Fig. 29.1
3-D reconstructions of human embryos. Approximate gestational age 8 weeks (crown-rump length 18 m, Carnegie stage 14). Frontal views. The heart and part of the left atrial dorsal walls have been resected. (a) Normal embryo. Arrows indicate 4 pulmonary veins that drain the lung towards the left atrium (LA). There are no pulmonary-to-systemic connections anymore via the splanchnic venous plexus (SVP), although small communications of this plexus into the right and left cardinal veins (RCV and LCV) can still be observed. (b) Embryo with abnormal pulmonary venous connection. The black arrows point at 2 right-sided pulmonary veins normally draining towards the LA. The left superior pulmonary vein is lacking (open arrow), and the left inferior pulmonary vein is devoid of blood (black arrowhead). Note the extensive SVP, with numerous connections to both lungs. Also, a large connection (C) is present between the SVP and the LCV. Abbreviations: AO aorta, DA ductus arteriosus, DAO descending aorta, E esophagus, INV innominate vein, T trachea (Source: Modified after Rammos et al. [30])
Clinically it is important to know that the myocardial sleeve surrounding the pulmonary vein is missing in hearts with TAPVC, while the left atrial dorsal wall is hypoplastic [2]. The accompanying sinus node dysfunction in some of these patients [3, 4] points to an abnormal contribution of the so-called posterior heart field (part of the second heart field, see Chap. 30), which is also evident in the patient group with total absence of the myocardial sleeve presenting with a low incidence of atrial arrhythmias [4]. We have to keep in mind that many other patterns of pulmonary venous return are encountered such as partial anomalous pulmonary venous return including scimitar vein [5], cor triatriatum, primary and acquired stenosis, sinus venosus defects, atrial septal defect II (ASDII), and variant numbers of pulmonary veins which may complicate an adequate diagnosis [6].
29.2 TAPVC and Genetics
Partial anomalous pulmonary venous connections have been found after abnormal gene expressions involving several syndromes, including mutations in GATA4 (GATA binding protein 4) [7] and MEK1/MEK2 (MAPK/ERK kinase 1/2; also known as MAP2K1/2, mitogen-activated protein kinase kinase 1/2) [8]. TAPVC is found in conjunction with heterotaxia syndrome involving the nodal pathway [9] and Zic3 (Zic family member 3) mutations [10], Holt-Oram syndrome, cat eye syndrome [11–13], craniofacial and skeletal dysmorphia [13], cardio-facio-cutaneous syndrome [8], and Williams syndrome [14]. It has been reported with an autosomal dominance with variable expression and incomplete penetrance [15]. Genetic screens reveal rare copy number variation [16], submicroscopic genomic aberrations [17], and mutations in histone-modifying genes [18] related to TAPVC. The occurrence is also related to environmental factors such as exposure to lead, solvents, and pesticides, which may superimpose on a familial susceptibility for TAPVC [19]. It may be concluded that many pathways are involved in the normal development of the pulmonary venous connections and as a consequence that disturbance of many genetic and epigenetic pathways lead to partial or total pulmonary venous misconnections. Analysis of families with high frequency of TAPVC revealed three candidate genes: TAPVR1, ANKRD1/CARP (ankyrin repeat domain 1, cardiac muscle), and SEMA3D (semaphorin 3d).
TAPVR1 has been mapped to chromosome 4q12, the centromeric region containing the kinase domain receptor (KDR) gene, that plays a role in vasculogenesis [20]. Disturbed vaculogenesis may potentially lead to deficient development of the mid-pharyngeal endothelial strand, causing PAPVC with persisting pulmonary-to-systemic connections, although the exact developmental mechanism has not been elucidated. More recently, the TAPVR1 region has been narrowed down to an intergenic region between PDGFRA and KIT [21]. Mutation analysis of the PDGFRA (platelet-derived growth factor receptor, alpha polypeptide) gene in TAPVC patients revealed a rare sequence variant pointing towards a presumptive TAPVC gene. Mouse models with deficient PDGFr alpha show a small DMP (see Chap. 30) and abnormal drainage of the systemic and pulmonary veins, although a phenotype of TAPVC was not observed [22].
Furthermore, the ANKRD1/CARP gene encoding a muscle specific protein has been implicated in cardiac transcriptional regulation and myofibrillar assay [23]. It was localized to chromosome 10, proximal to a previously found translocation point [24, 25]. The ANKRD1/CARP gene is regulated by GATA4, and disruption of this signalling axis in vitro leads to sarcomere disarray [26]. Ankyrin repeat proteins are stress inducible that are thought to be involved in mechanosensing in response to pressure overload [27]. Therefore, the mechanism of the occurrence of TAPVC may relate to atresia caused by dysregulated flow through the embryonic mid-pharyngeal endothelial strand (MPES), although this remains speculative at this point.
As described above, pulmonary vein development starts with the early embryonic connection of the MPES to the heart [28]. The development of the early pulmonary veins depends heavily on the MPES and the associated semaphoring signalling (Chap. 30). Sequencing of SEMA3D in patients with TAPVC and PAPVC revealed two variants. One variant, a c.193 T >C missense, resulted in a serine-to-proline substitution, whereas a second variant, a c.1806 T >A missense mutation (phenylalanine-to-leucine), was present in an individual with PAPVC with an associated ventricular septal defect but no signs of heterotaxia. The amino acid alteration is located in the immunoglobulin-like domain of SEMA3d probably important for both endothelial neuropilin receptor binding and functional activity [29].
29.3 Conclusion
In conclusion, several mutations have been described in the human population that affect the formation of the pulmonary venous drainage. The perturbed genes are involved in PDGF signalling, sarcomere formation, and endothelial migration, thereby regulating various phases of differentiation. In view of the complexity of the interactions, it will be no surprise to find in the near future that more signalling pathways are involved.
References
1.
2.
Douglas YL, Jongbloed MR, den Hartog WC et al (2009) Pulmonary vein and atrial wall pathology in human total anomalous pulmonary venous connection. Int J Cardiol 134:302–312PubMedCrossRef
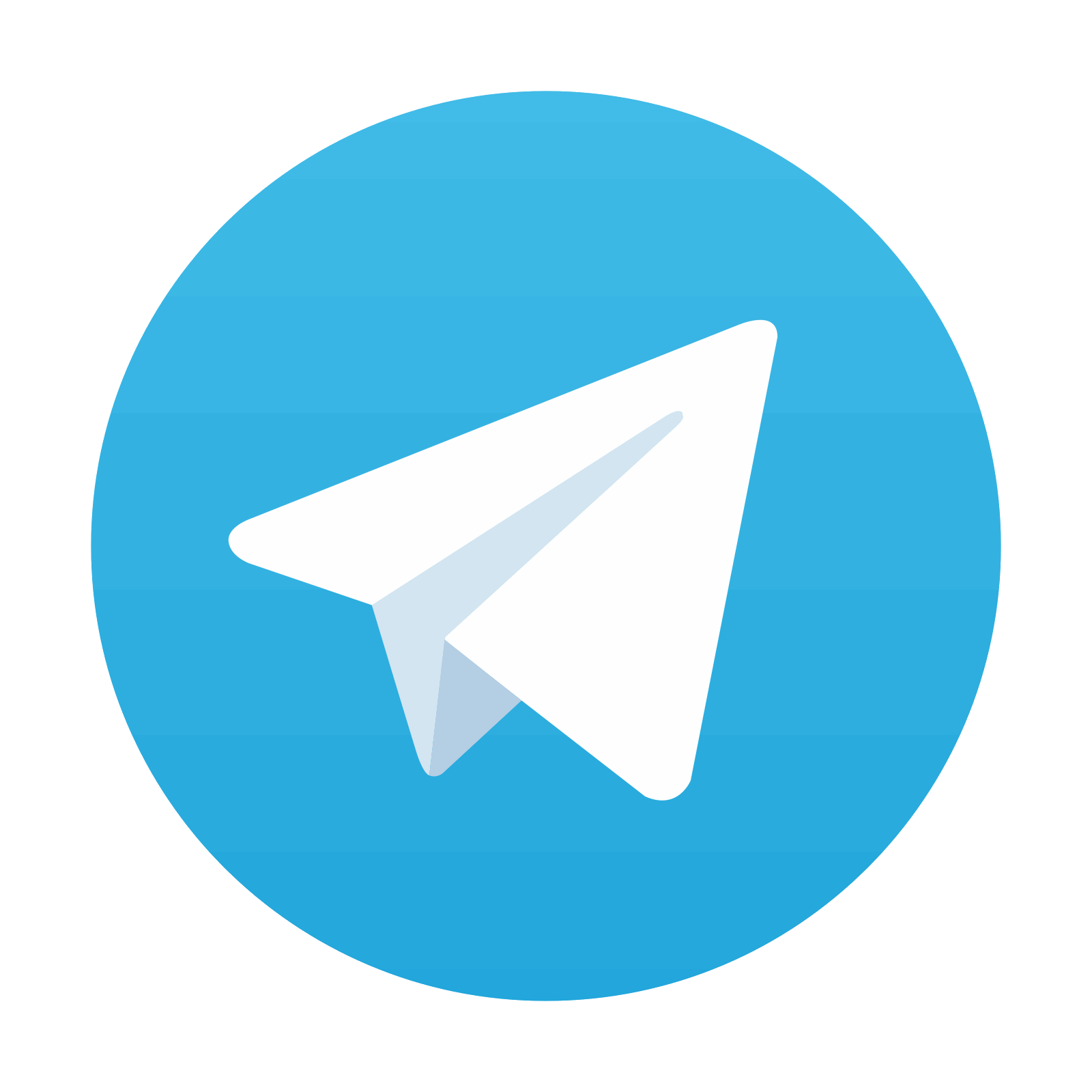
Stay updated, free articles. Join our Telegram channel

Full access? Get Clinical Tree
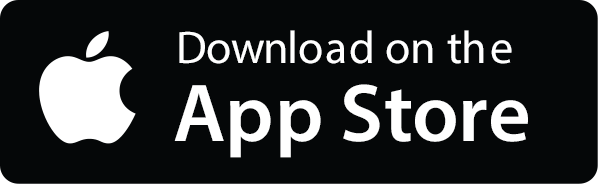
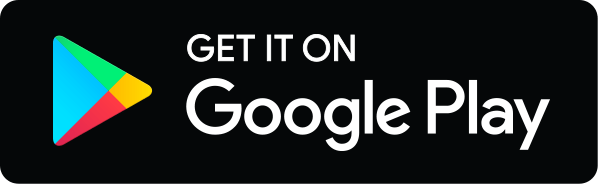