have with regard to the definition of a syndrome. Geneticists define a syndrome as involving organs of several systems. Patients with the classical picture of hypoplasia of the left heart rarely have syndromic problems elsewhere in their bodies. If we wish to be specific, therefore, it is better to use hypoplasia of the left heart to describe the anatomical features of the entity to be discussed in this chapter. It is most unlikely, nonetheless, that those who diagnose and treat the entity will call it anything other than hypoplastic left heart syndrome, so we anticipate that the classical term will retain its currency. 3
Although the combinations of anatomical lesions that constitute the syndrome are variable, the features when seen together are unmistakable. Thus, the syndrome includes the majority of cases of aortic atresia, and most cases of atresia of the left atrioventricular valve. Aortic atresia, nonetheless, when associated with deficient ventricular septation, can be found with a left ventricle of normal size. In these cases, the aorta may be in potential connection with the morphologically left ventricle. The atretic aorta may also be connected potentially to the morphologically right ventricle when the ventriculo-arterial connections are essentially discordant. In the latter setting, of course, the morphologically left ventricle is dominant rather than hypoplastic. In most of the cases of left atrioventricular valvar atresia with right hand ventricular topology, it is the left ventricle which is hypoplastic. Patients with hearts of this type fall within the group of classic cases when the ventricular septum is intact. When both arteries take their origin from the morphologically right ventricle in this setting, the aorta may be of good size despite the left ventricular hypoplasia. Furthermore, should the ventriculo-arterial connections be discordant, the left ventricle can again be hypoplastic. With such discordant ventriculo-arterial connections, the small left ventricle is in potential communication with the pulmonary trunk rather than the aorta. In the rare instances of this combination existing with an intact ventricular septum, the combination will present clinically as pulmonary atresia, although the ventricular morphology is more representative of hypoplasia of the left heart. The left ventricle, aortic valve, and ascending aorta may all also be hypoplastic in the context of atrioventricular septal defect with common atrioventricular junction when there is right ventricular dominance. Patients with this combination are discussed in Chapter 36 . In most of the cases initially studied by Noonan and Nadas, 2 aortic coarctation was the major anatomical feature, although the left ventricle was described as being hypoplastic. It is questionable whether such cases would nowadays be considered as examples of hypoplastic left heart syndrome, albeit that examples of the classical syndrome are held to exist with mitral and aortic valves of normal dimensions relative to the co-existing hypoplasia of the left ventricle. Some, therefore, describe these cases as a complex rather than a syndrome, and maintain that biventricular repair is possible following simple relief of any obstruction within the aortic arch and descending aorta. 4
It follows from all the above discussion relating to different morphological patterns that there are major difficulties in providing an all-encompassing anatomical definition for hypoplasia of the left heart. It is more realistic, therefore, to analyze the unifying haemodynamic features. In physiological and clinical terms, hypoplasia of the left heart can be defined as the situation in which the systemic circulation is dependent on the morphologically right ventricle in the setting of atresia or severe hypoplasia of the aortic valve. 5 For the purposes of this chapter, we will follow this definition, dealing exclusively with those lesions having a small morphologically left ventricle, an intact ventricular septum, and either atresia or critical stenosis of the aortic valve.
EPIDEMIOLOGY
Before fetal echocardiography routinely became a part of antenatal screening, the reported incidence of the hypoplastic left heart syndrome ranged from 0.16 to 0.36 per 1000 live births. 6,7 In many countries, screening to establish normality of the four cardiac chambers is now routinely performed during antenatal ultrasonography, and the diagnosis of hypoplasia of the left heart is often made by non-cardiologists. Some parents, learning of the diagnosis prior to 20 weeks of gestation, will opt for termination of the pregnancy. This contributed, in some areas of the world, to a decline in the number of neonates born with the syndrome. 8 In this respect, although the syndrome is not a common form of congenital cardiac disease, in the past it generated a large proportion of the cardiovascular mortality occurring in the first month of life, since prior to the late 1980s, there was no treatment for the condition. It remains to be seen, with the improvements in surgical treatment to be discussed in this chapter, whether the observed decline in incidence because of termination of pregnancy will continue. In support of this speculation, a recent report from the United Kingdom demonstrated a reduction in the rate of termination of pregnancy of 50%. 9
Of those surviving to term, two-thirds are male. It has been estimated that the risk of recurrence in siblings is 0.5%, with a risk of 2.2% for the occurrence of other congenitally malformed hearts. 10 The syndrome can occur in the setting of Turner’s syndrome, or its mosaic variant. It can also accompany Noonan’s syndrome, microdeletion of chromosome 22q11, Holt–Oram syndrome, Edward’s syndrome, and Jacobsen syndrome, and can be found with other chromosomal abnormalities, including trisomy 13, trisomy 18, and deletions of chromosomes 4q, 4p, 11q, and 18p. It has also been associated with different mendelian syndromes, but without a robust genetic association. With the advent of fetal echocardiography, it has become evident that recurrence of obstructive lesions in the left heart is particularly high in mothers referred with a family history of congenital cardiac disease. Such observations support a genetic predisposition to the development of such lesions. Epidemiological evidence in favour of this notion was provided by the Baltimore–Washington infant study. 11 It is also increasingly recognised that first-degree relatives of probands with hypoplasia of the left heart are at increased risk of less severe abnormalities of the left heart. In two recent reports from the United States, a bicuspid aortic valve was identified in 5% to 11% of first-degree relatives of affected probands. 12,13
AETIOLOGY
The aetiology of hypoplasia of the left heart is unknown in most instances. Associations with chromosomal or extracardiac defects are inconsistent, but more common than with many other forms of congenital cardiac malformations. Thus far, they have failed to refine our understanding of the genetic precursor of the disease, although recent work suggests that the basic helix-loop-helix transcription factors may play a role. 14 Most current theories on the aetiology of hypoplastic left heart syndrome are based on autopsy findings, along with echocardiography, particularly fetal echocardiography. It is examination of the heart during the latter trimesters of gestation that has better enabled us to define its normal and abnormal development. In those eventually developing the syndrome, evidence of impaired growth of the left ventricle has been associated with diminished inflow or obstruction to outflow, although this does not become obvious until after 20 weeks of gestation. 15
Experiments using chick embryos showed that hypoplasia of the left ventricle could be produced following intervention to occlude the mitral valve. 16 This is unlikely to underscore development of the syndrome in humans, as mitral stenosis occurring as a primary lesion results in enlargement, rather than hypoplasia, of the left atrium. Displacement of the primary atrial septum has been noted in autopsied specimens, 17 and has been presumed potentially to reduce flow through the inferior caval vein via the oval foramen to the left atrium and left ventricle, resulting in left ventricular hypoplasia. The displaced septum could equally be the consequence of the ordered flow. Indeed, studies of flow during fetal life suggest that it is likely that the ventricular problem occurs first. 18 Obstruction to the outflow from the left ventricle is always present, and it, too, has been implicated as an aetiological factor. 19 Ventricular endocardial fibro-elastosis is prominent when the mitral valve is patent. In these patients, there can be little doubt that the high left ventricular pressure, particularly in diastole, is an important additional aetiological factor in the development of the endocardial ischaemia and scarring. In the light of the varied anatomical findings to be described below when the left ventricle is hypoplastic, it is unlikely that a solitary aetiological factor unifies their development. The familial occurrence of obstructive lesions in the left ventricular outflow tract and aortic arch, nonetheless, suggests that ongoing genetic advances will provide additional aetiological insights.
ANATOMY
Overall Structure
Hypoplasia of the left heart is usually found in the setting of usual atrial arrangement with concordant atrioventricular and ventriculo-arterial connections ( Fig. 29-1 ), with absence of the left atrioventricular connection in some instances ( Fig. 29-2 ). The combination should be anticipated to exist in the mirror-imaged format. There is usually obstruction to the left ventricular inflow and outflow, but this is variable. At the extreme, the left ventricular cavity may be no more than a slit in the postero-inferior wall of the ventricular mass (see Fig. 29-2 ).


While the anatomist can discover small ventricles by dissecting between the delimiting coronary arteries, it is almost impossible to determine morphologically when the left ventricle is to be considered hypoplastic in patients with aortic valvar stenosis rather than atresia. Some of these hearts show obvious fibro-elastosis in the small ventricle and certainly fulfill the anatomical criterions for the syndrome ( Fig. 29-3 ).

Under other circumstances, the ventricle may seem hypoplastic, but the morphologist will be unable to judge its ability to maintain an effective systemic circulation during life. It is the existence of such small left ventricles, in which the dimensions of the aortic and mitral valves are in keeping with the dimensions of the ventricle itself, which underscore the suggestion that some forms of the syndrome can be corrected in biventricular fashion without the need for surgery on either valve, hence the description of the so-called hypoplastic left heart complex. 4
Typically, the left ventricular walls are hypertrophied (see Fig. 29-1 ). Indeed, the mass of the left ventricle may rarely be increased rather than decreased, despite the cavitary hypoplasia. The left atrium is usually small (see Fig. 29-2 ). In typical cases, there can be hypertrophy of the left atrial walls, and some patients can be seen with an enlarged atrium, particularly the appendage. Left atrial endocardial fibro-elastosis is rare. In up to one-tenth of patients, the atrial septum will be intact ( Fig. 29-4 ). In some of these patients with an intact floor to the oval fossa, while the pulmonary venous connections are normal, the pulmonary venous drainage can be abnormal because of the presence of a so-called levo-atrial cardinal vein, or because of fenestration of the walls that usually separate the left atrium from the cavity of the coronary sinus. This provides an overflow for the left atrial return. In other circumstances, however, there is no such overflow when the atrial septum is intact. The resulting increase in left atrial pressure then produces increased left atrial hypertrophy, along with changes in the lungs, including arterialisation of the pulmonary veins ( Fig. 29-5 ) and lymphangiectasia, with a cobblestone appearance of the pulmonary surfaces seen at autopsy ( Fig. 29-6 ). This is a bad prognostic feature. In most instances, however, the oval foramen is patent and does not obstruct flow from left to right, albeit that the primary atrial septum is frequently deviated into the left atrium ( Fig. 29-7 ). 17 Anomalous pulmonary venous connections are seen in a small proportion of patients, with supracardiac drainage being most often encountered.




Obstruction of Inflow to, and Outflow from, the Left Ventricle
In almost all cases, the mitral valve is either stenosed or atretic. In the presence of mitral stenosis, all components of the valve contribute to the obstruction ( Fig. 29-8 ). When atretic, the mitral valve may be imperforate, or absent together with the left atrioventricular connection (see Figs. 29-2 and 29-7 ). Left ventricular endocardial fibro-elastosis is seen only when the mitral valve is perforate (see Figs. 29-3 and 29-8 ). Obstruction of the left ventricular outflow tract is an invariable feature. Aortic atresia, as with mitral atresia, can result from an imperforate valve, but more usually there is no evidence of persisting leaflets at the ventriculo arterial junction, fibromuscular tissue interposing between the ventricular cavity and the blind-ending aortic root (see Fig. 29-1 ). The aortic root itself is usually markedly hypoplastic, with the ascending aorta serving only as a conduit to feed the coronary arteries. The components of the arch itself are variably hypoplastic, including the ascending component. 20 When the outflow tract is patent, the aortic leaflets are thickened and dysplastic (see Fig. 29-3 ). Rarely, they may be absent, with a stenosing ring seen at the ventriculo-arterial junction, similar to that observed in so-called absent pulmonary valve syndrome. 21 Further distally, aortic coarctation is common, occurring in more than four-fifths of patients. The obstructive shelf is typically in preductal location ( Fig. 29-9 )but can be found paraductally ( Fig. 29-10 ). Ductal tissue is not only incorporated into the stenosing shelf, but also may extend proximally and distally, 22 an important point for those undertaking surgical palliation of these patients.



The Coronary Arteries and Conduction Tissues
Abnormalities of the coronary arteries, such as ventriculo-coronary fistulas and abnormal tortuosity, are more common in the subgroup of patients with mitral stenosis and aortic atresia, but not nearly as frequent as in pulmonary atresia with intact ventricular septum (see Chapter 30 ). Coronary arterial fistulas, if present, are not necessarily associated with abnormalities of the right ventricular myocardium, but can produce an increased risk of mortality at the first stage of Norwood reconstruction. 23 The conduction tissues are in their expected location, albeit with miniaturisation of the left bundle branch in keeping with the size of the left ventricle.
NATURAL HISTORY
The two most significant advances in altering the natural history in infants with hypoplasia of the left heart are the widespread availability of antenatal ultrasonography and prostaglandin E 1 . As mentioned above, in the current era the majority of neonates who survive to term have already been diagnosed antenatally by ultrasonography. It is increasingly rare for neonates to present with critical congenital cardiac disease with shock, acidosis, and hypoxemia. 24 Antenatal diagnosis allows the neonate to be delivered at or near a tertiary care centre for therapy, stabilisation with prostaglandin, and the ability to plan intervention on a semi-elective basis, with a decreased likelihood of cerebral injury, as well as hepatic, renal, or cardiac dysfunction. Prior to the availability of prostaglandin E 1 , virtually all children died in early infancy, nine-tenths within the first 30 days of life. 25
Atresia, or significant hypoplasia, of the mitral valve results in a physiological state where there is complete mixing of the systemic and pulmonary venous circulations in the right atrium. The mixed venous blood then passes across the tricuspid valve to the dominant right ventricle. Thereafter, the right ventricular output must be divided between the pulmonary and systemic arterial circuits, which are in parallel. In this situation the saturations of oxygen in the pulmonary artery and the aorta are equal, and the ventricular output is the sum of the flow of blood to the lungs and to the systemic circulation. The proportion of the ventricular output that goes to either of these vascular beds is determined by the relative resistance to flow into the two circuits. Tricuspid regurgitation may further add to the volume work of the dominant right ventricle.
THE TRANSITIONAL CIRCULATION
In the neonate with hypoplasia of the left heart, the normal fall in pulmonary vascular resistance over the first few hours to days of life, in the absence of a significant obstruction to flow into the lungs, results gradually in increased pulmonary flow. The right ventricle becomes progressively volume overloaded, with mildly elevated end-diastolic and atrial pressures. Expected physiological responses include oliguria, with an increase in retention of salt and water, an increase in ventricular stroke volume, tachycardia, and tachypnea. If the saturation of oxygen in the pulmonary veins is assumed to be from 95% to 100%, and that in the systemic veins is between 60% and 65%, with equal distributions of pulmonary and systemic flows, and hence venous returns, the saturation of oxygen in the aorta will be approximately 80%. This has been termed the so-called balanced circulation.
With a continued decrease in the pulmonary vascular resistance, there is an increase in flow to the lungs, and the neonate may show signs of respiratory distress. The greater proportion of pulmonary venous return in the mixed ventricular blood results in an elevated saturation of oxygen in the systemic circulation, to approximately 90% or higher, and visible cyanosis may be mild or absent. Prior to surgical intervention, a number of manoeuvres may be employed to balance the circulation, in attempts to maximise delivery of oxygen and systemic flow. These include sedation, neuromuscular blockade, tracheal intubation and mechanical ventilation with precise control of pH and alveolar pressures of carbon dioxide, addition of inspired gases such as nitrogen or carbon dioxide, and transfusion to increase the viscosity of the blood. Manoeuvres that lower systemic vascular resistance, such as the use of intravenous vasodilators, despite reducing pulmonary flow, may compromise systemic flow. The goal of management prior to surgery, therefore, should not be to seek to balance the circulations by equalizing pulmonary and systemic flows, but rather to provide adequate delivery of oxygen to the tissues. In usual practise, this correlates more or less with equal pulmonary and systemic flows. Many patients, nonetheless, will have adequate delivery of oxygen with minimal symptoms despite significantly increased flow to the lungs, a situation frequently dubbed pulmonary over-circulation. Such pulmonary over-circulation, nonetheless, does not cause a problem unless accompanied by systemic undercirculation. In practise, most patients tolerate the transitional circulation well for a number of days or longer, and most invasive interventions can and should be avoided. In most of these patients, an escalation in ventilation and pharmacological management only temporises the need for surgical intervention, and increases the potential for iatrogenic complications. Patients with unbalanced functionally univentricular physiology may be considered in a spectrum of two physiological extremes, namely, inadequate flow to the lungs, which results in hypoxemia, and excessive flow, which results in congestive cardiac failure.
Inadequate Flow
The newborn with hypoplasia of the left heart and an inadequate arterial saturation of oxygen, below 65%, may have limited flow of blood to the lungs due to elevated pulmonary vascular resistance. More commonly, however, the cause is obstruction to pulmonary venous outflow, most commonly due to a restrictive intra-atrial communication. Alternatively, the hypoxaemic newborn may have adequate flow to the lungs, but this will be hypoxaemia due to pulmonary disease, the latter causing intrapulmonary right-to-left shunting.
Excessive Flow
A more common situation in patients with hypoplasia of the left heart who have not undergone surgical intervention is progressively increasing flow to the lungs at the expense of systemic flow. When severe, this results in systemic hypoperfusion, metabolic acidosis, and shock. Once persistent patency of the arterial duct has been confirmed, manoeuvers should be instituted to minimise systemic and maximise pulmonary vascular resistance. Hypotensive patients with a relatively high arterial oxygen saturation, greater than 95%, generally have a severe steal by the pulmonary vascular circuit from the combined ventricular output. In these over-circulated patients, excessive inotropic support, particularly at alpha-doses, should be minimised. Reduction of afterload using agents such as sodium nitroprusside or milrinone may be especially helpful in patients with elevated systemic vascular resistance and an adequate blood pressure.
Patients with hypoplasia of the left heart and high arterial saturations, nonetheless, may suffer decreased delivery of oxygen to the tissues. The increased content of oxygen comes at the expense of a relative reduction of systemic flow, which results in inadequate perfusion, metabolic acidosis, and low cardiac output. In addition, ventricular mural tension and consumption of oxygen are increased in the dilated, volume-overloaded, dominant right ventricle, potentially contributing to myocardial dysfunction and atrioventricular valvar regurgitation. Progressive metabolic acidosis is a worrisome sign in these patients and requires prompt evaluation and, in many cases, surgical intervention.
Manoeuvres to increase pulmonary vascular resistance have been shown clinically to be effective in reducing excessive flow to the lungs. Provision of supplemental inspired nitrogen or carbon dioxide may elevate pulmonary vascular resistance by inducing alveolar hypoxia. The haematocrit should be maintained at greater than 40% to 45%, as the increased viscosity may also serve to elevate the pulmonary vascular resistance. Intubation and mechanical ventilation with sedation, paralysis, and permissive hypoventilation can be used to elevate the partial pressures of carbon dioxide into the range from 40 to 50 torr.
As already discussed, patients with marked over-circulation and systemic hypoperfusion should not undergo a lengthy period of medical management of their unstable physiology prior to surgical intervention. If a patient requires intubation and sedation to maintain adequate systemic flow, early surgical intervention is indicated to achieve more favourable physiology.
CLINICAL PRESENTATION AND PRE-OPERATIVE STABILISATION
Neonates tend to present in one of three mutually exclusive ways. If the diagnosis has been made prenatally, an expectant team of caregivers manages a metabolically stable neonate. Neonates can also present with symptoms of mild congestive heart failure, with or without a cardiac murmur and no dysfunction of the end-organs, or with profound circulatory collapse and failure of organs in multiple systems. In this last group, a sudden deterioration takes place, with rapidly progressive congestive cardiac failure and shock occurring concurrent with constriction of the arterial duct. There is decreased systemic perfusion, and greatly increased flow to the lungs, largely independent of the level of pulmonary vascular resistance. The peripheral pulses are weak to absent. Renal, hepatic, coronary, and central nervous system perfusions are compromised, possibly resulting in acute tubular necrosis, necrotizing enterocolitis, or cerebral infarction or haemorrhage. A vicious cycle may also result from inadequate retrograde perfusion of the ascending aorta and coronary arterial supply, with further myocardial dysfunction and continued compromise of flow to the coronary arteries. The ratio of pulmonary-to-systemic flows approaches infinity as systemic flow nears zero. Thus, one has the paradoxical presentation of a profound metabolic acidosis in the face of a relatively high partial pressure of oxygen, in the region of 70 to 100 mm Hg. At the initial presentation, sepsis is frequently suspected before the cardiac diagnosis is made.
Analysis of arterial blood gases is typically a commonly employed indicator of haemodynamic stability and adequacy of systemic delivery of oxygen. While a low arterial saturation of oxygen, in the region from 75% to 80%, with a normal pH and partial pressures of carbon dioxide, indicates an acceptable balance of systemic and pulmonary flows with adequate peripheral perfusion, elevated saturations of greater than 95%, when associated with acidosis, represent significantly increased pulmonary and decreased systemic flows, with probable myocardial dysfunction and secondary effects on other organ systems.
Oxygen
In general, supplemental oxygen should be avoided pre-operatively in the infant with hypoplasia of the left heart. Oxygen will act as a pulmonary vasodilator, increasing the flow of blood to the lungs at the expense of systemic flow. The presence of pulmonary pathology, such as atelectasis, meconium aspiration, or pneumonia, or a restrictive interatrial communication, may result in a requirement for supplemental oxygen. In the absence of these features, most neonates with hypoplasia of the left heart have increased pulmonary flow prior to surgery, with saturations of oxygen in room air from 88% to 95%, representing partial pressures of oxygen from 45 to 50 mm Hg or greater. Patients with a mildly restrictive interatrial communication and/or elevated pulmonary vascular resistance will have saturations of oxygen in the high 70s to low 80s. Although the temptation is to increase the inspired oxygen, if the low saturation represents the best balance between pulmonary and systemic flows, increasing the supplemental oxygen may paradoxically decrease delivery of oxygen to the tissues.
When to Treat a High Saturation of Oxygen
The clinically stable infant may tolerate significantly increased pulmonary flow in the pre-operative period without significant intervention or obvious untoward effects. When the diagnosis is made early, before significant constriction of the arterial duct and development of a state of low flow, acidosis is usually avoided. Infants in this setting often have a mild degree of restriction across the interatrial communication, with left atrial hypertension elevating pulmonary vascular resistance, and limiting to some extent the flow to the lungs. Although tachypnoeic, with respiratory rates from 60 to 80 per minute, and with an arterial oxygen saturation of 90% or higher, they remain clinically stable with minimal intervention. This group of patients maintains adequate systemic flow despite increased pulmonary flow. Further interventions designed to achieve an arbitrary partial pressure of oxygen, such as intubation and/or supplemental provision of inspired gases, may not stabilise the patient, but rather increase the potential for iatrogenic complications. In short, the neonate without significant symptoms of congestive heart failure, acidosis, or progressive respiratory distress should not necessarily require significant therapy to treat high partial pressures of oxygen. Only two subsets of infants require aggressive pre-operative management of the balance between their pulmonary and systemic blood flow. The first group is made up of those who are diagnosed late, following constriction of the arterial duct and circulatory collapse. The second group is composed of those with clinically significant pulmonary over-circulation and systemic undercirculation, independent of the timing of diagnosis.
Presentation in Shock
Infants presenting with inadequate systemic cardiac output, either due to ductal constriction or pulmonary over-circulation, often have depressed cardiac function with significant tricuspid insufficiency, profound metabolic acidosis, usually some hepatic and renal failure, and occasionally bowel ischaemia or disseminated intravascular coagulopathy. These infants require rapid and aggressive resuscitation. Prostaglandin E 1 must be given, the dose being adjusted according to the degree of patency of the arterial duct as revealed echocardiographically. Intravenous infusions of catecholamines, such as dopamine or adrenaline, will help augment overall cardiac output. Sedation, paralysis, and endotracheal intubation will allow for controlled ventilation and minimise systemic consumption of oxygen. If a patient continues to have inadequate delivery of systemic oxygen despite controlled ventilation with 21% oxygen, the addition of inspired carbon dioxide has been shown to improve systemic delivery of oxygen (see below). Most commonly, infants with hypoplasia of the left heart presenting in shock benefit from recovery of end-organ function before proceeding to the operating room for surgical reconstruction or transplantation. Importantly, infants with persistent metabolic acidosis despite resuscitative efforts should be evaluated by echocardiography to establish adequate patency of the arterial duct. Rarely, a pre-operative infant with hypoplasia of the left heart persists with medically refractory pulmonary over-circulation and may require urgent construction of the initial stage of the Norwood sequence.
Hypotension
Although mild pre-operative hypotension is common, and frequently thought to be caused by increased pulmonary flow at the expense of systemic flow, alternative aetiologies also play a role. Prostaglandins are potent pulmonary and systemic vasodilators, and adequate fluids are needed following their administration. Neonatal myocardium is particularly sensitive to intracellular calcium and glucose, so the levels of both agents in the serum should be monitored periodically. Myocardial dysfunction may co-exist, and although small-to-moderate doses of inotropic agents are frequently beneficial, large doses may have a deleterious effect, depending on the relative effects on the systemic and pulmonary vascular circulations. Preferential selective elevations of systemic vascular tone will secondarily increase pulmonary flow, and careful monitoring of mean arterial blood pressure and arterial saturations of oxygen is warranted. Tricuspid regurgitation from an anatomically abnormal valve, or secondary to ventricular dysfunction, may also contribute. Low doses of inhibitors of phosphodiesterase, such as milrinone, which may seem counter-intuitive for the mildly hypotensive patient, may improve overall perfusion and delivery of oxygen. Patients with hypotension that remains refractory despite all of the above interventions should undergo echocardiographic evaluation to ensure that the arterial duct remains widely patent and non-restrictive. Inadvertent or unrecognised problems in the administration of prostaglandin E 1 can constrict the arterial duct.
Inspired Gases
The use of mixtures of inspired gases to balance the pulmonary and systemic flows prior to surgical intervention remains controversial. 26–28 Until recently, prospective, controlled data comparing hypoxia to hypercarbia was limited to studies in shunt-dependent animal models of the functionally univentricular circulation. 29 Use of both induced alveolar hypoxia and increased inspired carbon dioxide produced a significant decrease in the ratio of pulmonary to systemic flows compared to baseline. In a prospective crossover study measuring saturations of oxygen in the superior caval vein and aorta while estimating saturations of oxygen in the brain using near infrared spectroscopy, however, it was shown that hypoxia induced by nitrogen and hypercarbia by carbon dioxide both decreased the ratio of pulmonary to systemic flows. Importantly, only hypercarbia increased cardiac output and cerebral oxygenation. 27
In our practise at The Children’s Hospital of Philadelphia, therefore, we tend not to use inspired gases pre-operatively. Surgery is undertaken as soon as is medically and logistically possible. For the neonate presenting with circulatory collapse and pulmonary over-circulation, and already intubated and mechanically ventilated, nonetheless, we give inspired carbon dioxide until surgery is undertaken. For patients in whom surgery must be delayed, and there is clinically significant pulmonary over-circulation with low systemic flow, nitrogen is inspired to the spontaneously breathing patient, and carbon dioxide reserved for the mechanically ventilated patient until surgery is undertaken.
Restrictive Atrial Septum
A severely restrictive atrial septum limits outflow from the pulmonary veins. If coupled with mitral atresia, this results in profound obstruction to left atrial egress, producing pulmonary venous hypertension and hypoxaemia following birth. Newborns with this combination are extremely unstable, and difficult to manage immediately following birth. Recent strategies to improve the dismal prognosis have been met with limited, albeit encouraging, success, including prenatal intervention with static atrial septal dilation with or without placement of a stent, and/or planned delivery by cesarean section at a centre equipped to provide surgical or catheter intervention within a very short time frame. Following birth, if inadequate flow to the lungs and systemic oxygenation are confirmed, the infant should be intubated, umbilical access obtained, and prostaglandin E 1 given in the delivery room, followed by direct transportation to the operating room or catheterisation lab where the planned septectomy or catheter intervention may be performed. In the absence of a prenatal diagnosis, the clinical presentation is identical to obstructed totally anomalous pulmonary venous return, or severe pulmonary disease. From the stance of management, intubation, positive end expiratory pressure, and supplemental oxygen are needed to maintain a saturation of oxygen in the aorta of around 80%, coupled with umbilical access, inotropic support, and other supportive strategies.
Unfortunately, the association of a severely restrictive or intact atrial septum with hypoplasia of the left heart has a significantly worse prognosis over the short and long term compared with other forms of left heart hypoplasia 30,31 mainly due to the secondary changes that occur in the pulmonary vascular bed from fetal obstruction to pulmonary venous outflow (see Fig. 29-5 ). The pulmonary vascular tree is abnormal in all patients with hypoplasia of the left heart, with an increase in the number of arteries per unit area of lung, the arteries themselves having a considerable increase in muscularity. Increased medial arterial thickness is present, as well as extension of muscle into smaller and more peripheral arteries than is normal. This may explain the predilection towards extreme sensitivity of the pulmonary vascular bed to vasoactive agents. The pulmonary veins are typically dilated with thickened walls. The degree of restriction at the atrial level influences the pathological findings within the pulmonary vascular tree. Increased arterial tortuosity and arteriopathy have been noted, along with arterialisation of the pulmonary veins. 30 These pulmonary venous abnormalities further contribute to the pathophysiology once these infants are born, and may explain why most continue to do poorly with high mortality despite creation of a large and effective interatrial communication at birth.
PRE-OPERATIVE EVALUATION
Newborns with hypoplasia of the left heart require a thorough evaluation of cardiac and non-cardiac issues upon admission to the intensive care unit. Vascular access should be secured, prostaglandin administered, and evaluation of acid-base status undertaken. The physical examination should assess adequacy of systemic perfusion, and carefully evaluate for additional congenital anomalies and dysmorphic features. A complete echocardiogram is usually sufficient to plan surgical intervention. In particular, care should be taken to establish the presence or absence of bilateral superior caval veins; the number of, and patterns of flow in, the pulmonary veins; the state of the atrial septum and ventricular myocardium; the anatomy and function of the tricuspid and pulmonary valves; the size of the ascending aorta; and the presence of any abnormal patterns of branching of the brachiocephalic arteries. Representative examples of important aspects of the anatomy are shown in Figures 29-11 to 29-13 . The patency and characteristics of flow through the arterial duct are crucial to maintaining the stability of the patient and understanding the physiology, and may be utilised to estimate the ratio of pulmonary to systemic flows 32 ( Fig. 29-14 ). Coronary arterial sinusoids may be identified in some patients in the setting of aortic atresia and mitral stenosis. These are particularly important to identify as they have been recently shown to be risk factors for mortality following surgical intervention. 33,34 Should there be evidence of retardation of growth or additional congenital anomalies, the remaining systems such as the brain, kidneys, liver, and gastrointestinal tract should be evaluated. Consultation with a clinical geneticist may be helpful.




THERAPEUTIC OPTIONS AND POST-OPERATIVE CARE
There are currently four options available for the neonate with hypoplasia of the left heart. The first is to abstain from intervention. The second alternative is that of staged reconstruction, beginning with the first stage of the Norwood protocol and culminating in construction of the Fontan circulation. The third option is to opt for primary cardiac transplation. The fourth involves a hybrid approach, stenting the arterial duct, banding the pulmonary arteries, and opening the interatrial communication if necessary.
The option of declining to intervene is now controversial. 26,35,36 Most centres currently active in cardiac surgery for neonates and infants do not actively offer non-intervention, 26 although non-intervention might be adopted by many providers of health care if personally faced with the situation. 35 It is beyond the scope of this chapter to review the ethical issues, tenets of informed consent, and changing landscape of this controversial topic.
EVOLUTION OF SURGICAL THERAPY
Surgical palliation for a patient with hypoplasia of the left heart was first attempted in 1961, 37 and the first survivors were reported in the early 1970s. 38,39 Alternative innovative approaches were then reported in the later part of the 1970s, indicating that staged reconstruction would be needed successfully to achieve the Fontan circulation. 40 Such a staged approach was formulated by Norwood during the early 1980s. 41 Even in experienced centres, however, initial survival remained poor. Shortly thereafter, primary cardiac transplantation was shown to be achievable in the neonate. 42 While the initial surgical mortality was superior to staged reconstruction, and the possibility of a biventricular circulation was appealing, the approach was limited by the availability of suitable donors, with many patients dying in the waiting period prior to transplantation. The introduction of ABO-incompatible cardiac transplantation increased the availability of donors, 43 albeit that such an approach has not universally been adopted as primary therapy at many centres. 26 The most recent major innovations have involved combined transcatheter and surgical approaches. 44,45 Such palliation with a ductal stenting and banding of the pulmonary arteries was initially conceived as a bridge to cardiac transplantation, but more recently has been used prior to a simultaneous reconstruction of the aortic arch and construction of a superior cavopulmonary connection, dubbed the comprehensive second stage. The ideal surgical strategy, therefore, remains controversial. Most likely it will eventually be tailored to fit the risk factors of the specific patient. Results from all currently available strategies, nonetheless, have vastly improved the outlook for infants born with hypoplasia of the left heart.
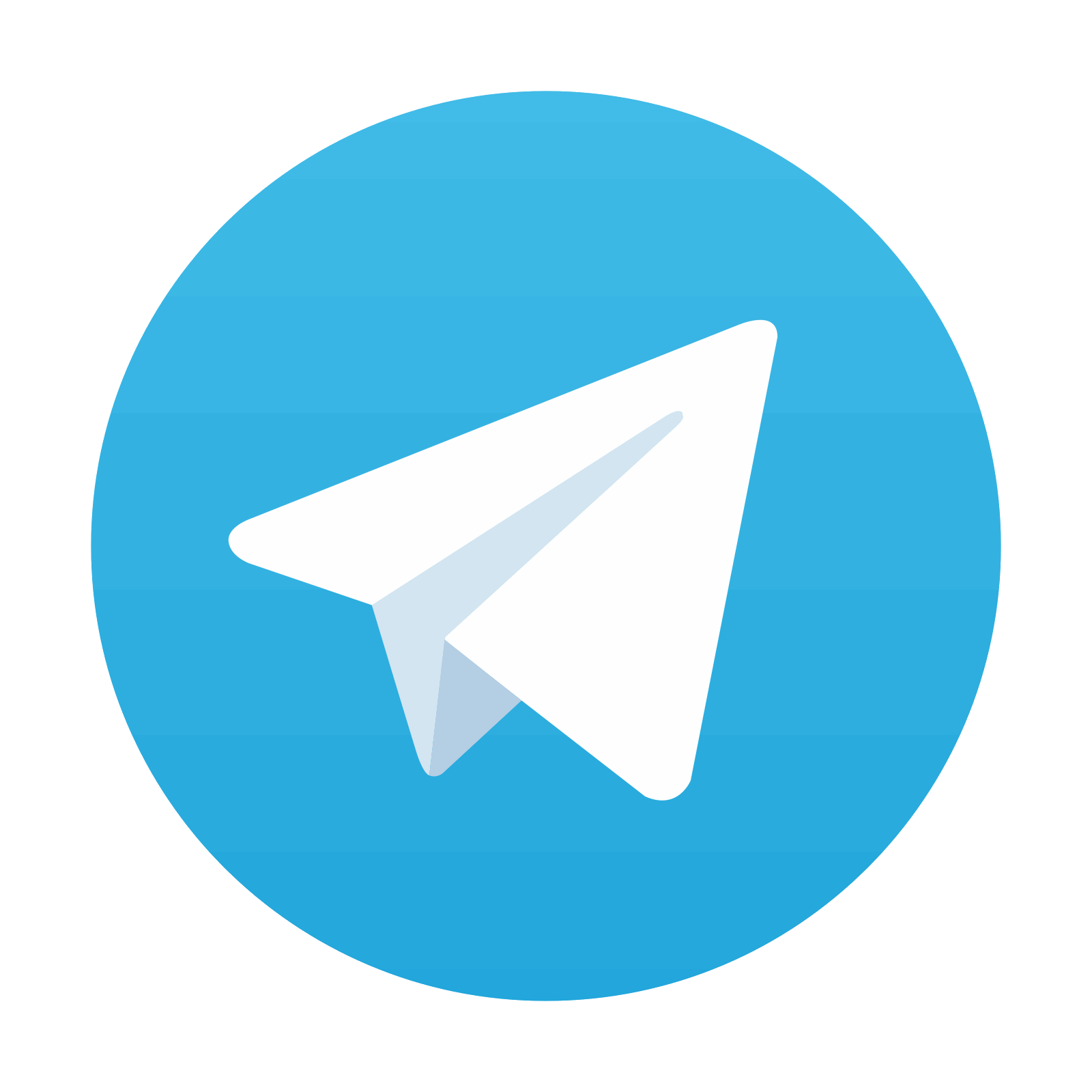
Stay updated, free articles. Join our Telegram channel

Full access? Get Clinical Tree
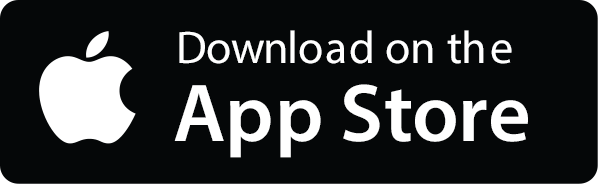
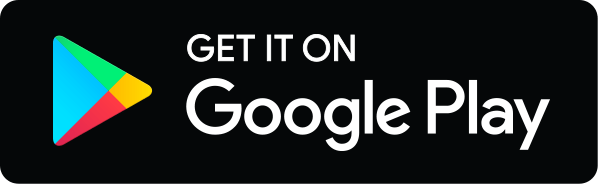